ABSTRACT
Species of the subgenus Brachypetalum are the most primitive, most ornamental and most threatened group in the Orchid. This study revealed the ecological characteristics, soil nutrient characteristics and soil fungal community structure of habitats of the subgenus Brachypetalum in Southwest China. Lays a foundation for research on the wild populations and conservation Brachypetalum. The results showed that species of the subgenus Brachypetalum preferred a cool and humid environment, grew in scattered or aggregated form in narrow negative terrain, mainly in humic soil. The soil physical and chemical properties and soil enzyme activity indexes of the habitats were significantly different among different species, and the soil properties of different distribution points of the same species also varied greatly. There were significant differences in the soil fungal community structure among the habitats of different species. Basidiomycetes and ascomycetes were the main fungi in habitats of subgenus Brachypetalum species, and their relative abundance varied among different species. The functional groups of soil fungi were mainly symbiotic fungi and saprophytic fungi. LEfSe analysis found that there were different numbers and species of biomarkers in the habitats of subgenus Brachypetalum species, indicating that the habitat preference characteristics of each species in subgenus Brachypetalum were reflected in the fungal community. It was found that environmental factors had an impact on the changes in soil fungal communities in the habitats of subgenus Brachypetalum species, with climatic factors having the highest explanation rate (20.96%). Soil properties were significantly positively or negatively correlated with a variety of dominant soil fungal groups. Conclusions: The results of this study lay the foundation for the study of the habitat characteristics of wild populations of subgenus Brachypetalum and provides data to support in situ and ex situ conservation in the future.
Orchidaceae is one of the largest families of higher plants. There are approximately 800 genera and 25,000–35000 orchid species in the world,Citation1 accounting for approximately 10% of the total number of seed plants. Orchids have highly specialized organs, diverse forms, special pollination mechanisms, and strong ability to adapt to the environment.Citation2,Citation3They are widely distributed in various terrestrial ecosystems except for polar and extremely arid desert areasCitation4,Citation5, have important scientific research value and unique ornamental and medicinal value, which has attracted the attention of many researchers and flower lovers around the worldCitation6,Citation7.
The genus Paphiopedilum is a primitive group of Orchidaceae.Citation8,Citation9 There are more than 80 known species of Paphiopedilum, which are distributed in tropical and subtropical regions of Asia. With the breakthrough of molecular technology in phylogeny, the genus Paphiopedilum was divided into three subgenera, Brachypetalum, Paphiopedilum, and Parvisepalum, according the current mainstream view.Citation10,Citation11 There are nine known species of subgenus Brachypetalum. They are mainly distributed in Southwest China. Species of the subgenus Brachypetalum are the most primitive, most ornamental and most threatened group in the genus Paphiopedilum. Research on Brachypetalum has been carried out for a long time, and significant achievements have been made regarding leaf photosynthetic regulationCitation12 and in vitro culture,Citation13 especially in the study of mycorrhizal fungi.Citation14 However, most of the recent research has been on species cultivation, and research on wild Brachypetalum populations is insufficient, lacking systematic and in-depth studies. On the one hand, due to its special pollination mechanism and fungus-induced germination, the subgenus Brachypetalum is often clustered and narrow in distribution, making it difficult to locate. On the other hand, the growth environment of species is remote and dangerous, which increases the cost of research and threatens the safety of investigators, making it difficult to study wild populations.
Southwest China is considered to be the concentrated distribution area and origin and differentiation center of subgenus Brachypetalum. According to Pengcheng Ye et al,Citation15 only 194 natural distribution points of 7 known species of Brachypetalum in Southwest China have been found. Most of them are distributed in karst limestone mountains in Yunnan, Guangxi and Guizhou. The soil layer in this area is thin and discontinuous, with exposed rocks and fragmented habitats, which are severely affected by human activities, and most natural distribution points of Brachypetalum species are not included in a protected area.Citation16 Hence, Brachypetalum species in this region are facing the double dilemma of extinction risk and insufficient protection and research. At present, the ecological characteristics of the habitats of the subgenus Brachypetalum are still unclear, and the characteristics of soil fungal communities in these habitats are also poorly studied. One of the most important factors restricting the conservation of the subgenus Brachypetalum in China is that we know little about its habitat ecological characteristics. The more we know about the ecological background of species, the more likely we are to suggest practical conservation measures for them.
This study reveals the habitat biological characteristics of Brachypetalum species in Southwest China, and lays a foundation for research on the wild populations and conservation Brachypetalum. This study adopts field investigation, environmental data acquisition, determination of soil nutrients, and soil microbial high-throughput sequencing methods to explore the following questions: (1) What are the environmental characteristics of the habitat of the subgenus Brachypetalum? (2) Do the habitat characteristics of Brachypetalum differ among different species? (3) Does the soil fungal community structure influence the habitats of Brachypetalum?
Materials and methods
Study area and sampling site
Southwest China is affected by the plateau monsoon climate and the subtropical humid monsoon climate. The terrain is dominated by mountainous plateaus. Southwest China also has the world’s largest and most concentrated distribution of contiguous typical karst formations. Because the region is close to the equator, the water and heat energy coupled with the complex topography create a rich biodiversity in Southwest China(). Yunnan, Guangxi and Guizhou are the main distribution areas of subgenus Brachypetalum and the three provinces with the highest biodiversity in China.
