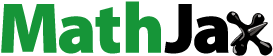
ABSTRACT
Objective
This study aimed to investigate the regulatory effects of exogenous hydrogen sulfide (H2S) on seed germination, seedling growth, and reactive oxygen species (ROS) homeostasis in alfalfa under chromium (Cr) ion (III) stress.
Methods
The effects of 0–4 mM Cr(III) on the germination and seedling growth of alfalfa were first assessed. Subsequently, following seed NaHS immersion, the influence of H2S on alfalfa seed germination and seedling growth under 2 mM Cr(III) stress was investigated, and the substance contents and enzyme activities associated with ROS metabolism were quantified.
Results
Compared to the control group, alfalfa plant germination was delayed under 2 mM Cr(III) stress for up to 48 h (p < 0.05). At 120 h, the total seedling length was approximately halved, and the root length was roughly one-third of the control. Treatment with 0.02–0.1 mM NaHS alleviated the delay in germination and root growth inhibition caused by 2 mM Cr(III) stress, resulting in an increased ratio of root length to hypocotyl length from 0.57 to 1 above. Additionally, immersion in 0.05 mM NaHS reduced hydrogen peroxide (H2O2) and oxygen-free radicals (O2· –) levels (p < 0.05), boosted glutathione (GSH) levels (p < 0.05), and notably enhanced catalase (CAT), ascorbate peroxidase (APX), and glutathione reductase (GR) activities (p < 0.05) compared to the 2 mM Cr(III) stress treatment group.
Conclusion
Seed immersion in NaHS mitigated the delay in germination and inhibition of root elongation under 2 mM Cr(III) stress. This effect is likely attributed to the regulation of intracellular ROS homeostasis and redox balance through enzymatic and non-enzymatic systems; thus, providing a potential mechanism for combating oxidative stress.
1. Introduction
Alfalfa (Medicago sativa L.) is widely grown as a forage species due to its excellent tolerance to saline‒alkali conditions and adaptability, making it a preferred choice for cultivation.Citation1 It has been extensively utilized and shows significant potential for soil remediation and environmental protection.Citation2,Citation3 However, in Cr(III)-contaminated soil,with a pH exceeding four, Cr(III) tends to become less soluble form (CrOH2t, Cr(OH)2*, Cr(OH)3) and bind with organic materials as the pH increases.Citation4 Further, in the presence of reducing agent, Cr(III) in turn forms stable soluble complexes in acidic conditions.Citation4 This occurrence adversely affects the normal growth and development of plants like alfalfa, compromising their overall vigor and productivity.Citation5–7 Compared to plants exposed to Cr(VI), rice seedling tissues exhibit a higher capacity for Cr accumulation under exposure Cr(III).Citation8 These factors should be considered when cultivating alfalfa in potentially Cr-contaminated areas. Further research into methods to mitigate Cr(III) impact on alfalfa growth could ensure its continued success as a valuable forage crop.
Currently, scientists are exploring innovative approaches to mitigate nonbiological stress effects on plants.Citation9–11 Seed priming can improve seed germination and seedling establishment in response to harsh environmental conditions, including significant abiotic and biotic stresses.Citation12 H2S signaling molecules have been shown to induce stress tolerance by modulating antioxidant activity, reducing glutathione levels, increasing osmotic regulator accumulation, and enhancing expression of stress-related genes and cell signaling proteins.Citation13,Citation14 Pretreating Arabidopsis with H2S before drought stress regulates anthocyanin, proline, and hydrogen peroxide levels, amino acid metabolism, autophagy, protein ubiquitination, and redox homeostasis via protein persulfidation.Citation15 Under salt stress, the H2S-regulated protein persulfidation enhances the activity of Glucose-6-phosphate dehydrogenases (G6PDs) in both Arabidopsis and tomato cell sap by inducing structural changes in the homotetrameric form of cytosolic G6PD, thereby mitigating oxidative damage.Citation16 Under toxic metal stress, H2S modifies metal transporter proteins, enhances antioxidant capacity, and participates in protein S-sulfhydration and microRNA activity to counteract toxic metal stress.Citation17 NaHS, an exogenous H2S donor, partially alleviates Cr(VI) stress-induced growth inhibition in Zea mays L., indicating the protective role of H2S signaling in plants facing heavy metal contamination.Citation18 Additionally, H2S reduces lipid peroxidation, improves growth inhibition, and alleviates metal toxicity in alfalfa plants exposed to lead (Pb) and cadmium (Cd) stress,Citation19,Citation20 highlighting its potential to enhance plant health and resilience to environmental stressors. These findings underscore the potential of H2S as a natural remedy to mitigate heavy metal contamination’s adverse effects on plant growth and development.
It was hypothesized that H2S could potentially mitigate adverse effects and improve the germination and growth of alfalfa seeds by regulating ROS metabolism. To test this hypothesis and understand the underlying mechanism, alfalfa seeds were exposed to various concentrations of Cr(III) solution, and their germination and seedling growth were observed. Subsequently, different doses of the H2S donor NaHS were applied to evaluate the germination rate and root elongation of the seeds, while also assessing ROS production and the response of both antioxidant enzyme systems and non-enzymatic systems. These findings are expected to offer scientific evidence supporting the use of H2S to enhance alfalfa growth in Cr(III)-contaminated soils.
