ABSTRACT
The reversible photostriction (photomechanical strain) in Ge35S65 chalcogenide thin film deposited by a solvent casting method has been monitored using a fiber Bragg grating (FBG) sensor. The shift in Bragg wavelength is used as a probing parameter to quantitatively measure the photoinduced strain arising because of structural modifications in these films under illumination. Exposure to band gap light (405 nm) and above band gap light (302 and 254 nm) leads to a reversible photostriction effect of the order of 100 µε. The present study shows that FBG sensors can be used to effectively measure the optomechanical actuation in chalcogenide films caused by the reversible photostriction effect in the visible and ultraviolet wavelength region.
Nomenclature
FBG | = | fiber Bragg grating |
nm | = | nanometer |
ChG | = | chalcogenide |
PI | = | photoinduced |
SiN | = | silicon nitride |
KrF | = | krypton fluoride |
UV | = | ultra voilet |
mJ | = | millijoules |
Hz | = | Hertz |
mm | = | millimeter |
GeS | = | Ge35S65 composition |
Tg | = | glass transition temperature |
h | = | hour |
PA | = | n-propyl amine |
mol L−1 | = | moles per litre |
FE-SEM | = | field emission scanning electron microscope |
EDS | = | energy dispersive X-ray spectroscopy |
mL | = | milliliter |
mW | = | milliwatt |
cm | = | centimeter |
λB | = | Bragg wavelength |
neff | = | effective refractive index |
ʌ | = | grating pitch |
ΔλB | = | Bragg wavelength shift |
pm | = | picometer |
µm | = | micrometer |
ε | = | photoinduced strain |
min | = | minute |
s | = | second |
με | = | micro-strain |
1. Introduction
Chalcogenide (ChG) glasses are photoactive materials which readily exhibit a variety of photoinduced (PI) effects when subjected to an optical illumination that enable them to be used as optical imaging or storage media. In the category of sulfide-based ChG glassy systems, Ge–S and As–S glasses exhibit a wide variety of photostructural changes when excited with bandgap light. Among these two, the binary Ge–S glassy system has been preferred for our photomechanical studies, as they display several interesting features, such as high photomodification characteristics, high glass transition temperature, and are less hazardous than arsenic-based ChGs[Citation1]. Furthermore, various PI effects such as photodarkening, photobleaching, photoexpansion, photorefraction have been observed earlier in amorphous Ge–S films, as a result of certain structural, chemical changes or the combination of both[Citation2Citation3Citation4].
Similar to other materials, such as ferroelectric compounds, semiconductors, carbon nanotubes, and polymers, ChG films also exhibit the photostriction phenomenon[Citation5,Citation6]. Krecmer et al.[Citation7] and Stuchlik et al.[Citation8] have already demonstrated the polarization-dependent photostriction effect in arsenic-based ChG thin film deposited on SiN cantilevers. However, the slow and delayed photoresponse as well as the requirement of high temperature for reversal process, limit the use of ChGs (such as As2Se3, As2S3, As40Se50Ge10) in fabricating effective photostrictive actuators; in addition, it has not been possible to quantitatively measure the photostriction induced them[Citation6]. In recent years, fiber Bragg gratings (FBGs) have been used as efficient physical, chemical and biological sensors due to high sensitivity and fast response to even a small external perturbation[Citation9,Citation10]. FBG acts as a wavelength specific dielectric mirror which reflects a particular wavelength of the incident radiation, called Bragg wavelength. The shift in Bragg wavelength is used as a typical probing parameter to interpret the measurands.
