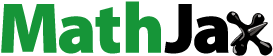
Abstract
Objectives
Social dysfunction is one of the most common signs of major neuropsychiatric disorders. The Default Mode Network (DMN) is crucially implicated in both psychopathology and social dysfunction, although the transdiagnostic properties of social dysfunction remains unknown. As part of the pan-European PRISM (Psychiatric Ratings using Intermediate Stratified Markers) project, we explored cross-disorder impact of social dysfunction on DMN connectivity.
Methods
We studied DMN intrinsic functional connectivity in relation to social dysfunction by applying Independent Component Analysis and Dual Regression on resting-state fMRI data, among schizophrenia (SZ; N = 48), Alzheimer disease (AD; N = 47) patients and healthy controls (HC; N = 55). Social dysfunction was operationalised via the Social Functioning Scale (SFS) and De Jong-Gierveld Loneliness Scale (LON).
Results
Both SFS and LON were independently associated with diminished DMN connectional integrity within rostromedial prefrontal DMN subterritories (pcorrected range = 0.02–0.04). The combined effect of these indicators (Mean.SFS + LON) on diminished DMN connectivity was even more pronounced (both spatially and statistically), independent of diagnostic status, and not confounded by key clinical or sociodemographic effects, comprising large sections of rostromedial and dorsomedial prefrontal cortex (pcorrected=0.01).
Conclusions
These findings pinpoint DMN connectional alterations as putative transdiagnostic endophenotypes for social dysfunction and could aid personalised care initiatives grounded in social behaviour.
Introduction
Adaptive social functioning is critical to human survival and heavily dependent on complex neurocognitive systems unique to human beings (Holt-Lunstad et al. Citation2010; Porcelli et al. Citation2019; van der Wee et al. Citation2019). Social dysfunction is accordingly one of the first and most common signs of major neuropsychiatric disorders. This is likely because of the enormous amount and complexity of brain network processes required to initiate and maintain adaptive social behaviour (Porcelli et al. Citation2019; van der Wee et al. Citation2019). Stimulated by clinical observations, converging lines of research report shared negative symptomatology such as social dysfunction across multiple neuropsychiatric disorders (Winograd-Gurvich et al. Citation2006; Insel et al. Citation2010; Porcelli et al. Citation2019; Ike et al. Citation2020). This advocates for a neurobiological correlate at the basis of social dysfunction that is possibly distinct and partly independent of the current neuropsychiatric nosologies (Buckholtz and Meyer-Lindenberg Citation2012; Porcelli et al. Citation2019). Empirical data in support of this notion, however, are lacking and this tends to preclude a thorough understanding of social dysfunction as a transdiagnostic phenotype rather than a symptom of psychiatric nosologies (Kas et al. Citation2007; Insel et al. Citation2010). To this end, the pan-European PRISM (Psychiatric Ratings using Intermediate Stratified Markers) project examined the cross-disorder value of social dysfunction and its putatively distinct neurobiological correlates in two distinctive disorders, Schizophrenia (SZ) and Alzheimer’s Disease (AD) (Kas et al. Citation2019). Whereas these two disorders differ in core symptoms, genetic profile, and underlying neurobiology, they importantly overlap considerably in social deficits (i.e. social withdrawal, interpersonal dysfunction, loneliness) (Kas et al. Citation2019; Cuthbert Citation2019). This renders them therefore as ideal candidates to address the PRISM study objectives. Using distinctively different neuropsychiatric disorders mitigates confounding effects and allows identification of truly transdiagnostic effects/associations (Kas et al. Citation2019). This aligns nicely with the RDoC perspective that clinical psychological problems are best defined along functional domains with shared neurobiological substrates, regardless of diagnostic nosologies, to attain novel insights and advance treatment (Insel et al. Citation2010).
A neurobiological system potentially relevant to both social (dys)function and SZ/AD pathophysiology is the brain’s Default Mode Network (DMN), which crucially shapes various aspects of human social behaviour (Buckner et al. Citation2008; Mars et al. Citation2012; Mazza et al. Citation2013; Andrews-Hanna et al. Citation2014; Li et al. Citation2014; Padmanabhan et al. Citation2017; Badhwar et al. Citation2017; Hu et al. Citation2017; Pievani et al. Citation2017; Lefort-Besnard et al. Citation2018; Spreng et al. Citation2020). The complexity of DMN function is reflected by the broad scope of brain areas involved in the DMN (Buckner et al. Citation2008; Andrews-Hanna et al. Citation2010, Citation2014; Spreng and Andrews-Hanna Citation2015). The core DMN system mainly processes personally relevant, sociocognitive information, with the rostromedial prefrontal cortex (PFC) and posterior cingulate cortex being its key nodes (Buckner et al. Citation2008; Andrews-Hanna et al. Citation2010, Citation2014; Spreng and Andrews-Hanna Citation2015). The medial temporal section of DMN is associated with recollection of experiences and autobiographical processing and is comprised of the hippocampal formation, retrosplenial cortex, inferior parietal lobule, and ventromedial PFC (Buckner et al. Citation2008; Andrews-Hanna et al. Citation2010, Citation2014; Spreng and Andrews-Hanna Citation2015). The dorsomedial section of DMN, on the other hand, is predominantly involved in socially coloured, meta-cognitive processes and mentalizing (i.e. inferences about others’ internal state) and is anchored in the temporoparietal junction (TPJ), superior frontal gyrus (SFG) and dorsomedial PFC (Buckner et al. Citation2008; Andrews-Hanna et al. Citation2010, Citation2014; Spreng and Andrews-Hanna Citation2015). DMN sub-sections are highly intertwined and this allows for whole-network parallel functioning, which is a key ingredient to DMN modulation of complex human social behaviours (Spreng and Andrews-Hanna Citation2015). Crucially, the DMN largely overlaps with the so-called ‘social brain’, for which perturbations among SZ/AD patients have been suggested and linked to social impairment (Porcelli et al. Citation2019).