Survey and sample collection
Because the number of natural distribution sites for each species of the subgenus Brachypetalum was not the same, and some natural distribution sites were seriously disturbed by human beings, the number of sampling sites for each species could not be equal. Based on the number of distributions of subgenus Brachypetalum in Southwest China (), we selected natural distribution sites that were unperturbed and grew well, and ensure that each species has at least three sampling sites and that the habitat characteristics are as representative of the population as possible, a total of 35 natural distribution sites of 7 species of subgenus Brachypetalum were surveyed for sample collection. First, the growth status, number of individuals of Brachypetalum species were recorded. Then, habitat variables were recorded, including longitude and latitude, altitude, slope, aspect, vegetation type, habitat fragmentation degree, litter thickness, and dominant species. After removing dead branches and litter, 0.5 g soil from the habitat was collected according to the five-point sampling method, mixed to form a composite fungal sample (avoiding rhizosphere soil) and frozen in liquid nitrogen for high-throughput sequencing. An additional 200 g of soil from the habitat was also collected according to the above sampling method and thoroughly mixed, then placed in sampling bags and taken to the laboratory for testing soil physical and chemical properties and enzyme activities.
Table 1. Number of natural distribution sites and number of sampling sites for each species of the subgenus Brachypetalum.
Environmental data acquisition
We obtained 19 climatic factors from WorldClim (https://www.worldclim.org/) and obtained the biodiversity index, vegetation normalization index and primary productivity index from the Resource and Environmental Science and Data Center of the Chinese Academy of Sciences (RESDC) (https://www.resdc.cn/) and the National Qinghai-Tibet Plateau Data Center (TPDC) (http://data.tpdc.ac.cn/). The geographical coordinate system of all environmental variables was GCS _ WGS _ 1984. ArcGIS software was used to extract the environmental data of each Paphiopedilum species distribution point for subsequent analysis.
Analysis of soil physicochemical and enzyme activity
The soil physicochemical properties were analyzed and are described briefly as follows.Citation17 Soil pH (water:soil = 2.5:1) was determined using a pH meter (PHS-3 G, Hanon Instruments, China). The water content was determined by the 105°C drying-weighing method. Total organic carbon (TOC) was determined by the KCr2O7-H2SO4 external heating method. Total nitrogen (TN) was determined by the semimicro Kjeldahl method. Total phosphorus (TP) was determined by the HClO4-H2SO4 digestion-molybdenum antimony colorimetric method. Total potassium (TK) was determined by NaOH melting-flame spectrophotometry. Available phosphorus (AP) was determined by extraction with 0.03 mol/L NH4F and 0.025 mol/L HCl and analyzed by the molybdenum antimony anti-colorimetric method. Available potassium (AK) was extracted with 1 mol/L neutral NH4OAc followed by flame photometer analysis. Ammonium nitrogen and nitrate nitrogen (AN) were determined by extraction with 2 mol/L KCl followed by analysis in a continuous flow analyzer. The soil enzyme activities were determined using an enzyme analysis kit (Yangling Xinhua Ecological Technology Co., LTD., Shanxi, China).
Molecular analysis of soil fungal communities
DNA was extracted from the samples by the CTAB method, and the extracted DNA was detected by 1% agarose gel electrophoresis. PCR extraction was performed using barcode primers and high-fidelity enzymes. The fungal primer sequencesCitation18,Citation19 used were (5’-AAGCTCGTAGTTGAATTTCG-3’ and 5’-CCCAACTATCCCTATTAATCAT-3’). The PCR products were mixed and detected by 2% agarose gel electrophoresis. High-quality PCR products were used for Illumina HiSeq sequencing. First, Trimmomatic (version 0.33)Citation20 was used to filter the quality of the original data, and Cutadapt (version 1.9.1)Citation21 was used to identify and remove the primer sequence Quercus glaucaces. Subsequently, USEARCH (version 10) was used to splice the paired-end reads and remove the chimaeras (UCHIME, version 8.1)Citation22,Citation23 Chimeric sequences were removed to finally obtain high-quality sequences, and the characteristic sequences were taxonomically annotated using the Simple Bayes classifier using UNITE as the reference database.
Statistical analysis
One-way ANOVA was used to test for differences in soil physicochemical properties among habitats of Brachypetalum species, and the significance level was set to P < 0.05. The alpha diversity of the soil fungal communities in the habitats was represented by the Chao1 index, Ace index, Shannon‒Wiener index and Simpson index, and the differences in alpha diversity among species were analyzed by least significant difference (LSD) multiple comparison tests. Analysis of similarities (ANOSIM) was used to test whether the difference between species (two or more groups) was significantly greater than the difference within the group to determine whether the grouping was meaningful. Principal coordinates analysis (PCoA) was used to examine the difference in soil fungal community structure among habitats of each Brachypetalum species, and the explanation by different grouping factors of the difference in samples was analyzed. The FUNGuild database was used to determine the fungal functional groups. Canonical correlation analysis (CCA) was used to reveal the effects of environmental factors, climatic factors and soil factors on the changes in fungal community structure. A Spearman correlation heatmap was used to analyze the correlation between soil factors and dominant fungal groups. The soil fungal analysis was completed with the help of the Biomarker Cloud Platform (https://international.biocloud.net). LEfSe (Line Discriminant Analysis (LDA) Effect Size) can realize the comparison between two or more groups, as well as the comparison between subgroups within groups, so as to find the biomarker with statistical differences between different groups. LEfSe analysis was used to find biomarker with statistically significant differences in the habitat soil of each species of the subgenus BrachypetalumCitation24.