2. Materials and methods
2.1 Materials
The variety of alfalfa utilized in this study was Medicago sativa L. cv. Victoria, which was procured from the Seed Station of Xifeng District, Qingyang City, China. The source of Cr(III) was CrCl3·6 H2O (Kermel, Tianjin, China), and the H2S signaling molecule donor was NaHS (Urchem, Shanghai, China).
2.2 Methods
2.2.1 Seed pretreatment and planting
Alfalfa seeds that were fully grained and free of disease and pests were selected, cleaned, and sterilized with 2% NaClO for 10 min, immersed in purified water for 12 h, and subjected to two-dimensional paper chromatography with different concentrations of Cr(III) (0, 0.5, 1, 2, 3, and 4 mM) in germination boxes (12 cm 12 cm
6 cm), to measure the stress effect of Cr(III). A Cr(III) concentration of 0 was used as a control.
After cleaning and disinfection, the alfalfa seeds were immersed in different concentrations of NaHS (0, 0.02, 0.05, 0.08, 0.10, 0.15, or 0.20 mM) for 12 h and subsequently subjected to two-dimensional paper chromatography in germination boxes containing 2 mM Cr(III) to determine the effect of NaHS immersion on Cr(III) stress. In control samples, NaHS and 2 mM Cr(III) were replaced with distilled water without any treatment.
In every experiment, a total of 50 seeds were sown in each box, with four replicate samples for each treatment. Following planting, the plants were placed in an incubator (BOXUN, GSP-9080MBE, China) at a temperature of 25 ± 0.5°C, humidity of 60% and no light for 48 h, after which they were transferred to an illuminated incubator (GXM, GXM-358C-3, China) with a light intensity of 200 µmol m−2 s−1, a photoperiod of 14 h, and appropriate daily watering. The humidity was maintained at 60%. The relevant indices were measured at the end of the fifth day, and the experiments were repeated 3 times.
2.2.2 Determination of alfalfa seed germination
Using a radicle breaking 1 mm through the seed coat as the germination standard, the growth of the seeds was monitored at 12-hour intervals, and the number of sprouted seeds was recorded at each time point to calculate the germination rate (germination rate = number of germinated seeds/total number of seeds) over a period of 84 h. After 120 h (5 days), photographs were taken, and the total seedling length and root length were measured. Additionally, the ratio of root length to hypocotyl length (ratio = root length/total seedling length) was calculated. All photographs were captured using a Power Shot SX30IS digital camera.
2.2.3 Determination of ROS and peroxidation products of membrane lipids
The concentration of H2O2 was determined using the xylenol orange method described by Gay et al.Citation21 O2· – was measured following the method developed by Elstner et al.Citation22 Malondialdehyde (MDA) was quantified employing the thiobarbituric acid (TBA) assay outlined by Yang et al.Citation23
2.2.4 Determination of enzyme activity and substance content of the ROS scavenging system
The activities of superoxide dismutase (SOD), CAT, peroxidase (POD), APX, and GR were determined following the methods outlined in the studies by Amooaghaie,Citation24 FosterCitation25 and Oloumi.Citation10 Alfalfa seedlings (0.5 g) were weighed, homogenized with 5 mL of phosphate buffer (50 mM PBS, pH 7.8) on ice, and then centrifuged at 10,000 × g for 15 min at 4°C. The resulting supernatant was collected to evaluate the activities of SOD, CAT, POD, and GR. SOD activity was assessed using the NBT photoreduction method at 560 nm, with one unit of enzyme activity defined as 50% inhibition of NBT photoreduction. CAT activity was measured at 240 nm by monitoring H2O2 decomposition, where a decrease in OD of 0.01 per minute was defined as one unit of enzyme activity. POD activity was determined by measuring the increase in OD at 470 nm due to guaiacol oxidation in the presence of H2O2, with one unit of enzyme activity corresponding to an OD increase of 0.01 per minute. GR enzyme activity was evaluated at 340 nm, with one unit of GR defined as the rate of NADPH oxidation and absorbance decrease at 1 µmol min−1. These enzyme activities are expressed in units of U·g−1 FW.
Alfalfa seedlings (0.5 g) were weighed and homogenized by adding 5 mL of phosphate buffer (50 mM K2HPO4-KH2PO4, pH 7.0, containing 2 mmol/L ascorbic acid (AsA) and 0.1 mmol/L EDTA-Na2) on ice and then centrifuged at 10,000×g for 15 min at 4°C. Of supernatant, 100 µL was mixed with 1.9 mL of solution (50 mM PBS, pH 7.0, 0.3 mmol/L AsA), and then 1 mL of H2O2 was added immediately. The change in OD at 290 nm was measured to calculate the APX enzyme activity.
The GSH content was measured using the dinitrobenzoic acid method,Citation26 and the determination of AsA was based on the method proposed by Jin et al.Citation27 Soluble sugars were quantified using the anthrone colorimetric method;Citation28 soluble protein content was assessed by the Coomassie brilliant blue method;Citation29 and proline content was evaluated through a tninhydrin chromogenic assay.Citation30
2.3 Data processing
SPSS version 20.0 was used for one-way ANOVA. For post hoc testing, Duncan’s multiple range test (DMRT) was utilized with a significance level of p < 0.05 to determine statistically significant differences among groups. Each treatment had 4 independent biological replicates (n = 4).