In this work, an FBG sensor has been used for in situ monitoring and quantification of the reversible photostriction in Ge35S65 film which has been coated on the FBG sensor. The main aim of this study is to detect the photoinduced mechanical (especially photoinduced mechanical strain) changes in Ge35S65 ChG film, which can lead to the development of novel optical actuators in optical communications.[Citation9]
2. Experiments
The FBG sensor used have been fabricated using a phase mask and KrF excimer UV laser operating at 248 nm wavelength with pulse energy of 2.56 mJ at 100 Hz. A photosensitive fiber [Fibercore, SM1500 (4.2/80)] has been used to inscribe gratings over a length of 3 mm with a baseline Bragg wavelength around 1550 nm.[Citation11,Citation12] The ChG film has been coated onto the FBG using solvent casting technique. This method is cost and energy effective, having other advantages, such as coverage of a larger area, low-temperature controllability, and smaller surface roughness.[Citation13,Citation14]
To begin with, bulk ChG glass of Ge35S65 composition (GeS) is synthesized by melt quenching technique as described elsewhere.[Citation15,Citation16] Second, a nanocolloidal GeS solution is prepared, by grinding and dissolving the bulk GeS glass in n-propylamine (PA) solvent at 1.5 mol L−1 concentration in a sealed glass container. The solution is stirred for 7 days to obtain uniformity. The resulting solution is sonicated for an hour, and a small amount is dropped onto the FBG region of the optical fiber. The drop-casted region is first annealed at 60°C (which is greater than the boiling point of n-propylamine 45°C) for about 6 h, and subsequently, it is annealed at 150°C (sub-Tg) for an hour to dry off the solvent.
The GeS-coated FBG sensor is characterized using scanning electron microscopy [FE-SEM (Carl Zeiss), ULTRA 55]. The molecular structure of bulk GeS glass and the coated GeS thin film has been investigated using Raman spectroscopy (Renishaw, RM 1000) with an excitation wavelength of 785 nm at 1 mW of laser power using a 50 × objective lens. The optical absorption spectra of GeS solution and PA solvent have been obtained using a UV–vis spectrophotometer (Analytik Jena SPECORD S-600) in the range 250–550 nm, in a standard 3 mL quartz cell having 1 cm path length.
An excitation light source in the UV spectral range has been deployed, to study the photostriction effect since this energy is equal to or higher than chemical bond energy for most of the ChG materials.[Citation17] In the first experiment, a UV-C light source (Philips, TUV 8 W FAM) with a peak wavelength of 254 nm with a power density of ∼53 mW cm−2, and a UV-B band light (Philips, PL-S 9 W/12/2P) with a peak wavelength of 302 nm with a power density of ∼53 mW cm−2 are used for irradiation. The UV-C (254 nm) and UV-B (302 nm) bulbs are covered fully with an aluminum foil with an aperture which matches the length of the FBG sensor created. The fiber with inscribed Bragg grating structure is scanned across the UV lamps very closely, for detecting the grating region of FBG sensor. Once the maximum response for a stipulated period is attained, the UV source and FBG sensors are fixed for further experimentation.
Furthermore, a laser pointer source with wavelength 405 nm having a spot size of about 3 mm diameter and maximum power of 95 mW has been utilized. The power control of the 405 nm light has been enabled using external neutral density filters. All the light excitation experiments have been conducted at room temperature and by placing the pump light perpendicular to the axis of the GeS-coated FBG sensor.
The schematic of the experimental setup is shown in . When a broadband source (1510–1590 nm) is passed through the core of the GeS-coated FBG sensor, a particular wavelength (λB) which satisfies Bragg’s condition is reflected back from the gratings,[Citation18] which is given by
3. Results and discussion
The FE-SEM images obtained, confirm the presence of GeS film along the fiber and the thickness of the coated GeS film is ∼16 µm. shows the longitudinal view of the GeS-coated FBG, respectively. The shows the EDS image of the small inset portion (spectrum 1) of the FE-SEM of GeS film coated along the fiber. The EDS analysis shows that there is a decrease in the sulfur content (60.32%) and an increase in germanium content (39.68%) in the GeS film coated on the optical fiber [].
Figure 2. (a) FE-SEM image of the coated GeS layer around the cladding of the FBG; (b) EDS image of the small inset portion (spectrum 1) of the FE-SEM image of GeS film coated along the fiber; (c) UV–visible absorption spectra of the GeS glass solution with PA as reference; (d) Raman spectra of the bulk GeS glass and GeS film coated on FBG sensor.