Results
Ecological characteristics of habitats of species in subgenus Brachypetalum
The study found that P. malipoense is distributed below 1000 m above sea level and grows in evergreen broad-leaved and deciduous mixed forests with normal or karst landforms, mainly occur in limestone brown soil and humus soil (). P. micranthum is the most widely distributed species in the subgenus Brachypetalum, occurring over a large elevational range (566–1628 m). It is mainly distributed in evergreen broad-leaved deciduous mixed forest and secondary forests in the karst mountainous area, distribution area ranging from 25 m2 to 2000 m2 (). P. wenshanense and P. armeniacum are distributed on the 1500-m high mountains of Yunnan Province. The main types of habitat vegetation are secondary shrub forests. P. emersonii only grows on vertical stone walls in karst areas (), and there is almost no habitat soil, and it is the species with the most hostile habitat among the subgenus Brachypetalum. P. concolor and P. bellatulum live in rock crevices in karst areas and depend on the soil in the crevices. In the survey, it was found that the microhabitat conditions of species in subgenus Brachypetalum have similar characteristics. For example, the slope of the habitat is usually determined by the terrain, the slope range is wide, from gentle to steep, and the slope direction is mainly toward the shade, such as toward the west and northwest (). These species often grow at the roots of large trees or in negative terrain, such as rock crevices and stone troughs, which is conducive to soil deposition and water retention, and the soil layer is shallow. The soil of the habitats is composed of humus soil or limestone and dolomite sedimentary soil. The soil layer is shallow and discontinuous, which also causes the species to occur in aggregations or dispersed, showing their adaption to topographic changes.
Figure 2. Habitats of species in subgenus Brachypetalum. (a) Paphiopedilum malipoense; (b) P. emersonii; (c) P. micranthum; (d) P. concolor; (e) P. bellatulum; (f) P. wenshanense, (g) P. armeniacum.
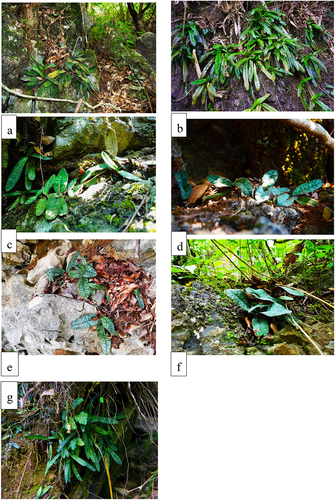
Table 2. Ecological characteristics of habitats of species in subgenus Brachypetalum.
Soil chemical properties and enzyme activities of habitats of species in subgenus Brachypetalum
It was found that the total phosphorus content in the habitat of P. bellatulum and P. concolor was significantly higher than that of P. wenshanense, and the soil organic carbon content in the habitat of P. micranthum was significantly higher than that of P. wenshanense, with an average value of approximately 4 times that of P. wenshanense (). The soil available phosphorus content in the habitat of P. wenshanense was also significantly lower than that of all species in subgenus Brachypetalum except P. armeniacum. The soil pH values of the P. emersonii and P. bellatulum habitats were significantly higher than that of the P. armeniacum habitat. The pH value of the habitats of all species in subgenus Brachypetalum was weakly acidic or alkaline. There was no significant difference in the activities of glucosidase, polyphenol oxidase or peroxidase in the soil of the habitats. However, the soil sucrase activities of P. malipoense and P. concolor habitats were significantly higher than those of the P. emersonii habitat, and the soil cellulase activity of habitats of different species also showed the same pattern (). Finally, the soil enzyme activities in the P. concolor habitat were significantly higher than those in the P. wenshanense habitat.
Table 3. Soil physicochemical properties and enzyme activities in habitats of species in subgenus Brachypetalum.
Soil fungal OTUs in the habitat of subgenus Brachypetalum
Based on Illumina NovaSeq sequencing, a total of 2,798,190 pairs of reads were obtained from habitat soil fungal samples from 35 sampling points, and a total of 2,787,667 clean reads were generated after quality control and splicing of paired-end reads. At least 79,226 clean reads were generated per sample, with an average of 79,648 clean reads (). The dilution curve can accurately reflect the sequencing depth of samples. As shown in , at 97% similarity, the dilution curves of soil fungi in the habitats of all species in subgenus Brachypetalum tended to be flat, indicating that the sample size was representative of the soil fungal community in the Brachypetalum habitats as a whole. A total of 435 fungal species belonging to 337 genera, 175 families, 38 classes, 86 orders, and 13 phyla were detected in the samples. A total of 1,872 fungal operational taxonomic units (OTUs) were obtained based on 97% similarity as the cutoff, among which the most soil-specific OTUs were found in the habitat of P. bellatulum (332), followed by P. micranthum (289), P. malipoense (210), P. emersonii (155), P. concolor (154), and P. armeniacum (144). There were only 138 unique OTUs in the habitat of P. wenshanense. Nine OTUs were common to the habitats of all species.
Comparison of fungal diversity among habitats of species in subgenus Brachypetalum
The alpha diversity of soil fungi in the habitats of species in subgenus Brachypetalum is shown in . There was no significant difference in the ACE index, Chao1 index or Simpson index of soil fungi among the habitats of the species in subgenus Brachypetalum. The Shannon index of soil fungi in the habitats of P. bellatulum and P. malipoense was significantly higher than that in the habitat of P. micranthum. Principal coordinate analysis (PCoA) based on the Bray‒Curtis distance revealed the relative differences in the fungal community of the soil sampling points in the habitats of the species in subgenus Brachypetalum (). The first principal axis explained 4.67%, the second principal axis explained 4.17%, and the third principal axis explained 3.73%, together explaining 12.57% of the differences. The Adonis test also revealed that the grouping of the species in subgenus Brachypetalum explained 21.9% of the sample differences. The nonparametric ANOSIM also confirmed that the difference in soil fungal community structure among the habitats of species in subgenus Brachypetalum was significantly greater than that within the habitats (R = 0.278, p = 0.001) ().