3. Results
3.1 Effects of Cr(III) on alfalfa seed germination and seedling growth
The statistical outcomes for alfalfa seed germination under Cr(III) stress are detailed in . At a concentration of 0.5 mM, the germination rate surpassed that of the control group from 24 to 84 h, indicating a promotion of germination by Cr. However, at concentrations of 1 mM and 2 mM, the germination rate was lower than the control’s before 48 h, indicating a delay in effect. Additionally, at 3 mM concentration, the seed germination rate remained lower than the control’s until 72 h, indicating an intensified delay effect. Exposure to 4 mM Cr resulted in delayed seed germination, with a final germination rate significantly lower than that of the control group.
Table 1. Germination of alfalfa seeds under 0–4 mM Cr(III) stress for 84 h (germination percentage, %).
Regarding seedling growth, there were no notable differences in the growth of alfalfa seedlings compared to the control group when Cr concentrations were 0.5 mM and 1 mM. The plants exhibited good germ and radicle growth along with two cotyledons (). However, at Cr concentrations ≥2 mM, there was a significant decrease in radicle length, and the radicle turned green. With increasing Cr concentration, there was a rise in the number of ungerminated seeds (). At a Cr concentration of 4 mM, most seeds germinated, but only a few developed radicles ().
Figure 1. Seedling growth of alfalfa plants under 0–4 mM Cr(III) stress for 5 days after planting. a. 0 mM; b. 0.5 mM; c. 1 mM; d. 2 mM; e. 3 mM; f. 4 mM.
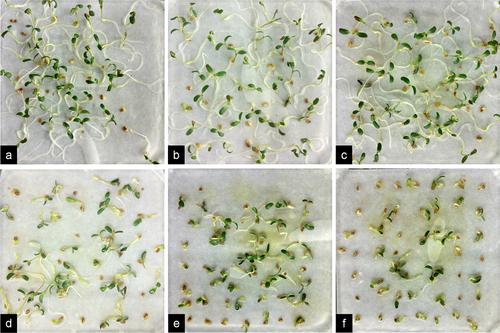
Total length and radicle length were quantified (). At a Cr stress concentration of 0.5 mM, seedlings showed greater total length than those in the control group, while radicle length decreased. This suggests that 0.5 mM Cr primarily promoted seedling growth through hypocotyl elongation, while root elongation was hindered. At concentrations ≥2 mM, plant growth notably declined, with significant decreases in total length and root elongation (p < 0.05). The total length and root length were approximately half and one-third of those in the control group, respectively, at a 2 mM Cr concentration (). Further examination revealed that at concentrations of 3 mM and 4 mM, roots were very short, and root tips were blunt and round ().
Figure 2. Seedling length (a), (b) and comparison of root and Hhypocotyl morphology (c) of alfalfa under 0–4 mM Cr(III) stress for 5 days after seed planting. The different lower case letters above the columns indicate significant differences among the treatments (p <0.05), and the Cr concentrations from left to right are 0, 0.5, 1, 2, 3, and 4 mM in Panel C.
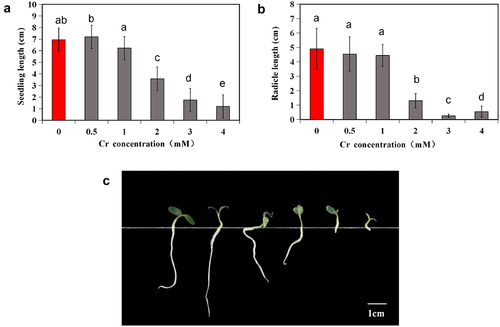
3.2 Effects of exogenous H2S on the germination and seedling growth of alfalfa under 2 mM Cr(III) stress
Seeds were pretreated with the H2S donor NaHS before exposure to 2 mM Cr(III). Analysis of seed germination dynamics suggested that, compared with that in the control group, the seed germination rate in the 0.02–0.15 mM NaHS pretreatment group was consistently greater than that in the 2 mM Cr(III) stress group at each time point, suggesting that exogenous H2S could mitigate the inhibitory effect of Cr(III) stress on seed germination. Notably, the 0.05 mM NaHS treatment had the most pronounced effect ().
Table 2. Effect of NaHS on the germination of alfalfa plants under 2 mM Cr(III) stress within 84 h (germination percentage, %).
Upon thorough examination of the results depicted in , it was noted that treating seeds with NaHS at concentrations ranging from 0.02 to 0.10 mM before exposure to Cr stress resulted in enhanced seedling growth compared to stress alone. Subsequent analysis of the data on seedling length and radicle root length indicated that the total length of plants treated with NaHS at concentrations between 0.05 and 0.15 mM prior to Cr stress treatment was notably greater than that of plants subjected solely to stress (p < 0.05) (). Additionally, radicle length was significantly greater with pretreatment of ≥0.02 mM NaHS compared to single stress conditions (p < 0.05) (). The ratio of radicle root length to hypocotyl length was only 0.57 under the 2 mM Cr treatment (), lower than that of the control, indicating inhibition of root elongation by Cr(III); however, this ratio increased under exogenous H2S treatment, with the effects of the 0.05 and 0.15 mM NaHS treatments being most pronounced. These findings suggest that exogenous H2S has the potential to alleviate the inhibitory effects of Cr stress on root elongation.