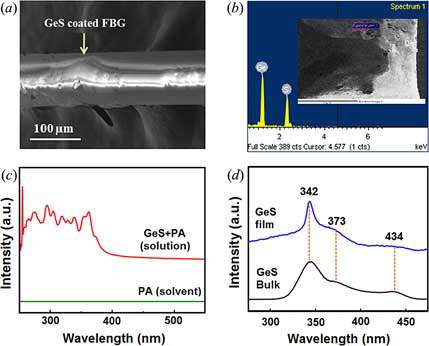
It can be seen from the optical absorption spectrum as shown in that, a strong absorption is observed in the near UV radiation by the GeS glass which is in agreement with the earlier results.[Citation19] shows the Raman spectrum of bulk GeS and GeS thin film which exhibits similar peak positions, in agreement with the earlier results.[Citation20] There is a narrowing observed for the peak at 342 cm−1 which can be attributed to solvent-induced structural changes.[Citation21] However, these modifications are found to have a less significant effect on the optical properties of the coated GeS film [].
The photoinduced strain (ε) in GeS causes a red shift in the λB of FBG sensor with both 254 and 302 nm light illumination. It is found that the shift in Bragg wavelength before and after UV irradiation is directly proportional to light absorbed by the GeS. The ε in GeS-coated FBG is related to the ΔλB by the following equation.[Citation18]
The ε measured by a GeS-coated FBG sensor in microstrain (με) corresponds to the sum of the photothermal strain in the substrate (silica fiber) (εt) in με as well as photostriction in GeS (εm) in με as shown in following equation.[Citation10]
To quantitatively estimate the photothermal strain in silica fiber, a bare FBG sensor (bare-FBG) is placed close to the GeS-coated FBG sensor. During the illumination experiments, bare FBG experiences photothermal strain which can be estimated using Equation (1).[Citation22] This enables the calculation the photostriction induced microstrain accurately.[Citation10]
In this experiment, UV radiation of wavelengths 302 and 254 nm have been used, which are band gap and above-band gap illumination, for analyzing the wavelength shift in GeS-coated FBG and bare-FBG including their corresponding optical reflection. shows the screenshots of the optical reflection spectrum of bare FBG and GeS-coated FBG for 302 and 254 nm illumination. For the GeS-coated FBG sensor, the Bragg wavelength is blue-shifted with respect to bare-FBG as shown in . Once the UV-C (254 nm) is illuminated, the Bragg wavelengths (λB) of both GeS-coated FBG and bare FBG sensors are increased as shown in . However, the shift in Bragg wavelength for GeS-coated FBG is higher than that of bare FBG due to additional strain caused by the photostriction in GeS. A similar effect is also observed in the case of UV-B (302 nm) illumination as shown in . When the UV sources are turned off, the Bragg wavelengths of GeS-coated FBG and bare-FBG recover almost to their initial values as shown in ).
Figure 3. Comparison of GeS-coated FBG (black line) and bare-FBG (violet line) reflection spectra (screenshots) obtained using a FBG interrogator. (a) Before any illumination, (b) on 254 nm light illumination, (c) on 302 nm light illumination, and (d) after cessation of the excitation light.
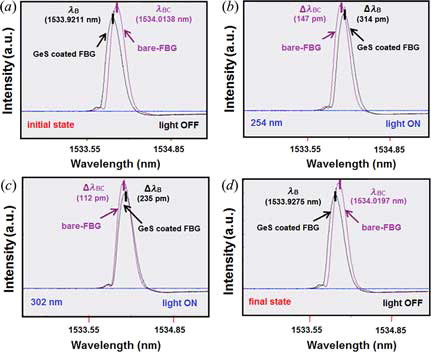
and shows the reversible ON and OFF cyclic responses of GeS-coated FBG and bare-FBG upon exposure to 254 and 302 nm light, respectively. Upon exposure to UV illumination, the Bragg wavelength increases gradually with time and gets saturated (∼40 min); after the UV lights are turned off, the Bragg wavelength gradually decreases with time and reaches almost its initial values (∼30 min). The GeS-coated FBG and bare-FBG both exhibit similar switching responses for 254 and 302 nm radiations. However, the shift in Bragg wavelength of GeS-coated FBG is much higher than that of bare-FBG without GeS. For 254 and 302 nm excitation, the GeS-coated FBG sensor shows a maximum ΔλB of ∼314 and ∼235 pm, respectively, which is ∼2 times higher than that of bare-FBG as shown in and . This indicates the effect of photostriction induced strain in GeS of the GeS-coated FBG sensor, after it is exposed to UV radiations.