Alterations in DMN connectional integrity among SZ and AD patients have been described per disorder in several overview papers and linked to deficits in social, cognitive, and affective processes that the DMN seems to subserve (e.g. self-referential processing, mentalising, emotion recognition/resonance) (Mazza et al. Citation2013; Badhwar et al. Citation2017; Hu et al. Citation2017; Pievani et al. Citation2017; Lefort-Besnard et al. Citation2018; Porcelli et al. Citation2019; Li et al. Citation2019). The most consistent finding in both SZ and AD is altered functional connectivity patterns within and between cortical midline sections of the DMN (Whitfield-Gabrieli and Ford Citation2012; Mazza et al. Citation2013; Anticevic et al. Citation2015; Badhwar et al. Citation2017; Hu et al. Citation2017; Pievani et al. Citation2017; Lefort-Besnard et al. Citation2018; Li et al. Citation2019; Porcelli et al. Citation2019). Of note, DMN disturbances are also observed in other neuropsychiatric disorders characterised by severe social dysfunction, including autism, social phobia, and depression (Maresh et al. Citation2014; Kaiser et al. Citation2015; Mulders et al. Citation2015; Padmanabhan et al. Citation2017; Dixon et al. Citation2020; Saris et al. Citation2020), further corroborating the importance of DMN to both normal and disturbed social functioning. It is assumed that large-scale brain network dysregulations, particularly those in the DMN, may contribute to core deficits in social, general cognitive, and affective functions, which in turn, could trigger (pre)clinical symptomologies in neuropsychiatric disorders such as SZ and AD (Menon Citation2011). Hence, a brain network approach towards social dysfunction in these distinct disorders offers a powerful means to transdiagnostically investigate how dysfunctional brain architecture could interfere with adaptive social behaviour. Suboptimal DMN integrity has indeed been reported in relation to general social dysfunction as well as subjective feelings of loneliness (Cacioppo et al. Citation2014; Schmälzle et al. Citation2017). Most convincing piece of evidence in this regard was recently presented in the massive UK Biobank dataset (N∼38000), which robustly linked DMN dysconnectivity to subjective feelings of loneliness (Spreng et al. Citation2020).
Yet, no study has thus far directly examined the association between social dysfunction and DMN connectional integrity across SZ/AD patients and matched healthy controls. As such, we lack an integrated account of underlying mechanisms or a consensus regarding the framework of these relationships. The current study hence innovatively addressed this issue, by examining whether DMN connectivity is transdiagnostically coupled with social dysfunction, both its behavioural component and its subjective experience, as these are often observed in SZ/AD patients and tend to co-occur with DMN disintegrity (Porcelli et al. Citation2019; Cacioppo et al. Citation2014; Schmälzle et al. Citation2017). Diagnostic nosologies were accounted for in all analyses to identify truly transdiagnostic effects. Key clinical and demographic variables were corrected for to further aid robustness and specificity of findings. Importantly, all data collection (including MRI) and participant-level assessments were performed according to fully harmonised protocols across sites. We aimed to include patients with a relatively recent disease onset in order to capture as much as possible of the underlying neurobiology of social dysfunction rather than long-term consequences of psychopathology or neurodegeneration. Post hoc analyses additionally examined whether any brain–behaviour relationship documented here is specific/exclusive to the DMN. Based on prior work on DMN and social (dys)functioning, we hypothesised social dysfunction to transdiagnostically relate to diminished DMN connectional integrity, especially within its cortical midline sections (Mazza et al. Citation2013; Badhwar et al. Citation2017; Hu et al. Citation2017; Pievani et al. Citation2017; Lefort-Besnard et al. Citation2018; Porcelli et al. Citation2019; Li et al. Citation2019; Saris et al. Citation2020). We also anticipated these effects to be distinct and (partly) independent of that of diagnostic status (Porcelli et al. Citation2019).
Methods and materials
Participants
Data for the current study were derived from the PRISM study and included 48 SZ and 47 AD patients, along with 55 matched HC participants (28 HC younger (18–45 years) and 27 HC older (50–80 years)) (Kas et al. Citation2019; Bilderbeck et al. Citation2019). Participants were recruited between April 2017 and April 2019 from three sites in the Netherlands (University Medical Centre Utrecht, VU University Medical Centre, and Leiden University Medical Centre) and two sites in Spain (Hospital General Universitario Gregorio Marañón and Hospital Universitario de La Princesa). provides an overview of participant inclusion per site. Importantly, all data collection and participant-level assessments were performed according to fully harmonised protocols across sites. All participants provided verbal and written informed consent prior to participation and were considered as sufficiently competent to participate by researchers and caregivers.
Table 1. Baseline characteristics (N = 150).
Clinical assessment
DSM-IV diagnosis of SZ was confirmed using the Mini-International Neuropsychiatric Interview (M.I.N.I.-screen), with at least one psychotic episode and maximum of 15-year disease duration since diagnosis (Sheehan et al. Citation1998). SZ patients had to be on stable antipsychotic/anticholinergic/antidepressant medication dosage for at least eight weeks, and 18–45 years of age. SZ patients were excluded if they scored 22 on the seven-item positive symptoms subscale of the PANSS, to rule out an active psychotic episode possibly hampering adequate study participation (Kay et al. Citation1987; Bilderbeck et al. Citation2019). Diagnosis of probable AD was established according to the National Institute on Ageing and the Alzheimer’s Association criteria (McKhann et al. Citation2011). AD patients had to additionally score 20–26 (i.e. mild AD pathology) on the mini-mental state examination (MMSE), and be 50–80 years of age (Folstein et al. Citation1975). AD patients with history of strokes, either based on clinical judgement, medical history or imaging results, were excluded. As the patient groups differed significantly in age, we also included two age-matched healthy control groups in the study to mitigate age effects, while additionally correcting for age and age-squared in all analyses. HC participants exclusion criteria were any history of psychopathologies (as confirmed by the MINI) or neurological disorders, and usage of psychotropics and central nervous system affecting medication. More detail regarding participant in- and exclusion criteria is provided in the Supplement. Cognitive dysfunction was estimated in AD patients using the Alzheimer’s Disease Assessment Scale – Cognitive subscale (ADAS-cog) (Rosen et al. Citation1984). Current states of positive and negative symptoms of schizophrenia were measured using the PANSS (positive and negative syndrome scale) (Kay et al. Citation1987).