Figure 3. Effect of exogenous H2S on the growth of alfalfa plants treated with 2 mM Cr(III) for 5 days after planting. a. CK; b. 2 mM Cr; c. 2 mM Cr +0.02 mM NaHS; d. 2 mM Cr +0.05 mM NaHS; e. 2 mM Cr +0.08 mM NaHS; f. 2 mM Cr +0.10 mM NaHS; g. 2 mM Cr +0.15 mM NaHS; h. 2 mM Cr +0.2 mM NaHS.
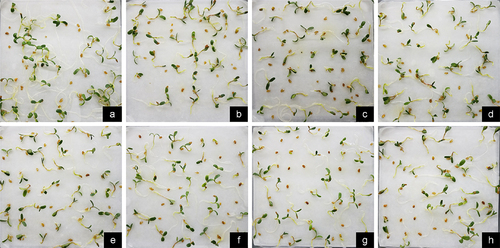
Figure 4. Effects of exogenous H2S on seedling length (a) and radicle length (b) of alfalfa plants treated with 2 mM Cr(III) for 5 days after seed planting. The x-axis represents each treatment group. The different lowercase letters above the columns indicate significant differences among the treatments (p < 0.05), as indicated below.
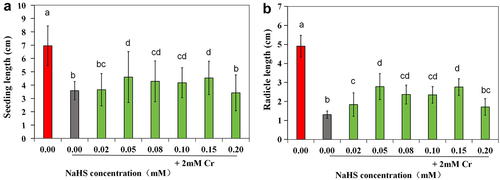
Table 3. Effect of NaHS immersion on the root length:hypocotyl ratio of alfalfa seedlings under 2 mM Cr(III) stress.
3.3 Effects of exogenous H2S on O2· –, H2O2 and MDA contents under 2 mM Cr(III) stress
Compared to the control group, there was a noticeable increase in H2O2 and O2· – levels under 2 mM Cr(III) stress (p < 0.05). Additionally, MDA, proline, soluble sugar, and soluble protein levels were significantly elevated (p < 0.05). Furthermore, compared to the Cr(III) stress group, H2O2 and O2· – levels significantly decreased in seeds treated with 0.05 mM and 0.08 mM NaHS (p < 0.05), with the most significant effect observed in these treatment groups. The MDA content notably decreased (p < 0.05), with no discernible difference between the 0.02 and 0.05 mM NaHS immersion groups and the control group (untreated). Proline and soluble sugar levels were higher than those in the control group (p < 0.05) but significantly lower than those in the Cr(III) stress group (p < 0.05). The soluble protein content was lower than that of the Cr(III) stress group (p < 0.05), with no difference observed between the Cr(III) stress group and the control group (p < 0.05) ().
3.4 Effects of exogenous H2S on the enzyme activities and contents of ROS scavengers in alfalfa under 2 mM Cr(III) stress
Compared with those in the control group, the activities of SOD and CAT were significantly elevated in the 2 mM Cr(III) stress group (p < 0.05), while there were no notable changes in the GSH content or POD, APX, or GR enzyme activity (). SOD activity in the 0.05 mM NaHS treatment group was significantly greater than that in the control group (p < 0.05) but notably lower than that in the stress group (p < 0.05) (). The activity of CAT was significantly greater in the 0.02 mM and 0.05 mM NaHS treatment groups than in the control group (p < 0.05) (). However, when the concentration of NaHS exceeded 0.05 mM, POD activity decreased with increasing NaHS concentration (). APX enzyme activity was also significantly different from that of the control (p < 0.05) (). Furthermore, when the seed concentration of NaHS ranged from 0.02 mM to 0.20 mM, there was an initial increase followed by a decrease in the GSH content, while the content of AsA initially decreased followed by an increase (). Additionally, compared with that in the control group, the GR enzyme activity in the 0.02 mM, 0.05 mM, 0.10 mM and 0.15 mM NaHS treatment groups significantly increased (p < 0.05) ().
4. Discussion
At low concentrations (≤0.5 mM), Cr(III) has been observed to enhance the growth of alfalfa seedlings, consistent with findings in wheat by Chen et al.,Citation31 while not significantly affecting the growth of sorghum plants.Citation32 Under 2 mM Cr(III) stress, alfalfa seed germination was delayed, resulting in a lower germination level compared to the control until 48 h. After 5 days of planting, the total seedling length was approximately half that of the control, with the root length being around one-third. Additionally, root tips showed a blunt and round shape, indicating inhibition of seed germination and impact on both root elongation and root tip development. Scoccianti et al. also noted a similar trend in celery, where the inhibitory effect of 0.01 to 1 mM Cr(III) on seed germination and hypocotyl elongation increased with concentration, with complete blockage observed at 10 mM.Citation33 However, even at the lowest Cr(III) dose, celery seedling roots suffered severe damage, highlighting alfalfa’s relatively high tolerance to Cr(III).