Figure 4. Reversible change in Bragg wavelength for four consecutive cycles with respect to time in GeS-coated FBG and bare-FBG under illumination with (a) 254 nm, (b) 302 nm, (c) the Bragg wavelength shift in GeS material after eliminating the effect of temperature as a function of wavelength (254, 302 nm), and (d) the variation of photostriction with time in GeS-coated FBG with different light illumination (254, 302 nm).
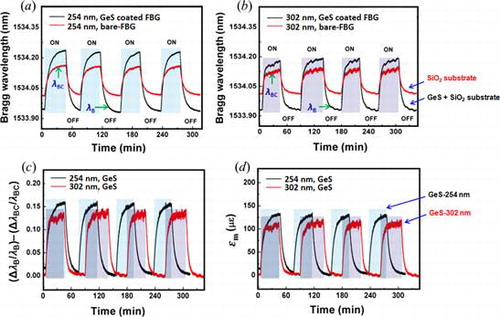
The effect of bare-FBG has been normalized and subtracted from the response of the GeS-coated FBG sensor; this gives the actual strain response of GeS material as shown in . In addition, shows the change in εm with respect to time in GeS material upon irradiation with 254 and 302 nm which is determined from the shift in the Bragg wavelength by Equation (4). The obtained results for the coated GeS film upon irradiation with 254 and 302 nm have been presented in . The photostriction induced in GeS film by 254 nm light is greater than that induced by 302 nm light which is in agreement with our absorption studies shown in the and .
Table 1. Summary of experimental results obtained from the photomechanical studies on the coated GeS film under UV lamps.
In the present study, UV lamps have been used to study the photothermodynamic structural changes in the GeS films. The response time of the UV lamp is slow (12–20 min) due to low power excitation. This further motivates to perform experiments to study the effect of higher wavelength (405 nm laser light) excitation with higher powers on the rise and fall times of the GeS-coated FBG sensor.
shows the ON and OFF states of Bragg wavelength shift and ε developed in GeS-coated FBG sensor for variable power densities using 405 nm light. The GeS-coated FBG sensor is found to respond linearly with an increase in power density of the excitation as seen in . A maximum of < 2°C rise (∼16 pm Bragg wavelength shift) has been observed in the case of bare-FBG upon exposure to 405 nm laser with a maximum power density of 1.34 W cm−2. Also, the bare-FBG response to 405 nm light actuation is relatively smaller (10 times) than GeS-coated FBG as shown in . Therefore, the ΔλBC/λBC term can be neglected in Equation (4). Hence, the photoinduced strain (ε) response on 405 nm laser irradiation mostly corresponds to the photo induced changes in GeS film.
Figure 5. (a) The reversible Bragg wavelength change and photoinduced strain changes obtained using 405 nm laser excitation with different powers in GeS-coated FBG sensor; (b) comparison of shift in Bragg wavelength with different 405 nm laser power for GeS-coated FBG and bare-FBG sensor; (c) rise time and (d) fall time obtained for GeS-coated FBG sensor with 405 nm light excitation.
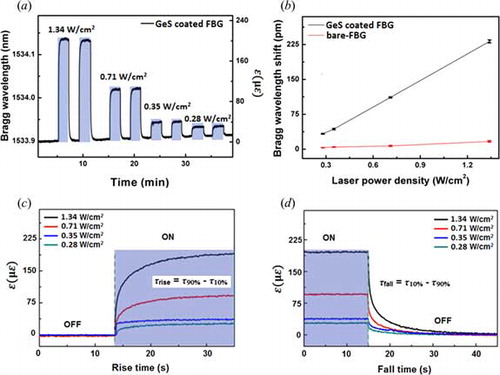
In addition, the rise (τrise = τ90% − τ10%) and fall (τfall = τ10% − τ90%) times for GeS-coated FBG sensor under 405 nm illumination, are determined for different powers as shown in and ; where τ90%, and τ10% are the time taken to create the 90 and 10% of photoinduced strain (ε) using illumination in the case of GeS-coated FBG sensor. The rise and fall times of GeS-coated FBG sensor for photoinduced actuation are determined for different laser power densities (0.28–1.34 W cm−2), from the . The summarizes the obtained photostriction [using Equation (4)], rise and fall time for GeS film on exposure to 405 nm laser with different laser power densities. The response and recovery time for 405 nm laser light illumination are of the order of seconds. Upon excitation with 405 nm light at a power density of 1.34 and 0.71 W cm−2, the τrise is found to be greater than the τfall. The τrise is found to be lesser than the τfall when excited at power densities of 0.35 and 0.28 W cm−2, as shown in and .