Indicators of social dysfunction
The current study examined both the behavioural component and subjective experience of social dysfunction (Maresh et al. Citation2014; Kaiser et al. Citation2015). The behavioural aspect of social dysfunction was indexed with the Social Functioning Scale (SFS) (Birchwood et al. Citation1990). The SFS multidimensionally examines social functioning with seven subscales: social withdrawal, interpersonal functioning, prosocial activities, recreational activities, competence and performance independence and employment. The subscale ‘employment’ was not used for total scale analyses, since most participants were retired in the older HC and AD group introducing a bias in line with reporting in a previous study (Vázquez Morejón et al. Citation2000). All subscales had different raw maximum scores ranging from 18–39. Questions were largely quantitative in nature such as: ‘how often do you go to…’. We reverse scored the individual subscales so that a higher score would indicate more social dysfunction. The total score on the SFS, following the original SFS scoring guidelines with provided conversion table, was used for the final imaging analyses (Birchwood et al. Citation1990). The Jong-Gierveld Loneliness (LON) questionnaire was employed to assess the subjective experience of social dysfunction in the form of feelings of loneliness, with higher total scores indicating more loneliness, with questions such as: ‘do you have someone in whom you can confide’ (de Jong-Gierveld et al. Citation1985). The LON questionnaire consists of 11 items scored on a 3-point Likert scale, with a maximum score of 33. The two questionnaires were moderately correlated (Spearman’s r = 0.54, p < 0.001), suggesting that while having some overlap they capture partly different aspects of social dysfunction. We also examined the average cumulative effect of the behavioural aspect and subjective experience of social dysfunction (Mean.SFS + LON) on DMN connectional integrity.
MRI data acquisition and pre-processing
Resting-state fMRI and structural MRI data were acquired according to fully harmonised protocols across sites (see Supplementary data). Philips Achieva 3 T MRI scanners were used at the Dutch sites, while a Siemens Prisma 3 T MRI scanner was used at the Spanish site. All MRI assessments for both Spanish recruiting sites were performed on a single MRI scanner. An extensive quality assurance protocol was implemented to mitigate any scanner-specific effects, which included harmonised scanning protocols, pilot testing with a ‘traveling head’, and correction for site in all statistical analyses (see Supplementary data for details). All data were subsequently pre-processed and cleaned according to established protocols and current standards, using FSL software (V6.0; http://fsl.fmrib.ox.ac.uk/fsl/fslwiki/, see also Supplementary data). Additional (micro)motion-related artefact removal (ICA-AROMA), and white matter/cerebrospinal fluid signal removal was implemented to further eliminate noise.
DMN functional connectivity and social dysfunction
depicts the analytical pipeline employed in this study, with detailed description provided in the Supplement. In short, probabilistic Independent Component Analysis (ICA) within FSL’s MELODIC module was used for data-driven decomposition of the entire pre-processed RS-fMRI dataset into 20 temporally and spatially independent components (i.e. intrinsic functional brain networks) (Nickerson et al. Citation2017). This level of granularity is typically used to identify large-scale, canonical brain networks (Smith et al. Citation2009; Laird et al. Citation2011). The group-average set of components (i.e. resting state networks) generated by MELODIC was then used to generate subject-specific versions of them (spatial maps and associated time courses) via FSL’s Dual Regression tool (Nickerson et al. Citation2017). The DMN was identified based on its distinct topological architecture, as described in seminal overview papers and subjected to further analysis (; Smith et al. Citation2009; Laird et al. Citation2011).
Figure 1. Functional connectivity analyses of the Default Mode Network (DMN). Collected resting-state fMRI data were first preprocessed and cleaned. Data from all participants was concatenated across time and submitted to a probabilistic group independent component analysis (ICA) using MELODIC. The group ICA produced a set of 20 independent spatial maps/components (i.e. functional networks). The set of spatial maps generated by MELODIC was then used to generate subject-specific versions of these spatial maps, and associated time courses, using Dual Regression. That is, for each subject, the group-average set of spatial maps was regressed (as spatial regressors in multiple regression) onto the subject’s 4 D space-time dataset. This resulted in a set of subject-specific time series, one per group-level spatial map. Next, these time series were regressed (as temporal regressors, again using multiple regression) against the same 4 D dataset, resulting in a set of subject-specific spatial maps, one per group-level spatial map. Our component of interest (i.e. DMN) was then selected based on spatial similarity to functional networks described in prior seminal papers on DMN connectivity and architecture. Finally, permutation testing (N = 5000) was used to examine the association between DMN connectivity and social dysfunction proxies, while correcting for key clinical and sociodemographic factors. Results were adjusted for multiple comparisons using Threshold-Free Cluster Enhancement with Family-Wise Error correction at p < 0.05. Adapted and reprinted with permission from Wiley Periodicals, Inc.: Human Brain Mapping (Smith et al. Citation2015).