Strategies such as neodymium and silicon have been proposed to mitigate Cr(III) stress effects and enhance plant tolerance.Citation7,Citation34 Additionally, H2S, a signaling molecule, has been found to boost chlorophyll and biomass levels in alfalfaCitation35 and enhance growth and yield in soilless-cultivated strawberry plants.Citation36 Moreover, H2S has been demonstrated to stimulate alfalfa hypocotyl elongation by influencing cellulose content and fibril arrangement.Citation37 Treating alfalfa seeds with the exogenous H2S donor NaHS at concentrations of 0.02, 0.05, and 0.10 mM before planting alleviated the delay in seed germination induced by 2 mM Cr(III) stress. Even at 36 h, there was no significant difference in seed germination rate compared to the control, indicating an overall improvement. Statistical analysis revealed that exogenous H2S signaling boosted total and root length under Cr(III) stress, along with the ratio of root length to hypocotyl, suggesting alleviation of the inhibitory effect of 2 mM Cr(III) stress on root elongation. At NaHS concentrations of 0.05 mM and 0.15 mM, seedling total length, radicle length, and the root length to hypocotyl ratio peaked, indicating significant weakening of NaHS’s inhibitory effect on root elongation. Similarly, other studies have reported that exogenous H2S can alleviate growth inhibition caused by abiotic stress in plants like Miscanthus sacchariflorus, strawberry plants, and foxtail millet.Citation38–40 When plants encounter metal stress, an excess of metal ions can induce osmotic stress and ion toxicity, resulting in the accumulation of ROS, which compromises cell membrane integrity.Citation41,Citation42 This triggers plants to counteract oxidative damage and protect themselves through both enzymatic pathways (such as SOD, CAT, POD, and APX) and non-enzymatic pathways (such as AsA and GSH).Citation6,Citation7,Citation43,Citation44 Different growth stages and metal ion stresses prompt plants to employ various defense mechanisms against ROS and maintain ROS balance.Citation32,Citation45 This study revealed that treating seeds with suitable NaHS concentrations before exposure to 2 mM Cr(III) stress reduced the accumulation of H2O2 and O2· – induced by stress. The most significant effect was observed with NaHS concentrations between 0.05 and 0.10 mM. Under 2 mM Cr(III) stress, MDA levels increased, indicating oxidative damage to membrane lipids. Consequently, intracellular osmotic regulatory substances like proline, soluble sugars, and soluble proteins increased significantly to preserve homeostasis and shield cells from oxidative damage and other harmful factors affecting their structure and function. After treatment with 0.02 and 0.05 mM NaHS, a marked decrease in MDA levels was noted, suggesting a reduction in oxidative damage to membrane lipids. This could be attributed to increased H2S content following NaHS immersion, directly involved in ROS scavenging or enhancing ROS scavenging capability. Additionally, there was no significant decrease in proline or soluble sugar content, indicating tissue possessed osmoregulatory mechanisms to resist oxidative damage and protect itself.
Exposure to Cr(III) adversely affects plant growth and morphology. Excessive Cr(III) can disrupt various plant metabolic processes, leading to chlorosis, necrosis, impaired photosynthesis, and eventual mortality.Citation6,Citation33 Lin et al. demonstrated that rice plants, in the presence of sodium hydrosulfide,Citation45 mitigated the impact of Cr(III) stress through both enzymatic and non-enzymatic pathways. Under Cr(III) stress, the activities of SOD, CAT, POD, and APX in alfalfa seedlings changed following NaHS immersion, with changes correlated with NaHS concentration. For instance, at a NaHS concentration of 0.02 mM, CAT, POD, and APX enzyme activities were significantly higher than in the 2 mM Cr(III) stress group (p < 0.05). At 0.05 mM NaHS, CAT and APX enzyme activities were also notably higher than in the 2 mM Cr(III) stress group (p < 0.05). These findings suggested NaHS immersion increased ROS scavenging enzyme activities under 2 mM Cr(III) stress. Zhang and Guo et al. similarly reported increased proline content and antioxidant enzyme activity after NaHS pretreatment in foxtail millet seedlings under salt stress and Salix matsudana Koidz under cadmium stress.Citation9,Citation39 AsA and GSH, two antioxidants, directly eliminate ROS through the ASA-GSH cycle, with GR playing a vital role in maintaining AsA and GSH redox balance.Citation46 Analysis of GSH and AsA contents and GR enzyme activity revealed the highest GSH level and lowest AsA level at 0.05–0.10 mM NaHS immersion. Additionally, GR enzyme activity at NaHS immersion concentrations of 0.02 mM, 0.05 mM, 0.10 mM, and 0.15 mM was significantly higher than the control (p < 0.05), indicating increased non-enzymatic ROS scavenging system activity under 2 mM Cr(III) stress. Thus, we hypothesize that exogenous H2S signaling may enhance alfalfa plant survival under 2 mM Cr(III) stress by activating both ROS scavenging enzymatic and non-enzymatic systems to counteract oxidative damage and maintain intracellular redox balance.
H2S may improve photosynthetic electron transport efficiency, potentially reducing ROS generation,Citation47 and upregulating antioxidant enzyme expression.Citation48 This could contribute to improved ROS metabolism balance and redox homeostasis maintenance. Yu et al. studied trivalent Cr(III)-treated rice plants, reporting Cr primarily accumulated in roots;Citation49 furthermore, they identified 10 specific OsMT genes regulating ROS removal with differential expression.Citation50 CYTc levels decreased in roots due to metal ion binding and increased in shoots, possibly due to ROS accumulation, serving as a signal for severe growth inhibition.Citation51 Additionally, cytochrome c oxidase (COX) gene family members, including COX1, COX2, COX5B, COX6A, COX6B, and COX7A, exhibited differential expression.Citation52 However, further investigation is needed to understand the molecular mechanism by which H2S affects the antioxidant system of alfalfa under Cr(III) stress.