Composition and functional groups of soil fungal communities in habitats of species in subgenus Brachypetalum
Basidiomycota and Ascomycota were the dominant phyla of the soil fungal community in the habitats of the species in subgenus Brachypetalum (), but the relative abundance of Basidiomycota was different among the habitats of different species, and the relative abundance of Basidiomycota was the highest in the habitats of P. armeniacum (74.29%), followed by P. micranthum (60.46%), P. wenshanense (56.54%), P. malipoense (40.26%), P. emersonii (37.32%), P. bellatulum (29.71%), and P. concolor (23.58%). The relative abundance of Ascomycota was highest in the soils of the habitat of P. concolor (66.46%), followed by P. emersonii (55.90%), P. bellatulum (52.82%), P. malipoense (41.02%), P. wenshanense (38.89%), P. micranthum (29.06%) and P. armeniacum (24.51%). It is worth noting that, except for the two dominant phyla, the relative abundance of the remaining dominant soil fungal phyla in the habitats of all species in subgenus Brachypetalum was less than 20%. At the genus level, soil fungi were abundant in the habitats of subgenus Brachypetalum species. Defined fungal groups were found in the habitats of each species, including Cerella, Archaeorhizomyces, Russula, Filamentum, Sporolium, Porcinella, Penicillium, and Silomonas. There are also a large number of undefined fungal groups and other fungi (). The functional groups of soil fungi in the habitats of each species were similar and divided by trophic type. The functional groups of soil fungi in the habitats of species in subgenus Brachypetalum were all symbiotroph, saprotroph and pathotrophic fungi (). Fungal groups were divided into functional groups based on the utilization of the same resources or the same biological functions. The fungal functional groups in the species habitats were more complex, and the relative abundance of the fungal functional groups varied among different habitats ().
LEfSe (line discriminant analysis (LDA) effect size) of soil fungi in habitats of subgenus Brachypetalum species
When the linear discriminant analysis (LDA) threshold was set to 4 and the classification level was from phylum to genus, a total of 42 biomarkers were identified in the habitats of subgenus Brachypetalum species (), among which 10 biomarkers were identified in the habitat soil of P. micranthum, where Russula_purpureovirescens had the largest contribution to the significant difference among species. P. armeniacum had 11 biomarkers, and Agaricomycetes had the largest LDA value. P. wenshanense had 5 biomarkers, and Boletales had the largest contribution. There were three biomarkers for P. concolor, with Ascomycota contributing the most, while P. malipoense only had two biomarkers, Entoloma and Entolomataceae. There were 6 biomarkers for P. bellatulum, all of which were associated with Sordariomycetes and unidentified fungi. There were 6 biomarkers for P. emersonii, among which the Sordariomycetes class contributed the most. Moving from inside to outside the circle means moving from the phylum to the genus level; yellow indicates no significant differences in subgenus Brachypetalum species, different colors represent different groupings, red in the evolutionary tree node plays an important role in the red group of biological classification, and green nodes for the green groups play an important role in the classification of organisms (). Therefore, it can be concluded that the biomarkers of fungal communities in the habitats of the species of subgenus Brachypetalum play a role at all levels, from phylum to genus ().
Effects of environmental factors on fungal communities in habitats of species in subgenus Brachypetalum
CCA was used to reveal the effects of geographical factors, habitat environmental conditions, climatic factors, soil physicochemical factors and soil enzyme activities on the changes in the fungal community in the habitats of species in subgenus Brachypetalum. The results showed that geographical factors revealed for 8.16% of the changes (), habitat environmental conditions revealed for 10.95% (), and climatic factors revealed for 20.96% (). Soil physicochemical properties revealed for 10.71% (), and soil enzyme activities revealed for 8.64% (). Climatic factors were the most important environmental factors affecting fungal communities. The correlation analysis of dominant soil fungal groups (top 15 dominant groups) and soil physicochemical properties and enzyme activities showed that there was a correlation between them(). Sebacina and phosphatase were significantly negatively correlated. Unclassified_Thelephoraceae and phosphatase, TP, and pH had a negative correlation. Unclassified_Hypocreales was significantly positively correlated with AK and cellulase. Unclassified_Sordariomycetes and phosphatase, TOC, AN, TK, pH were significantly positively correlated. Unclassified_Ascomycota and pH were significantly positively correlated; Archaeorhizomyces and TN, TP, TK, pH were significantly positively correlated. Unclassified_Agaricomycetes was significantly positively correlated with TK and pH.
Figure 10. Effects of environmental factors on soil fungal communities in habitats of species in subgenus Brachypetalum.
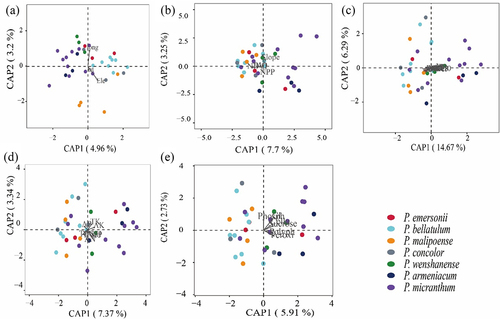
Discussion
As flagship species of ecological conservation, orchids are extremely sensitive to ecological changes.Citation25 Southwest China, as an important distribution area of species in the Brachypetalum subgenus.Citation26 The typical karst development in the region makes the regional ecosystem very fragile.Citation27 The ecological characteristics of the habitats of species in subgenus Brachypetalum, which can survive and reproduce in such a complex and severe environment, deserve further investigation.Citation28 The study revealed differences in soil types among the habitats of different species in subgenus Brachypetalum, which may be related to the landform types of the living environment of each species.Citation29 For example, the preferred soil type of P. armeniacum and P. wenshanense is usually loam soils, while the habitat of P. emersonii is mainly crevices on limestone walls. The other four species in subgenus Brachypetalum tend to live in humus soil. Additionally, this study also found that all species in subgenus Brachypetalum have similar habitat preferences, as they all preferred shady mountain slopes, often grow in negative terrain, which is able to hold water and facilitate soil deposition.Citation30 However, this may also be an important factor limiting population dispersal. This study also shows that there were significant differences among the habitats of different subgenus Brachypetalum species in regard to enzyme activity and soil physical and chemical properties, including organic carbon, total phosphorus, total potassium, pH, sucrase, cellulase, and phosphatase. Soil physical and chemical properties and enzyme activities in species’ growing environment are the common results of large-scale climatic conditions and small-scale environmental factors.Citation31,Citation32 This study found that there were large differences in altitude, topography, habitat characteristics and other factors among all species of subgenus Brachypetalum, and habitat characteristics also differed among different distribution points of the same species, such as vegetation type, slope, and soil type. These findings may be responsible for the differences in soil physical and chemical properties and enzyme activities among species of the subgenus Brachypetalum.