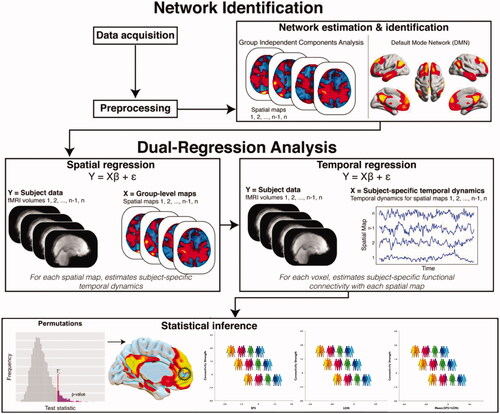
We explored the transdiagnostic effects of social dysfunction on DMN whole-network connectivity, using non-parametric, permutation-based General Linear Model (GLM) analyses with FSL’s Randomise tool (5000 permutations). The GLM included the individual participants’ SFS (i.e. behavioural social dysfunction) and LON (i.e. subjective experience social dysfunction) total scores as separate regressors, wherein both the unique and the average cumulative effects of these two constructs on DMN connectional integrity were examined. This dimensional analysis tested whether across the sample any linear associations can be found between DMN connectivity and individual participant’s SFS and/or LON scores. Diagnostic status (i.e. SZ/AD/HC-younger/HC-older) was also entered in the GLM as regressor, in order to correct for it and disentangle its impact on DMN from that of social dysfunction. Key clinical (psychotropic medication, comorbid symptomology) and sociodemographic (age, age squared, sex, education, scan site/type) factors were corrected for in the analyses to aid robustness and reliability of findings (added as regressors in GLM). All variables were demeaned across groups, with statistical thresholding and correction for multiple comparisons achieved through Threshold-Free Cluster Enhancement (TFCE) with family-wise error (FWE) correction at p < 0.05 (Smith and Nichols Citation2009).
Results
Sample characteristics
The sample characteristics are listed in . As expected, a large majority of SZ patients used antipsychotic medication (89.4%), with some also using antidepressants (20.8%). Nearly half of the AD patients used AD-specific medication (e.g. acetylcholinesterase inhibitor and/or NDMA receptor antagonist) (44.7%). Mean positive and negative symptoms in SZ patients was 11.0 and 14.9, respectively, as measured with the PANSS (indicating mild pathology, matching stable outpatient clinic characteristics). Mean dementia symptomatology was 26.8 on the ADAS-cog for the AD group (indicating mild dementia symptomatology). Compared to age-matched controls, patients had in general more depressive symptomatology and less positive affect, though none of the groups reached clinical depression levels, as participants with clinical depression were excluded before the analyses. The SFS and LON total scores differed between patient groups and their age-matched HC participants (p’s <0.001), except for LON scores between the AD patients and older healthy controls (p = 0.19). The patient groups (AD and SZ patients) differed significantly in their SFS, LON and mean SFS + LON scores (p < 0.001).
DMN connectivity and social dysfunction
The analyses revealed a transdiagnostic association between behavioural aspects of social dysfunction (SFS) and diminished DMN connectional integrity, specifically within the rostromedial PFC subterritory of the DMN (TFCE & FWE corrected, p = 0.02) (). A similar link was found between participants’ subjective experience of social dysfunction (LON) and diminished DMN connectional integrity in the rostromedial PFC (TFCE & FWE corrected, p = 0.04) (). The mean cumulative effect of SFS and LON on diminished DMN connectivity was even more pronounced (both spatially and statistically), comprising large sections of the rostromedial and dorsomedial PFC (p = 0.01) (). Post hoc analyses additionally revealed that above-mentioned effects were not moderated (interaction) by diagnostic status (p’s > 0.05), thus further corroborating their transdiagnostic nature.
Figure 2. Social dysfunction transdiagnostically associated with DMN connectivity. The upper panel (A) depicts medial views of the DMN (yellow-orange), with the effects site (rmPFC; blue) for the transdiagnostic negative association between DMN connectivity and the SFS superimposed on it (TFCE & FWE corrected, p = 0.02). The middle panel (B) depicts medial views of the DMN (yellow-orange), with the effects site (rmPFC; blue) for the transdiagnostic negative association between DMN connectivity and LON superimposed on it (TFCE & FWE corrected, P = 0.04). The lower panel (C) depicts medial views of the DMN (yellow-orange), with the effects site (rmPFC & dmPFC; blue) for the transdiagnostic negative association between DMN connectivity and Mean SFS + LON superimposed on it (TFCE & FWE corrected, p = 0.01). The last panel (D) depicts the DMN constellation as generated per our resting-state fMRI pipeline. The yellow-orange scalar bar represents connectivity strengths (Z-value) within DMN, while the blue scalar bar reflects significance level (P-value) of social dysfunction-DMN associations. The scatter plots (A, B, C) provide a quantitative visualisation of this effect, wherein mean connectivity estimates from the DMN effect sites (y axis) are plotted against social dysfunction scores (x axis). The values on y and x axis are Z-score residuals. The black solid line depicts the slope of the association, with the dotted bands indicating the 95% confidence interval of the slope. Higher positive values on the x axis indicate more severe social dysfunction. LON: de Jong-Gierveld Loneliness questionnaire; SFS: Social Functioning Scale
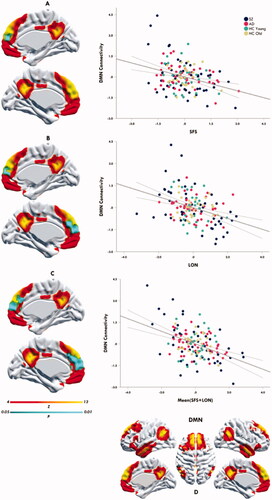
On top of our transdiagnostic analyses of social dysfunction–DMN relationships, our GLM model simultaneously also investigated the impact of diagnostic status on DMN connectivity, so to fully appreciate the unique contributions of social dysfunction to DMN integrity. Of note, these analyses thus mainly serve as sensitivity tests, and were not set up to examine the DMN-correlates of SZ/AD diagnostic status (already described extensively) (Badhwar et al. Citation2017; Hu et al. Citation2017). The analyses showed that SZ patients exhibited abnormally increased DMN connectivity within rostromedial PFC and temporoparietal territories, in comparisons to their age-matched healthy control peers (TFCE & FWE corrected, p = 0.01) (see Figure S1 in Supplementary data). No differences were found in DMN connectivity between the AD patients and older HC participants. These disorder-specific findings (or their lack of) clearly diverge from those of social dysfunction, being spatially different and directionally distinctive (increased vs. decreased connectivity), further corroborating the specificity of the social dysfunction-DMN relations we documented.