Table 2. Summary of experimental results obtained from the photomechanical studies on the coated GeS film under 405 nm laser.
Although the exact mechanism of photostriction observed in GeS is not implicit, an interplay between transient photodarkening (red shift of the optical absorption edge by illumination) and photoinduced volume expansion has been suggested to cause the photostriction effect.[Citation6,Citation17,Citation23Citation24Citation25Citation26] The photodarkening phenomenon mainly occurs in GeS film due to increase in the number of wrong bonds such as S-S [434 nm peak in Raman spectra shown in ].[Citation2,Citation4] And the characteristic behavior (i.e., τrise > τfall) observed in GeS could be correlated with the temporal influence of the ambient oxygen pressure on the surface of the coated GeS film during illumination.[Citation4,Citation17]
4. Summary
To conclude, a new method has been demonstrated for quantitative estimation of photostrictive actuation in the GeS film using FBG sensors. Above band-gap (254, 302 nm) and near band-gap (405 nm) illumination is used to study the phenomenon. The GeS exhibits a linear photostrictive behavior with an increase in laser power density of 405 nm laser. A maximum photostriction in the GeS material of the order of ∼10−4 is obtained for 405 nm (1.34 W cm−2) light illumination with a response time of ∼10 s. It is also evident from the present study that the photoresponsivity in visible and UV region of FBG sensor can be enhanced by coating a ChG over layer. Although the solvent-casting method allows the deposition of ChG thin film directly on an optical fiber, there are limitations on how well the material will be uniformly coated around the fiber. With this study as a base, further studies on thickness dependence and different ways of coating the ChG thin films uniformly around the fiber using solvent casting technique will be explored in future. Also future work in this direction can explore the applications of GeS-coated FBG for optical actuation/sensing applications such as radiation detection, chemical sensing, biosensor etc.[Citation9,Citation27,Citation28]
References
- Kuzukawa, Y.; Ganjoo, A.; Shimakawa, K. Photoinduced structural changes in obliquely deposited As-and Ge-based amorphous chalcogenides: Correlation between changes in thickness and band gap. J. Non-Cryst. Solids 1998, 227, 715–718.
- Ding, L.; Ren, J.; Jain, H.; Zhao, D.; Yang, G.; Chen, G. Self‐reversible photodarkening of the mixed GeS2–SbSI glasses. J. Am. Ceram. Soc. 2011, 94(6), 1657–1660.
- Knotek, P.; Tichy, L.; Arsova, D.; Ivanova, Z.G.; Ticha, H. Irreversible photobleaching, photorefraction and photoexpansion in GeS2 amorphous film. Mater. Chem. Phys. 2010, 119, 315–318.
- Messaddeq, S.H.; Mastelaro, V.R.; Li, M.S.; Tabackniks, M.; Lezal, D.; Ramos, A.; Messaddeq, Y. The influence of oxygen in the photoexpansion of GaGeS glasses. Appl. Surf. Sci. 2003, 205, 143–150.
- Igo, T.; Noguchi, Y.; Naga, H. Photoexpansion and thermal contraction of amorphous chalcogenide glasses. Appl. Phys. Lett. 1974, 25, 193–194.
- Kundys, B. Photostrictive materials. Appl. Phys. Rev. 2015, 2, 011301.
- Krecmer, P.; Moulin, A.M.; Stephenson, R.J.; Rayment, T.; Welland, M.E.; Elliott, S.R. Reversible nanocontraction and dilation in a solid induced by polarized light. Science 1997, 277, 1799–1802.
- Stuchlik, M.; Elliott, S.R. All optical actuation of amorphous chalcogenide-coated cantilevers. J. Non-Cryst. Solids 2007, 353, 250–262.
- Shivananju, B.N.; Suri, A.; Asokan, S.; Misra, A. Carbon nanotube coated fiber Bragg grating for photomechanical optic modulator. Rev. Sci. Instrum. 2013, 84, 095101.