5. Conclusion
In conclusion, exposure to Cr(III) stress led to delayed or inhibited seed germination and affected root elongation and root tip development in alfalfa. Presoaking alfalfa seeds with NaHS before planting was observed to alleviate the delayed germination and inhibited root elongation of alfalfa seeds under 2 mM Cr(III) stress. Subsequent investigations indicated that this effect was linked to the activation of intracellular antioxidant enzyme systems and non-enzymatic systems, which function to defend against oxidative damage and maintain the intracellular redox balance in alfalfa seedlings. Considering the role of NaHS, it is suggested that pretreating alfalfa seeds with 0.05 mM NaHS could effectively promote seed germination, enhance root elongation, and maintain the balance of ROS metabolism under 2 mM Cr(III) stress.
Contributions
TB and JY: conception and design, manuscript writing, writing-review and editing, and final approval of the manuscript. JL: project administration. TB: investigation, collection and assembly of data, data analysis and interpretation. TB and XF: conception and design, data analysis and interpretation, writing-original draft preparation. All authors have read and agreed to the published version of the manuscript.
Disclosure statement
No potential conflict of interest was reported by the author(s).
Additional information
Funding
References
- Lesins KA, Lesins I. Genus Medicago (Leguminosae): a taxogenetic study. The Hague: Dr. W, Junk Publishers; 2012.
- Chekol T, Vough LR. A study of the use of alfalfa (Medicago sativa L.) for the phytoremediation of organic contaminants in soil. Rem J. 2001;11(4):89–10. doi:10.1002/rem.1017.
- El-Ramady H, Abdalla N, Kovacs S, Domokos-Szabolcsy É, Bákonyi N, Fari M, Geilfus, C.M., Alfalfa growth under changing environments: an overview. Environ Biodivers Soil Secur. 2020;4(2020):201–224.
- Ertani A, Mietto A, Borin M, Nardi S. Chromium in agricultural soils and crops: a review. Water Air Soil Pollut. 2017;228(5):1–12. doi:10.1007/s11270-017-3356-y.
- Shafique F, Ali Q, Saleem MZ, Bhatti Y, Zikrea A, Malik D. Effect of manganese and chromium toxicity on growth and photosynthetic pigments of maize. Plant Cell Biotechnol Mol Bio. 2021;21:58–64.
- Rahman MM, Rahman MM, Islam KS, Chongling Y. Effect of chromium stress on antioxidative enzymes and malondialdehyde content activities in leaves and roots of mangrove seedlings Kandelia candel (L.) Druce. J For Environ Sci. 2010;26(3):171–179.
- Lu NH, Wu LM, Yang R, Li H, Shan CJ. Neodymium improves the activity of ascorbate-glutathione cycle and chloroplast function of wheat seedlings under chromium stress. Photosynthetica. 2020;58(3):748–754. doi:10.32615/ps.2020.024.
- Yu X-Z, Feng Y-X, Liang Y-P. Kinetics of phyto-accumulation of hexavalent and trivalent chromium in rice seedlings. Int Biodeterior Biodegradation. 2018;128:72–77. doi:10.1016/j.ibiod.2016.09.003.
- Guo L, Ling L, Wang X, Cheng T, Wang H, Ruan Y. Exogenous hydrogen sulfide and methylglyoxal alleviate cadmium-induced oxidative stress in Salix matsudana Koidz by regulating glutathione metabolism. BMC Plant Biol. 2023;23(1):1–16. doi:10.1186/s12870-023-04089-y.
- Oloumi H, Maleki M, Habibipour L, Lotfi S. Foliar application of NaHS alleviates Cd toxicity in soybean plants through regulation of Glutathione metabolism. Plant Stress. 2024;11:100363–100371. doi:10.1016/j.stress.2024.100363.
- Kaya C, Shabala S. Sodium hydrosulfide-mediated upregulation of nitrogen metabolism improves drought stress tolerance in pepper plants. Environ Exp Bot. 2023;209:105305–105319. doi:10.1016/j.envexpbot.2023.105305.
- Zulfiqar F. Effect of seed priming on horticultural crops. Sci Hortic (Amsterdam). 2021;286:110197–110203. doi:10.1016/j.scienta.2021.110197.
- Zulfiqar F, Ashraf M. Antioxidants as modulators of arsenic-induced oxidative stress tolerance in plants: An overview. J Hazard Mater. 2022;427:127891–1279109. doi:10.1016/j.jhazmat.2021.127891.
- Zulfiqar F, Hancock JT. Hydrogen sulfide in horticulture: emerging roles in the era of climate change. J Plant Physiol Biohem. 2020;155:667–675. doi:10.1016/j.plaphy.2020.08.010.
- Jurado-Flores A, Aroca A, Romero LC, Gotor C, Kopriva S, Kopriva S. Sulfide promotes tolerance to drought through protein persulfidation in Arabidopsis. J Exp Bot. 2023;74(15):4654–4669. doi:10.1093/jxb/erad165.
- Wang X, Shi C, Hu Y, Ma Y, Yi Y, Jia H, Li F, Sun H, Li T, Wang X. et al. Persulfidation maintains cytosolic G6PDs activity through changing tetrameric structure and competing cysteine sulfur oxidation under salt stress in Arabidopsis and tomato. New Phytol. 2023;240(2):626–643. doi:10.1111/nph.19188.