Characteristics of soil fungal communities in habitats of subgenus Brachypetalum species
In general, the fungal communities in the habitats of subgenus Brachypetalum species showed high specificity, and there were only 9 OTUs in the habitats, among which P. bellatulum and P. micranthum habitats had the most OTUs, which may be related to the complex and diverse niche typesCitation33. It was also found that the soil fungal community structure among the habitats of the species of subgenus Brachypetalum was significantly different, although the differences were small. Basidiomycota and Ascomycota were the main fungal groups across the habitats of subgenus Brachypetalum species, but the relative abundance changed with different species. The composition of fungi genera in the habitats of Brachypetalum species was more abundant, with a large number of relatively scarce fungi and unclassified fungi, which may be related to the complex microterrain in karst areas. Previous results showed that soil fungi are very abundant in southwest China.Citation34,Citation35 In addition, subgenus Brachypetalum species gradually cultivate rich fungal communities in their roots during growth, which further diversifies the fungal composition in the habitats of this group.Citation36–38 In this study, when the functional groups of soil fungi in the habitats of subgenus Brachypetalum species were defined according to the trophic type,Citation39 symbiotroph, saprotroph and pathotrophic fungi were the main groups founds, especially the former two, which revealed the demand for symbiotic fungi in the growth process of subgenus Brachypetalum species.Citation40–42 The abundant commensal fungi in the habitat created conditions for the colonization, growth and reproduction of subgenus Brachypetalum species.Citation43 According to the method of resource absorption,Citation44–46 fungi were divided into functional groups in this study. Soil fungi had multiple ecological functions in the habitat of subgenus Brachypetalum species, including Ericoid Mycorrhizal, Plant Parasite, Orchid Mycorrhizal, Epiphyte, Dung Saprotroph, Animal Endosymbiont, Leaf Saprotroph, Arbuscular Mycorrhizal, and Animal Pathogen. AMF have a positive effect on plants under abiotic stress such as drought, high temperature and heavy metals.Citation47 They can enhance plant resistance to abiotic stress by reducing membrane lipid peroxidation and membrane permeability,Citation48,Citation49 The existence of AMF may improve the survival of the subgenus Brachypetalum in extreme environments.Citation50,Citation51 In addition, a variety of saprophytic functions of fungal taxa were found in the habitat of subgenus Brachypetalum species. Southwest China has a high biodiversity and rich saprophytic fungi, which play an important role in the material cycle of the ecosystem and provide nutrients that can be directly absorbed by the subgenus Brachypetalum species. LEfSe showed that soil fungi in the habitats of each species of the subgenus Brachypetalum had different indicator species compositions. Differential indicator species were present from the phylum to genus level. Among them, the habitat of P. armeniacum and P. micranthum had the most differential indicator species. This analysis indicates the presence of unique biomarkers in the soil of the habitat of each species of the subgenus Brachypetalum.
Relationship between changes in soil fungal community structure and environmental variables in habitats of species in subgenus Brachypetalum
This study revealed the influence of a variety of environmental factors on the changes in soil fungal community structure among habitats of subgenus Brachypetalum species, among which climate factors accounted for the highest rate (20.96%). Climate factors have been considered the dominant factors affecting soil fungal changes, and climate variability directly and indirectly affects fungal ecological factors, which include fruit phenology, sporangium production and community composition based on sporangium presence, and climate factors such as light and precipitation directly cause changes in hydrothermal patterns, leading to different degrees of competition, symbiosis and exclusion of various fungi in fungal communitiesCitation52,Citation53 These factors change the structure of fungal communities. It is important to note that the contribution rates of the various environmental factors affecting the habitat soil fungal community composition were low, and the explanation for most of the community composition changes was not revealed and may be related to orchid biological characteristics. Subgenus Brachypetalum species have a typical mycorrhizal fungal structure. In different growth stages, the dominant groups of fungi were not the same, and in different seasons, there were also different fungal community composition structures.Citation53,Citation54 Conversely, the mycorrhizal characteristics of orchids can screen out fungal groups that can coexist and change the fungal community structure in the soil through alternate growth, antagonism, competition and other mechanisms.Citation55,Citation56 Mycorrhizal fungi and endophytic fungi communities in subgenus Brachypetalum species are worthy of further study in the future. They are not only the way through which orchids adapt and survive in the environment but also the main way for orchids to change soil fungal communities in the habitat. This study also revealed advantageous fungal groups in subgenus Brachypetalum species habitats and the relationship between soil physical and chemical properties. The results are is similar to previous studies, namely, the nature of soil material movements can cause changes in fungal community structure, and fungi are important microorganisms in soil.Citation57–60 At the same time, soil fungi play an important role in the soil material energy cycle and soil structure improvement through organic decomposition, nutrient release, metabolic enzymes and other pathways.