Network-specificity analyses
Post hoc analyses assessed whether brain-social behaviour relationships documented here are specific to the DMN. We therefore reran our dimensional brain-social dysfunction analyses, though now focussing on two other canonical brain networks often implicated in neuropsychiatric disorders: The Salience Network (SN; serves saliency mapping) and the Central Executive Network (CEN; governs executive functions & behavioural control). The influential triple network model of psychopathology posits that functional disorganisation within the DMN and these two networks collectively spur susceptibility for maladaptive social behaviour and mental disorders, including SZ and AD (Menon Citation2011). The SN and CEN were part of our 20 network MELODIC-ICA solution (see Methods), which automatically split up the CEN into a right- and left-lateralized network assembly. GLM modelling and analytical sequence proceeded exactly as described for the DMN-social dysfunction analyses, though statistical inferences were additionally Bonferroni corrected for N networks to control for multiple testing (TFCE & FWE; P 0.05/3 = 0.017). No significant link between social dysfunction and SN or CEN connectivity emerged though (P’s > 0.05), indicating that the brain-behaviour relationships reported here are specific to DMN.
Discussion
The current study examined how behavioural aspects and subjective experiences of social dysfunction transdiagnostically associate with DMN connectional integrity, among SZ/AD patients and age-matched healthy controls. We found that the behavioural aspect and subjective experience of social dysfunction are both transdiagnostically linked to decreased prefrontal DMN connectivity, with their cumulative effect being even more pronounced (both spatially and statistically). These effects were independent of diagnostic status, not confounded by key clinical (psychotropic medication, comorbid symptomology) and sociodemographic (age, sex, education, scan location) factors, and highly specific to the DMN. These findings pinpoint DMN connectional alterations as putative transdiagnostic endophenotypes for social dysfunction, and could plausibly aid personalised care initiatives grounded in social behaviour.
DMN connectivity and social dysfunction
Our analyses revealed that the behavioural aspect and subjective experience of social dysfunction are both uniquely and transdiagnostically associated with decreased DMN connectivity across SZ/AD/HC participants. The main effect site for this negative association comprised the rmPFC subsection of the DMN, and to a lesser extent its dmPFC subterritory. Findings largely echo the growing body of literature emphasising that the DMN is central to adaptive social functioning (Buckner et al. Citation2008; Schilbach et al. Citation2008; Andrews-Hanna Citation2012; Che et al. Citation2014; Spreng and Andrews-Hanna Citation2015). Social dysfunction has recently been associated with decreased connectivity in the rmPFC as part of the DMN in various neuropsychiatric disorders (Padmanabhan et al. Citation2017; Fox et al. Citation2017; Saris et al. Citation2020), though our study is the first to showcase this in a transdiagnostic sample. Although in close topological proximity, the rmPFC and dmPFC have differential functions within the DMN (Li et al. Citation2014; Andrews-Hanna et al. Citation2014; Dixon et al. Citation2017). The rmPFC is a key node of the core DMN system in addition to the PCC, (Andrews-Hanna et al. Citation2014; Buckner et al. Citation2008; Spreng and Andrews-Hanna Citation2015) and as such actively involved in coupling of the DMN subsystems. The rmPFC mainly functions within the core DMN system to support self-referential thoughts, self-other dichotomies, and socioemotional processing (Buckner et al. Citation2008; Andrews-Hanna et al. Citation2014; Spreng and Andrews-Hanna Citation2015; Dixon et al. Citation2017). The core DMN system is active when constructing future scenes based on autobiographical experiences, as well as when we are thinking of friends and individuals similar to ourselves (Benoit et al. Citation2010; Spreng and Andrews-Hanna Citation2015). Data on the rmPFC support the concept that self-referential processes are also employed for thinking about others (Benoit et al. Citation2010). The dmPFC on the other hand is part of the dorsomedial DMN (dmDMN) subsystem, which additionally comprises the TPJ and SFG (Andrews-Hanna et al. Citation2010; Benoit et al. Citation2010; Dixon et al. Citation2017). The dmDMN subsystem is predominantly active during higher order social processes such as self-reflective judgements, or when we are inferring upon the mental states of others (i.e. mentalizing) (Andrews-Hanna et al. Citation2014; Li et al. Citation2014; Spreng and Andrews-Hanna Citation2015).
In this study, more severe social dysfunction (mean cumulative effect of behavioural and affective social dysfunction) was associated with more widespread perturbations in the DMN, with diminished recruitment of the dmPFC (i.e. dmDMN subsystem node) in addition to the rmPFC (i.e. core DMN node). This supports the notion that the connectional disturbances in the core DMN could lead to dysconnectivity in the dmDMN subsystem as well (Menon Citation2011; Buckholtz and Meyer-Lindenberg Citation2012; Spreng and Andrews-Hanna Citation2015; Kaiser et al. Citation2015; Saris et al. Citation2020). However, it remains unclear if social dysfunction gives rise to DMN dysconnectivity or the other way around. The cross-sectional nature of this study does not allow for causal inferences, yet tentative empirical data tends to support the DMN dysconnectivity as the causal factor. Clinical examples include rTMS and psychotropic medication that manage to normalise DMN functional and connectional integrity, followed by altered (more favourable) social behaviour (Anderson et al. Citation2016; Pievani et al. Citation2017, Lorenzi et al. Citation2011; Goveas et al. Citation2011; Bais et al. Citation2017). Moreover, animal data describing stimulation/manipulation of key DMN subregions/circuits, moreover, show stark changes in social behaviour (Shemesh et al. Citation2016; Filiano et al. Citation2016; Mills et al. Citation2016; Zott et al. Citation2018; Missault et al. Citation2019; Ike et al. Citation2020). Additionally, top hits from a recently performed GWAS of the sociability trait were most heavily expressed in frontal DMN regions/circuits, with sociability and loneliness moreover being genetically linked (Bralten et al. Citation2019). Thus, irrespective of the origin of the perturbed functional connectivity within the DMN, disturbances in this crucial brain network appear to give rise to a wide variety of core deficits in sociocognitive and socioaffective functioning, which are likely to trigger (sub)clinical symptomatalogies (Kaiser et al. Citation2015).