- Kreuzer, M. Strain Measurement with Fiber Bragg Gratings Sensor. HBM GmbH, Darmstadt, Germany, 2013.
- Hill, K.O.; Fujii, Y.; Johnson, D.C.; Kawasaki, B. Photosensitivity in optical fiber waveguides: Application to reflection filter fabrication. Appl. Phys. Lett. 1978, 32, 647–649.
- Hill, K.O.; Malo, B.; Bilodeau, F.; Johnson, D.; Albert, J. Bragg gratings fabricated in monomode photosensitive optical fiber by UV exposure through a phase mask. Appl. Phys. Lett. 1993, 62, 1035–1037.
- Song, S.; Howard, S.S.; Liu, Z.; Dirisu, A.O.; Gmachl, C.F.; Arnold, C.B. Mode tuning of quantum cascade lasers through optical signal processing of chalcogenide glass claddings. Appl. Phys. Lett. 2006, 89, 041115.
- Markos, C.; Yannopoulos, S.N.; Vlachos, K. Chalcogenide glass layers in silica photonic crystal fibers. Opt. Express 2012, 20, 14814–14824.
- Hughes, M.; Yang, W.; Hewak, D. Fabrication and characterization of femtosecond laser written waveguides in chalcogenide glass. Appl. Phys. Lett. 2007, 90, 131113.
- Sivakumar, G.; Sabapathy, T.; Ayiriveetil, A.; Kar, A.K.; Asokan, S. Photo-thermal modifications in ultrafast laser inscribed chalcogenide glass waveguides. Proc. SPIE 2013, 8769, 87692L-1–5.
- Popescu, M.; Sava, F.; Lorinczi, A.; Iovu, M.S.; Shutov, S.; Hoyer, W.; Bradaczek, H. The effect of ultraviolet radiation on the structure of non-crystalline chalcogenides. J. Optoelectron. Adv. Mat. 2000, 2, 667–670.
- Othonos, A. Fiber Bragg gratings. Rev. Sci. Instrum. 1997, 68, 4309–4341.
- Huang, C.C.; Hewak, D.W. Deposition and characterization of germanium sulphide glass planar waveguides. Opt. Express 2004, 12, 2501–2506.
- Slang, S.; Janicek, P.; Palka, K.; Vlcek, M. Structure and properties of spin-coated Ge25S75 chalcogenide thin films. Opt. Mat. Express 2016, 6, 1973–1985.
- Waldmann, M.; Musgraves, J.D.; Richardson, K.; Arnold, C.B. Properties of solution processed Ge23Sb7S70. J. Mater. Chem. 2012, 22, 17848–17852.
- Kim, H.K.; Shin, W.; Ahn, T.J. UV sensor based on photomechanically functional polymer-coated FBG. IEEE Photon. Technol. Lett. 2010, 22, 1404–1406.
- Shimakawa, K.; Kolobov, A.; Elliott, S.R. Photoinduced effects and metastability in amorphous semiconductors and insulators. Adv. Phys. 1995, 44, 475–588.
- Gayathri, S.; Varma, G.S.; Asokan, S. The role of photo-striction in tailoring the nano-scale phase changes in amorphous selenium thin films. MRS Adv. 2016, 1(39), 2743–2748.
- Mishchenko, A.; Lindberg, G.P.; Weinstein, B.A.; Reznik, A. Comparative study of the photodarkening relaxation-kinetics in amorphous selenium for above-bandgap and sub-bandgap illumination. Appl. Phys. Lett. 2014, 105, 051912.
- Wen, S.C.; Chang, C.W.; Lin, C.M.; Liu, H.; Hsiao, V.K.S.; Yu, J.; Chen, Z. Light-induced switching of a chalcogenide-coated side-polished fiber device. Opt. Commun. 2015, 334, 110–114.
- Sridevi, S.; Vasu, K.S.; Asokan, S.; Sood, A.K. Sensitive detection of C-reactive protein using optical fiber Bragg gratings. Biosens. Bioelectron. 2015, 65, 251–256.
- Sridevi, S.; Vasu, K.S.; Bhat, N.; Asokan, S.; Sood, A.K. Ultra sensitive NO2 gas detection using the reduced graphene oxide coated etched fiber Bragg gratings. Sens. Act. B Chem. 2016, 223, 481–486.