Conclusions
This study revealed the habitat characteristics, including the fungal community structure and soil physical and chemical properties, of subgenus Brachypetalum species in southwestern China. It was found that the habitats of subgenus Brachypetalum species had similar physical characteristics, but soil physical and chemical properties and enzyme activity differed significantly among species. The soil fungal community in habitats of subgenus Brachypetalum species had differences in fungal diversity and particular OTUs. Basidiomycetes and ascomycetes were the dominant groups, and the main trophic fungal groups were symbiotrophic fungi and saprophytic fungi. LEfSe showed that there were different indicator species in the fungal communities of the habitats of subgenus Brachypetalum species, both at the phylum and genus levels. This study also identified the environmental factors influencing the changes in fungal community structure among the habitats of subgenus Brachypetalum species, but a high proportion of the variation was not explained, which may be related to the mycorrhizal specificity of subgenus Brachypetalum species, which is worthy of further investigation. This study lays a foundation for the future research and conservation of subgenus Brachypetalum species.
Disclosure statement
No potential conflict of interest was reported by the author(s).
Data availability statement
The data sets used and/or analyzed during the current study are available from the corresponding author on reasonable request.
Additional information
Funding
References
- Cozzolino S, Widmer A. Orchid diversity: an evolutionary consequence of deception? Trends Ecol Evol. 2005;20(9):487–14. doi:10.1016/j.tree.2005.06.004.
- Vishwakarma SK, Singh N, Kumaria S. Genome-wide identification and analysis of the PAL genes from the orchids apostasia shenzhenica, dendrobium catenatum and PHALAENOPSIS EQuestris. J Biomol Struct Dyn. 2021;41(4):1–14. doi:10.1080/07391102.2021.2019120.
- Zhang S, Yang Y, Li J, Qin J, Zhang W, Huang W, Hu H. Physiological diversity of orchids. Plant Divers. 2018;40(4):196–208. doi:10.1016/j.pld.2018.06.003.
- Vladan D, Spyros T, Dmitar L, Jovanović S, Jakovljević K, Stevanović V. Patterns of distribution, abundance and composition of forest terrestrial orchids. Biodivers Conserv. 2020;29(14):4111–4134. doi:10.1007/s10531-020-02067-6.
- Waterman RJ, Bidartondo MI. Deception above, deception below: linking pollination and mycorrhizal biology of orchids. J Exp Bot. 2008;59(5):1085–1096. doi:10.1093/jxb/erm366.
- Chengru L, Na D, Yamei Z, Wu S, Liu Z, Zhai J. A review for the breeding of orchids: current achievements and prospects. Hortic Plant J. 2021;7(5):380–392. doi:10.1016/j.hpj.2021.02.006.
- Yan L, Wang X, Liu H, Tian Y, Lian J, Yang R, Hao S, Wang X, Yang S, Li Q, et al. The genome of Dendrobium officinale illuminates the biology of the important traditional Chinese orchid herb. Mol Plant. 2015;8(6):922–934. doi:10.1016/j.molp.2014.12.011.
- Y GY, B LY, J LZ, Wang X-Q. Evolution and biogeography of the slipper orchids: Eocene vicariance of the conduplicate genera in the old and new world tropics. PLoS One. 2012;7(6):e38788. doi:10.1371/journal.pone.0038788.
- Wen-Ke Y, Tai-Qiang L, Shi-Mao W, Finnegan PM, Gao J-Y. Ex situ seed baiting to isolate germination-enhancing fungi for assisted colonization in Paphiopedilum spicerianum, a critically endangered orchid in China. Global Ecol Conserv. 2020;23:e01147. doi:10.1016/j.gecco.2020.e01147.
- J GZ, B ZS, Y GK, Li S-Y, Hu H. Leaf anatomical structures of Paphiopedilum and Cypripedium and their adaptive significance. J Plant Res. 2011;124(2):289–298. doi:10.1007/s10265-010-0372-z.
- Yuan L, Yang ZL, Li SY, Hu H, Huang JL. Mycorrhizal specificity, preference, and plasticity of six slipper orchids from South Western China. Mycorrhiza. 2010;20(8):559–568. doi:10.1007/s00572-010-0307-5.
- Feng JQ, Huang W, Wang JH, Zhang SB. Different strategies for photosynthetic regulation under fluctuating light in two sympatric Paphiopedilum species. Cells. 2021;10(6):1451. doi:10.3390/cells10061451.
- Zeng S, Huang W, Wu K, Zhang J, da Silva JAT, Duan J. In vitro propagation of Paphiopedilum orchids. Crit Rev Biotechnol. 2016;36(3):521–534. doi:10.3109/07388551.2014.993585.
- Nontachaiyapoom S, Sasirat S, Manoch L. Isolation and identification of Rhizoctonia-like fungi from roots of three orchid genera Paphiopedilum, Dendrobium, And Cymbidium, Collected In Chiang Rai And Chiang Mai Provinces Of Thailand[j]. Mycorrhiza. 2010;20(7):459–471. doi:10.1007/s00572-010-0297-3.
- C YP, Wu J, An M, Chen H, Zhao X, Jin X, Si Q. Geographical distribution and relationship with environmental factors of Paphiopedilum subgenus Brachypetalum Hallier (Orchidaceae) Taxa in Southwest China. Diversity. 2021;13(12):634. doi:10.3390/d13120634.
- Liang L, Kangning X. Study on peak cluster-depression rocky desertification landscape evolution and human activity-influence in South of China. Eur J Remote Sens. 2020;54(2):309–317. doi:10.1080/22797254.2020.1777588.
- Bao SD. Agrochemical analysis of soil [M]. China: China Agriculture Press; 2000.
- Dai M, Hamel C, D BL, Arnaud MS, Grant CA, Lupwayi NZ, Malhi SS, Lemke R. Negative and positive contributions of arbuscular mycorrhizal fungal taxa to wheat production and nutrient uptake efficiency in organic and conventional systems in the Canadian prairie. Soil Biol Biochem. 2014;74:156–166. doi:10.1016/j.soilbio.2014.03.016.