Our post hoc analyses did not find any significant links between social dysfunction and SN or CEN connectivity (P’s > 0.05, Bonferroni corrected), which was performed to probe the specificity of DMN disintegrity to social dysfunction. This is somewhat in contrast to current literature on large-scale networks and human behaviour or neuropsychiatry (Menon Citation2011). The triple network model of psychopathology, for instance, postulates that an imbalance within or between large-scale brain networks could derail key cognitive and emotional processes. Specifically, the SN mainly seems to serve as a crucial switch from the internal oriented DMN with the more externally oriented CEN (Menon Citation2011; Uddin Citation2015; Seeley Citation2019). Whereas the DMN is directly linked to self-related and social processes, the SN seems to differentiate between internal and extra personal stimuli in order to guide (social) behaviour. While the roles of the SN and CEN are notable in adaptive behavioural processes, findings from current and prior research indicate that the DMN is highly specific for social dysfunction. This is most convincingly illustrated in the massive UK Biobank study (N∼38000) where there was a link between impaired social behaviour and the DMN, but no other brain network (Spreng et al. Citation2020).
DMN connectivity and diagnostics
Though this study was not designed to replicate dysfunction previously well described changes in the DMN as a function of SZ or AD diagnosis, we did include categorical diagnostics in the model to pinpoint the unique transdiagnostic effect of social dysfunction on the DMN. This mainly served as a sensitivity test, and was not set up to examine the DMN-correlates of SZ/AD diagnostic status (described extensively elsewhere (Badhwar et al. Citation2017; Hu et al. Citation2017). We found disorder-specific increased connectivity in the DMN among SZ patients relative to their HC peers, mainly in the TPJ and rostromedial PFC. Although inconsistencies exist in the field showing both hypo- and hyper-connectivity of the DMN in SZ patients, most evidence points towards hyperconnectivity of the DMN (Whitfield-Gabrieli and Ford Citation2012; Mazza et al. Citation2013; Anticevic et al. Citation2015; Hu et al. Citation2017; Lefort-Besnard et al. Citation2018; Li et al. Citation2019; Doucet et al. Citation2020). The TPJ, for example, seems to govern the balance between external and internal stimuli, between sensory information and social cognition or the mirror system (Schilbach et al. Citation2008; Mars et al. Citation2012; Schilbach et al. Citation2012; Bzdok et al. Citation2013; Amft et al. Citation2015; Lefort-Besnard et al. Citation2018). An imbalance in this area might thus lead to altered integration of context-dependent information (Bzdok et al. Citation2013).
Commonly described disruptions of the DMN within AD patients are located in the medial temporal lobe and posterior cingulate cortex/precuneus, spreading to the lateral parietal and medial frontal regions of the DMN as the disease progresses (Pievani et al. Citation2011; Mohan et al. Citation2016). In contrast to these earlier findings, we found no dysconnectivity of the DMN in AD patients relative to their age-matched healthy controls. To reiterate, however, this study was not designed to replicate previously implicated changes in the DMN as a function of AD or SZ diagnosis. We only included categorical diagnostics in the model to pinpoint the unique transdiagnostic effects of social dysfunction on the DMN (over and above diagnostics), thus simply serving as a sensitivity test rather AD diagnostic correlates. Moreover, our relatively small and mildly impaired AD sample, along with the social dysfunction-oriented GLM models may have further contributed to this null finding.
The disorder-specific findings (or their lack of) clearly diverge from those of social dysfunction, being spatially different and directionally distinctive (increased vs. decreased connectivity). In short, while patient-status may affect DMN connectivity, it importantly does not account for the above-mentioned findings on social dysfunction-DMN coupling. In fact, our findings robustly showcase that DMN connectional changes are a neurobiological correlate of social dysfunction that is distinct and independent of current neuropsychiatric nosologies. Findings thus support the ongoing paradigm shift from the traditional nosological perspective on neuropsychiatry towards a more transdiagnostic approach of key functional domains and their neurobiobehavioral underpinnings, such as social (dys)function (Insel et al. Citation2010; Buckholtz and Meyer-Lindenberg Citation2012).