- Amist N, Singh N. Regulation of metal stress toxicity in plants by the hydrogen sulfide. In: Hydrogen Sulfide in plant biology. Elsevier Inc; 2021. p. 87–102. doi:10.1016/B978-0-323-85862-5.00013-0.
- Yang X, Ren J, Yang W, Xue J, Gao Z, Yang Z. Hydrogen sulfide alleviates chromium toxicity by promoting chromium sequestration and re-establishing redox homeostasis in Zea mays L. Environ Pollut. 2023;332:121958–121999. doi:10.1016/j.envpol.2023.121958.
- Li L, Wang Y, Shen W. Roles of hydrogen sulfide and nitric oxide in the alleviation of cadmium-induced oxidative damage in alfalfa seedling roots. BioMetals. 2012;25(3):617–631. doi:10.1007/s10534-012-9551-9.
- Yang X, Kong L, Wang Y, Su J, Shen W. Methane control of cadmium tolerance in alfalfa roots requires hydrogen sulfide. Environ Pollut. 2021;284:117123–117136. doi:10.1016/j.envpol.2021.117123.
- Gay C, Collins J, Gebicki JM. Hydroperoxide assay with the ferric–xylenol orange complex. Analytical Biochem. 1999;273(2):149–155. doi:10.1006/abio.1999.4208.
- Elstner EF, Heupel A. Inhibition of nitrite formation from hydroxylammoniumchloride: a simple assay for superoxide dismutase. Anal Biochem. 1976;70(2):616–620. doi:10.1016/0003-2697(76)90488-7.
- Yang M, Qin B-P, Ma X-L, Wang P, Li M-L, Chen L-I, Chen L-T, Sun A-Q, Wang Z-I, Yin Y-P. Foliar application of sodium hydrosulfide (NaHS), a hydrogen sulfide (H2S) donor, can protect seedlings against heat stress in wheat (Triticum aestivum L.). J Integr Agric. 2016;15(12):2745–2758. doi:10.1016/S2095-3119(16)61358-8.
- Amooaghaie R, Zangene-Madar F, Enteshari S. Role of two-sided crosstalk between NO and H2S on improvement of mineral homeostasis and antioxidative defense in Sesamum indicum under lead stress. Ecotoxicol Environ Saf. 2017;139:210–218. doi:10.1016/j.ecoenv.2017.01.037.
- Foster JG, JLJPp H. Responses of superoxide dismutase and glutathione reductase activities in cotton leaf tissue exposed to an atmosphere enriched in oxygen. Plant Physiol. 1980;66(3):482–487. doi:10.1104/pp.66.3.482.
- Wang H, Zhong X, W-Y S, Guo BJAJo G. Study of malondialdehyde (MDA) content, superoxide dismutase (SOD) and glutathione peroxidase (GSH-Px) activities in chickens infected with avian infectious bronchitis virus. Afr J Biotechnol. 2011;10(45):9213–9217. doi:10.5897/AJB11.782.
- Jin S, Ding Z, Xie J. Study of postharvest quality and antioxidant capacity of freshly cut amaranth after Blue LED light treatment. Plants. 2021;10(8):1614–1627. doi:10.3390/plants10081614.
- Li X, Jjs L. Determination of the content of soluble sugar in sweet corn with optimized anthrone colorimetric method. Storage Process. 2013;13(4):24–27.
- Sedmak JJ, Grossberg SE. A rapid, sensitive, and versatile assay for protein using Coomassie brilliant blue G250. Anal Biochem. 1977;79(1–2):544–552. doi:10.1016/0003-2697(77)90428-6.
- Bates LS, Waldren RA, Teare IJP. Rapid determination of free proline for water-stress studies. Plant Soil. 1973;39(1):205–207. doi:10.1007/BF00018060.
- Chen C-P, Juang K-W, Lin T-H, Lee D-Y. Assessing the phytotoxicity of chromium in Cr(VI)-spiked soils by Cr speciation using XANES and resin extractable Cr(III) and Cr(VI). Plant Soil. 2010;334(1–2):299–309. doi:10.1007/s11104-010-0383-5.
- Shanker AK, Pathmanabhan G. Speciation dependant antioxidative response in roots and leaves of sorghum (Sorghum bicolor (L.) Moench cv CO 27) under Cr (III) and Cr (VI) stress. Plant Soil. 2004;265(1):141–151. doi:10.1007/s11104-005-0332-x.
- Scoccianti V, Crinelli R, Tirillini B, Mancinelli V, Speranza A. Uptake and toxicity of Cr(III) in celery seedlings. Chemosphere. 2006;64(10):1695–1703. doi:10.1016/j.chemosphere.2006.01.005.
- Pang Z, Zhu Y, Guan D-X, Wang Y, Peng H, Liang Y. Unveiling mechanisms of silicon-mediated resistance to chromium stress in rice using a newly-developed hierarchical system. Plant Physiol Bioch. 2024;207:108368–108376. doi:10.1016/j.plaphy.2024.108368.
- Fang L, Ju W, Yang C, Duan C, Cui Y, Han F, Shen G, Zhang C. Application of signaling molecules in reducing metal accumulation in alfalfa and alleviating metal-induced phytotoxicity in Pb/Cd-contaminated soil. Ecotox Environ Safe. 2019;182:109459–109466. doi:10.1016/j.ecoenv.2019.109459.
- Bahmanbiglo FA, Eshghi S. Improving the growth, yield and iron concentration of strawberry using sodium hydrosulfide (NaHS) under soilless culture. J Plant Nutr. 2023;47(5):786–796. doi:10.1080/01904167.2023.2281518.