- De Beenhouwer M, Van Geel M, Ceulemans T, Muleta D, Lievens B, Honnay O. Changing soil characteristics alter the arbuscular mycorrhizal fungi communities of Arabica coffee (Coffea arabica) in Ethiopia across a management intensity gradient. Soil Biol Biochem. 2015;91:133–139. doi:10.1016/j.soilbio.2015.08.037.
- Bolger AM, Lohse M, Usadel B. Trimmomatic: a flexible trimmer for Illumina sequence data. Bioinformatics. 2014;30(15):2114–2120. doi:10.1093/bioinformatics/btu170.
- Martin M. Cutadapt removes adapter sequences from high-throughput sequencing reads. EMBnet Journal. 2011;17(1):10–12. doi:10.14806/ej.17.1.200.
- Edgar RC. UPARSE: highly accurate OTU sequences from microbial amplicon reads. Nat Methods. 2013;10(10):996–998. doi:10.1038/nmeth.2604.
- C ER, J HB, C CJ, Quince C, Knight R. UCHIME improves sensitivity and speed of chimera detection. Bioinformatics (Oxford, England). 2011;27(16):2194–2200. doi:10.1093/bioinformatics/btr381.
- Segata N, Izard J, Waldron L, Gevers D, Miropolsky L, Garrett WS, Huttenhower C. Metagenomic biomarker discovery and explanation. Genome Biol. 2011;12(6):1–18. doi:10.1186/gb-2011-12-6-r60.
- Jacquemyn H, Waud M, Brys R, Lallemand F, Courty PE, Robionek A, Selosse MA. Mycorrhizal associations and trophic modes in coexisting orchids: an ecological continuum between auto- and mixotrophy. Front Plant Sci. 2017;8:1497. doi:10.3389/fpls.2017.01497.
- Pei J, Yang W, Cai Y, Yi Y, Li X. Relationship between Vegetation and environment in an arid-hot valley in Southwestern China. Sustainability. 2018;10(12):4774. doi:10.3390/su10124774.
- Sinan L, Xiaoqing Z, Junwei P, Miao P, Wang Q, Tan K. Optimize and control territorial spatial functional areas to improve the ecological stability and total environment in karst areas of Southwest China. Land Use Policy. 2021;100:104940. doi:10.1016/j.landusepol.2020.104940.
- Tsai CC, Liao PC, Ko YZ, Chen CH, Chiang YC. Phylogeny and historical biogeography of paphiopedilum pfitzer (Orchidaceae) based on nuclear and plastid DNA. Front Plant Sci. 2020;11:126. doi:10.3389/fpls.2020.00126.
- Meng Z, Qi F, Yanyan Q, Cao J, Zhang M, Liu W, Deo RC, Zhang C, Li R, Li B, et al. The role of topography in shaping the spatial patterns of soil organic carbon. Catena. 2019;176:296–305. doi:10.1016/j.catena.2019.01.029.
- Li Y, Xiong K, Liu Z, Li K, Luo D. Distribution and influencing factors of soil organic carbon in a typical karst catchment undergoing natural restoration. Catena. 2022;212:106078. doi:10.1016/j.catena.2022.106078.
- A GT, D SS, J MA, Jeong J, Hamilton DP. Terrain effects on the spatial variability of soil physical and chemical properties. Soil Systems. 2019;4(1):1. doi:10.3390/soilsystems4010001.
- Wiesmeier M, Urbanski L, Hobley E, Lang B, von Lützow M, Marin-Spiotta E, van Wesemael B, Rabot E, Ließ M, Garcia-Franco N, et al. Soil organic carbon storage as a key function of soils - a review of drivers and indicators at various scales. Geoderma. 2019;333:149–162. doi:10.1016/j.geoderma.2018.07.026.
- Shen C, Wang J, Z HJ, Yu F-H, Ge Y. Plant diversity enhances soil fungal diversity and microbial resistance to plant invasion. Appl Environ Microbiol. 2021;87(11):e00251–21. doi:10.1128/AEM.00251-21.
- Ning H, Hui L, Zheng T, Li Z, Li G, Jiang Y, Hu X, Lou Y. Community size, activity and C: n stoichiometry of soil microorganisms following reforestation in a Karst region. Eur J Soil Biol. 2016;73:77–83. doi:10.1016/j.ejsobi.2016.01.007.
- Wang Y, Li S, Lang X, Huang X, Su J. Effects of microtopography on soil fungal community diversity, composition, and assembly in a subtropical monsoon evergreen broadleaf forest of Southwest China. Catena. 2022;211:106025. doi:10.1016/j.catena.2022.106025.
- Herrera H, I GR, Meneses C, Pereira G, Arriagada C. Orchid mycorrhizal interactions on the pacific side of the Andes from Chile. a review. J Soil Sci Plant Nutr. 2019;19:187–202. doi:10.1007/s42729-019-00026-x.
- Izuddin M, Srivathsan A, L LA, Yam TW, Webb EL. Availability of orchid mycorrhizal fungi on roadside trees in a tropical urban landscape. Sci Rep. 2019;9(1):19528. doi:10.1038/s41598-019-56049-y.
- Jaspreet K, Caleb P, Jyotsna S. Host population size is linked to orchid mycorrhizal fungal communities in roots and soil, which are shaped by microenvironment. Mycorrhiza. 2020;31(1):17–30. doi:10.1007/s00572-020-00993-5.
- Yeh CM, Chung K, Liang CK, Tsai WC. New insights into the symbiotic relationship between orchids and fungi. Appl Sci. 2019;9(3):585. doi:10.3390/app9030585.