Strengths and limitations
Our study has a number of limitations that need to be acknowledged. For example, the cross-sectional nature of this study does not allow for causal inferences. Ideally, longitudinal studies with additional neuropsychiatric disorders (e.g. depression/anxiety) should further explore and validate the findings reported here. We examined the link between DMN connectional integrity and social dysfunction, as measured independently by two different questionnaires and their mean cumulative score. However, questionnaires remain a self-reported proxy for social dysfunction of which we know it to be different – at least to some extent – from the social dysfunction perceived by others (Belfort et al. Citation2018; Jongs et al. submittted). The use of questionnaires to capture the notoriously complex phenomenon of social dysfunction is a vast simplification. However, to date, it is the best proxy available for easily accessible and reliable assessments until more sophisticated techniques are employed in this population (Eskes et al. Citation2016; Jongs et al. Citation2020). We included patients with a relatively recent disease onset and few comorbidities, in order to capture as much as possible the underlying neurobiology of social dysfunction rather than long-term consequences of psychopathology or neurodegeneration. Despite these efforts, we acknowledge that we cannot fully rule out such effects. We also did not specifically probe possible AD-specific grey matter atrophy, which is typical to more severe cases, whereas our AD patients had recent disease onset and few comorbidities. Moreover, it is good to highlight that the focus of the current paper is not on grey matter morphology but large-scale network functional connectivity, which is less susceptible to focal morphological atrophy. Our extensive image pre-processing pipeline and the data-driven network construction furthermore safeguard against confounding impact of many neurobiological features, including atrophy. In fact, the ICA/Dual Regression approach implemented here is strikingly apt in representing the topological architecture of the DMN in a reliable and consistent manner across various populations, irrespective of age, sex, or diagnostic status (Smith et al. Citation2009; Laird et al. Citation2011). Finally, the current paper has a strong transdiagnostic approach and the link between DMN disintegrity and social dysfunction is seen across the groups, not just in the AD participants (). That is in fact the beauty and strength of modern transdiagnostic psychiatry, it aims to pinpoint neuro-bio-behavioural relations independent of diagnostic classifications and their respective sequelae (Morris and Cuthbert Citation2012; Cuthbert Citation2015). Nonetheless, it is important to acknowledge that distinct neurobiological processes might underlie the link between DMN and social dysfunction in AD versus SZ patients. More specifically, while this link in AD patients may be propelled by DMN degeneration (i.e. loss of neuronal activity and subsequent network dysfunction), in SZ patients this could be more akin to circuit imbalances and neurodevelopmental anomalies within the DMN. While beyond the scope of the current study, future research should address these issues, ideally by combining longitudinal task-based and resting-state fMRI measures of DMN integrity, so to grasp activity-connectivity interdependencies.
In spite of these limitations, the study certainly adds to our understanding of transdiagnostic social dysfunction and its putative neurobiology (Insel et al. Citation2010; Kas et al. Citation2019). All data collection (including MRI) and participant-level assessments were moreover performed according to fully harmonised protocols across sites, and key clinical/sociodemographic factors corrected for, to boost robustness and reliability of our study results. We also ran network-sensitivity analyses, which reaffirmed that the documented social dysfunction effects are truly specific to the DMN. The study moreover has an innovative approach, combining two distinctive neuropsychiatric disorders with differing disease characteristics on the important topic of social dysfunction. Current findings on social dysfunction may therefore aid data-driven patient stratification initiatives given their transdiagnostic dimensional feature. Perhaps more importantly, these findings may also inform the development of more robust biomarkers and effective treatment strategies that are rooted in social behaviour, and its neurobehavioral underpinnings (Insel et al. Citation2010; Kas et al. Citation2019).
Conclusions
In summary, our findings suggest that social dysfunction transdiagnostically associates with DMN connectional disintegrity across SZ/AD patients and healthy controls. These findings pinpoint DMN connectional alterations as putative transdiagnostic endophenotypes for social dysfunction, and could plausibly aid personalised care initiatives grounded in social behaviour. These initial exploratory findings should be further validated, for example through multimodal examination of DMN connectivity and complex network analyses, to attain a more fine-grained understanding of DMN contributions to social (dys)function. The current findings could plausibly serve as a point of departure or source of hypothesis generation for these future endeavours.
Supplementary Material
Download MS Word (127.9 KB)Acknowledgements
The project leading to this application has received funding from the Innovative Medicines Initiative 2 Joint Undertaking under grant agreement No 115916. This Joint Undertaking receives support from the European Union’s Horizon 2020 research and innovation programme and EFPIA. This publication reflects only the author’s views and neither the IMI 2JU nor EFPIA nor the European Commission are liable for any use that may be made of the information contained therein.
Disclosure statement
CA has been a consultant to or has received honoraria or grants from Acadia, Angelini, Gedeon Richter, Janssen Cilag, Lundbeck, Minerva, Otsuka, Roche, Sage, Servier, Shire, Schering Plough, Sumitomo Dainippon Pharma, Sunovion and Takeda. MK has received (non-related) research funding from Novartis. BP has received (non-related) research funding from Jansen Research and Boehringer Ingelheim. ACB, AM, and GRD are fully employed by P1vital Ltd. GRD is an owner and shareholder of P1vital Ltd. and P1vital Products Ltd. IS, MA, LR, PV, YP, NW, AR, MM, SK JLAM and HM reported no financial interests or potential conflict of interests.
Data availability statement
The data that support the findings of this study are available from www.prism-project.eu but restrictions apply to the availability of these data, which were used under licence for the current study, and so are not publicly available. Data are however available upon reasonable request from www.prism-project.eu.
References
- Amft M, Bzdok D, Laird AR, Fox PT, Schilbach L, Eickhoff SB. 2015. Definition and characterization of an extended social-affective default network. Brain Struct Funct. 220(2):1031–1049.
- Anderson RJ, Hoy KE, Daskalakis ZJ, Fitzgerald PB. 2016. Repetitive transcranial magnetic stimulation for treatment resistant depression: re-establishing connections. Clin Neurophysiol. 127(11):3394–3405.
- Andrews-Hanna JR, Reidler JS, Sepulcre J, Poulin R, Buckner RL. 2010. Functional-anatomic fractionation of the brain’s default network. Neuron. 65(4):550–562.
- Andrews-Hanna JR, Smallwood J, Spreng RN. 2014. The default network and self-generated thought: component processes, dynamic control, and clinical relevance. Ann N Y Acad Sci. 1316(1):29–52.
- Andrews-Hanna JR. 2012. The brain's default network and its adaptive role in internal mentation. Neuroscientist. 18(3):251–270.
- Anticevic A, Hu X, Xiao Y, Hu J, Li F, Bi F, Cole MW, Savic A, Yang GJ, Repovs G, et al. 2015. Early-course unmedicated schizophrenia patients exhibit elevated prefrontal connectivity associated with longitudinal change. J Neurosci. 35(1):267–286.
- Badhwar AP, Tam A, Dansereau C, Orban P, Hoffstaedter F, Bellec P. 2017. Resting-state network dysfunction in Alzheimer's disease: a systematic review and meta-analysis. Alzheimers Dement (Amst)). 8:73–85.