- Li J, Wang X, Wang X, Ma P, Yin W, Wang Y, Chen Y, Chen S, Jia H. Hydrogen sulfide promotes hypocotyl elongation via increasing cellulose content and changing the arrangement of cellulose fibrils in alfalfa. J Exp Bot. 2020;71(19):5852–5864. doi:10.1093/jxb/eraa318.
- Zhang J, Liang X, Xie S, Liang Y, Liang S, Zhou J, Huang Y. Effects of hydrogen sulfide on the growth and physiological characteristics of Miscanthus sacchariflorus seedlings under cadmium stress. Ecotox Environ Safe. 2023;263:115281–115289. doi:10.1016/j.ecoenv.2023.115281.
- Zhang X, Ding Y, Yang M, Wei A, Huo D. The role of NaHS pretreatment in improving salt stress resistance in foxtail millet seedlings: physiological and molecular mechanisms. Plant Signaling Behav. 2023;18(1):2276611–2276623. doi:10.1080/15592324.2023.2276611.
- Zanganeh R, Jamei R, Rahmani F. Pre-sowing seed treatment with salicylic acid and sodium hydrosulfide confers Pb toxicity tolerance in maize (Zea mays L.). Ecotoxicol Environ Saf. 2020;206:111392–111400. doi:10.1016/j.ecoenv.2020.111392.
- Kharbech O, Houmani H, Chaoui A, Corpas FJ. Alleviation of Cr(VI)-induced oxidative stress in maize (Zea mays L.) seedlings by NO and H 2 S donors through differential organ-dependent regulation of ROS and NADPH-recycling metabolisms. J Plant Physiol. 2017;219:71–80. doi:10.1016/j.jplph.2017.09.010.
- Kaya C, Ashraf M, Akram NA. Hydrogen sulfide regulates the levels of key metabolites and antioxidant defense system to counteract oxidative stress in pepper (Capsicum annuum L.) plants exposed to high zinc regime. Environ Sci Pollut Res. 2018;25(13):12612–12618. doi:10.1007/s11356-018-1510-8.
- Song Y, Lv J, Ma Z, Dong W. The mechanism of alfalfa (Medicago sativa L.) response to abiotic stress. Plant Growth Regul. 2019;89(3):239–249. doi:10.1007/s10725-019-00530-1.
- da Silva Cj, Batista Fontes EP, Modolo LV, da Silva CJ. Salinity-induced accumulation of endogenous H2S and NO is associated with modulation of the antioxidant and redox defense systems in Nicotiana tabacum L. cv. Plant Sci. 2017;256:148–159. doi:10.1016/j.plantsci.2016.12.011.
- Lin Y-J, Feng X-H, Feng Y-X. Regulation of enzymatic and non-enzymatic antioxidants in rice seedlings against chromium stress through sodium hydrosulfide and sodium nitroprusside. Environ Sci Pollut Res. 2022;30(10):25851–25862. doi:10.1007/s11356-022-23917-6.
- Zhang X, Zheng X, Han Y, Yang R, Wang Q, Gong D, Li Y, Prusky D, Bi Y. UV-C irradiation maintains cell membrane integrity at wounds of potato tubers during healing by regulating ROS homeostasis and increasing antioxidant activity. Postharvest Biol Technol. 2023;199:112308–112315. doi:10.1016/j.postharvbio.2023.112308.
- Ozfidan-Konakci C, Yildiztugay E, Elbasan F, Kucukoduk M, Turkan I. Hydrogen sulfide (H2S) and nitric oxide (NO) alleviate cobalt toxicity in wheat (Triticum aestivum L.) by modulating photosynthesis, chloroplastic redox and antioxidant capacity. J Hazard Mater. 2020;388:122061–122075. doi:10.1016/j.jhazmat.2020.122061.
- Li S-P, Hu K-D, Hu L-Y, Li Y-H, Jiang A-M, Xiao F, Han Y, Liu Y-S, Zhang H. Hydrogen sulfide alleviates postharvest senescence of broccoli by modulating antioxidant defense and senescence-related gene expression. J Agr Food Chem. 2014;62(5):1119–1129. doi:10.1021/jf4047122.
- Yu X-Z, Fan W-J, Lin Y-J. Analysis of gene expression profiles for metal tolerance protein in rice seedlings exposed to both the toxic hexavalent chromium and trivalent chromium. Int Biodeterior Biodegradation. 2018;129:102–108. doi:10.1016/j.ibiod.2018.01.011.
- Yu X-Z, Lin Y-J, Zhang Q. Metallothioneins enhance chromium detoxification through scavenging ROS and stimulating metal chelation in Oryza sativa. Chemosphere. 2019;220:300–313. doi:10.1016/j.chemosphere.2018.12.119.
- Yu X-Z, Lu C-J, Li Y-H. Role of cytochrome c in modulating chromium-induced oxidative stress in Oryza sativa. Environ Sci Pollut Res. 2018;25(27):27639–27649. doi:10.1007/s11356-018-2817-1.
- Yu X-Z, Lu C-J, Lin Y-J, Li Y-H. Cr-induced disturbance on expression of six COX genes in rice seedlings. Int J Environ Sci Technol. 2019;16(5):2385–2394. doi:10.1007/s13762-018-1848-y.