- Daniel AB, Linda JJ, Stuart DC. Symbiotic bacteria of plant-associated fungi: friends or foes? Curr Opin Plant Biol. 2020;56:1–8. doi:10.1016/j.pbi.2019.10.010.
- Yuan-Yuan M, Xu-Li F, Lv-Rong Z, Shao S-C, Liu Q, Selosse M-A, Gao J-Y. Symbiotic fungi undergo a taxonomic and functional bottleneck during orchid seeds germination: a case study on Dendrobium moniliforme. Symbiosis. 2019;79:205–212. doi:10.1007/s13199-019-00647-x.
- J RD, G DJ, Francis R, Ligrone R, Russell A. Symbiotic fungal associations in ‘lower’ land plants. Philosophical transactions of the royal society of London. Series B Biol Sci. 2000;355(1398):815–831. doi:10.1098/rstb.2000.0617.
- Davide F, R SQV, Jasper VR, Termorshuizen AJ, Cotton TEA, Dumbrell AJ, Raaijmakers JM, Weigelt A, Mommer L. Plant functional group drives the community structure of saprophytic fungi in a grassland biodiversity experiment. Plant Soil. 2020;461(1–2):91–105. doi:10.1007/s11104-020-04454-y.
- García-Garrido JM, Ocampo JA. Regulation of the plant defence response in arbuscular mycorrhizal symbiosis. J Exp Bot. 2002;53(373):1377–1386. doi:10.1093/jxb/53.373.1377.
- John NK. Variation in plant response to native and exotic arbuscular mycorrhizal fungi. Ecology. 2003;84(9):2292–2301. doi:10.1890/02-0413.
- Antoine S, Hériché M, Boussageon R, Noceto P-A, van Tuinen D, Wipf D, Courty PE. A historical perspective on mycorrhizal mutualism emphasizing arbuscular mycorrhizas and their emerging challenges. Mycorrhiza. 2021;31(6):1–17. doi:10.1007/s00572-021-01053-2.
- Monika R, Zofia P. Prospects for arbuscular mycorrhizal fungi (AMF) to assist in phytoremediation of soil hydrocarbon contaminants. Chemosphere. 2016;162:105–116. doi:10.1016/j.chemosphere.2016.07.071.
- Ai YJ, Li FP, Yang JQ, Lu S, Gu HH. Research progress and potential functions of AMF and GRSP in the ecological remediation of metal tailings. Sustainability. 2022;14(15):9611. doi:10.3390/su14159611.
- Naheeda B, A MA, Yunyun S, Lei Y, Mustafa NSA, Ahmad P, Zhang L. Improved drought tolerance by AMF inoculation in Maize (Zea mays) involves physiological and biochemical implications. Plants. 2019;8(12):579. doi:10.3390/plants8120579.
- Xiao D, He X, Zhang W, Cheng M, Hu P, Wang K. Diazotroph and arbuscular mycorrhizal fungal diversity and community composition responses to karst and non-karst soils. Appl Soil Ecol. 2022;170:104227. doi:10.1016/j.apsoil.2021.104227.
- Xiao D, Chen Y, He X, Xu Z, Bai SH, Zhang W, Wang K. Temperature and precipitation significantly influence the interactions between arbuscular mycorrhizal fungi and diazotrophs in karst ecosystems. For Ecol Manage. 2021;497:119464. doi:10.1016/j.foreco.2021.119464.
- Suryanarayanan TS, Shaanker RU. Can fungal endophytes fast-track plant adaptations to climate change? Fungal Ecol. 2021;50:101039. doi:10.1016/j.funeco.2021.101039.
- Shi Y, Zhang K, Li Q, Liu X, He J-S, Chu H. Interannual climate variability and altered precipitation influence the soil microbial community structure in a Tibetan Plateau grassland. Sci Total Environ. 2019;714:136794. doi:10.1016/j.scitotenv.2020.136794.
- Jacquemyn H, Waud M, Merckx VS, Lievens B, Brys R. Mycorrhizal diversity, seed germination and long-term changes in population size across nine populations of the terrestrial orchid Neottia ovata. Mol Ecol. 2015;24(13):3269–3280. doi:10.1111/mec.13236.
- K SS, Davison J, Jairus T, Vasar M, Moora M, Zobel M, Öpik M. Non-random association patterns in a plant–mycorrhizal fungal network reveal host–symbiont specificity. Mol Ecol. 2018;28(2):365–378. doi:10.1111/mec.14924.
- J TY, Y ZD, Dai J, Li Y, Xing Y-M, Guo S-X, Chen J. Potential specificity between mycorrhizal fungi isolated from Widespread Dendrobium spp. and Rare D. huoshanense seeds. Curr Microbiol. 2022;79(9). doi:10.1007/s00284-022-02952-z.
- Xue PP, Carrillo Y, Pino V, Minasny B, McBratney AB. Soil properties drive microbial community structure in a large scale transect in South Eastern Australia. Sci Rep. 2018;8(1):11725. doi:10.1038/s41598-018-30005-8.
- Qi D, Wieneke X, Xue P, He L, DeSilva U. Total nitrogen is the main soil property associated with soil fungal community in karst rocky desertification regions in southwest China. Sci Rep. 2021;11(1):1–12. doi:10.1038/s41598-021-89448-1.
- Yongchun L, Yongfu L, C SX, Liang X, Qin H, Chen J, Xu Q. Linking soil fungal community structure and function to soil organic carbon chemical composition in intensively managed subtropical bamboo forests. Soil Biol Biochem. 2017;107:19–31. doi:10.1016/j.soilbio.2016.12.024.
- Hussnain M, Chiao-Ming L, W RF, Cheng L-C, Ko M-C, Lin Y-P. Climate and land cover shape the fungal community structure in topsoil. Sci Total Environ. 2021;751:141721. doi:10.1016/j.scitotenv.2020.141721.