- Bais L, Liemburg E, Vercammen A, Bruggeman R, Knegtering H, Aleman A. 2017. Effects of low frequency rTMS treatment on brain networks for inner speech in patients with schizophrenia and auditory verbal hallucinations. Prog Neuropsychopharmacol Biol Psychiatry. 78:105–113.
- Belfort T, Simões P, de Sousa MFB, Santos RL, Barbeito I, Torres B, Dourado MCN. 2018. The relationship between social cognition and awareness in Alzheimer disease. J Geriatr Psychiatry Neurol. 31(1):27–33.
- Benoit RG, Gilbert SJ, Volle E, Burgess PW. 2010. When i think about me and simulate you: medial rostral prefrontal cortex and self-referential processes. Neuroimage. 50(3):1340–1349.
- Bilderbeck AC, Penninx BWJH, Arango C, van der Wee N, Kahn R, Winter-van Rossum I, Hayen A, Kas MJ, Post A, Dawson GR, et al. 2019. Overview of the clinical implementation of a study exploring social withdrawal in patients with schizophrenia and Alzheimer's disease. Neurosci Biobehav Rev. 97:87–93.
- Birchwood M, Smith J, Cochrane R, Wetton S, Copestake S. 1990. The Social Functioning Scale. The development and validation of a new scale of social adjustment for use in family intervention programmes with schizophrenic patients. Br J Psychiatry. 157:853–859.
- Bralten J, Klemann C, Mota N, Klemann CJHM, Witte WD, Laing E, Collier DA, de Kluiver H, Baudin SEEC, Arango C, et al. 2019. Genetic underpinnings of sociability in the UK Biobank.
- Buckholtz JW, Meyer-Lindenberg A. 2012. Psychopathology and the human connectome: toward a transdiagnostic model of risk for mental illness. Neuron. 74(6):990–1004.
- Buckner RL, Andrews-Hanna JR, Schacter DL. 2008. The brain's default network: anatomy, function, and relevance to disease. Ann N Y Acad Sci. 1124:1–38.
- Bzdok D, Langner R, Schilbach L, Jakobs O, Roski C, Caspers S, Laird AR, Fox PT, Zilles K, Eickhoff SB. 2013. Characterization of the temporo-parietal junction by combining data-driven parcellation, complementary connectivity analyses, and functional decoding. Neuroimage. 81:381–392.
- Cacioppo S, Capitanio JP, Cacioppo JT. 2014. Toward a neurology of loneliness. Psychol Bull. 140(6):1464–1504.
- Che X, Zhang Q, Zhao J, Wei D, Li B, Guo Y, Qiu J, Liu Y. 2014. Synchronous activation within the default mode network correlates with perceived social support. Neuropsychologia. 63(1):26–33.
- Cuthbert BN. 2015. Research domain criteria: toward future psychiatric nosologies. Dialogues Clin Neurosci. 17(1):89–97.
- Cuthbert BN. 2019. The PRISM project: social withdrawal from an RDoC perspective. Neurosci Biobehav Rev. 97:34–37.
- de Jong-Gierveld J, Kamphuis F, Kamphuls F. 1985. The development of a Rasch-type loneliness scale. Appl Psychol Meas. 9(3):289–299.
- Dixon ML, Moodie CA, Goldin PR, Farb N, Heimberg RG, Gross JJ. 2020. Emotion regulation in social anxiety disorder: reappraisal and acceptance of negative self-beliefs. Biol Psychiatry Cogn Neurosci Neuroimaging. 5(1):119–129.
- Dixon ML, Thiruchselvam R, Todd R, Christoff K. 2017. Emotion and the prefrontal cortex: an integrative review. Psychol Bull. 143(10):1033–1081.
- Doucet GE, Janiri D, Howard R, O'Brien M, Andrews-Hanna JR, Frangou S. 2020. Transdiagnostic and disease-specific abnormalities in the default-mode network hubs in psychiatric disorders: a meta-analysis of resting-state functional imaging studies. Eur Psychiatry. 63(1):e57.
- Eskes P, Spruit M, Brinkkemper S, Vorstman J, Kas MJ. 2016. The sociability score: app-based social profiling from a healthcare perspective. Comput Human Behav. 59:39–48.
- Filiano AJ, Xu Y, Tustison NJ, Marsh RL, Baker W, Smirnov I, Overall CC, Gadani SP, Turner SD, Weng Z, et al. 2016. Unexpected role of interferon-γ in regulating neuronal connectivity and social behaviour. Nature. 535(7612):425–429.
- Folstein MF, Folstein SE, McHugh PR. 1975. "Mini-mental state". A practical method for grading the cognitive state of patients for the clinician”. J Psychiatr Res. 12(3):189–198.
- Fox JM, Abram SV, Reilly JL, Eack S, Goldman MB, Csernansky JG, Wang L, Smith MJ. 2017. Default mode functional connectivity is associated with social functioning in schizophrenia. J Abnorm Psychol. 126(4):392–405.
- Goveas JS, Xie C, Ward BD, Wu Z, Li W, Franczak M, Jones JL, Antuono PG, Li S-J. 2011. Recovery of hippocampal network connectivity correlates with cognitive improvement in mild Alzheimer's disease patients treated with donepezil assessed by resting-state fMRI. J Magn Reson Imaging. 34(4):764–773.
- Holt-Lunstad J, Smith TB, Layton JB. 2010. Social relationships and mortality risk: a meta-analytic review. PLoS Med. 7(7):e1000316.
- Hu ML, Zong XF, Mann JJ, Zheng JJ, Liao YH, Li ZC, He Y, Chen XG, Tang JS. 2017. A review of the functional and anatomical default mode network in schizophrenia. Neurosci Bull. 33(1):73–84.
- Ike KGO, de Boer SF, Buwalda B, Kas MJH. 2020. Social withdrawal: an initially adaptive behavior that becomes maladaptive when expressed excessively. Neurosci Biobehav Rev. 116:251–267.
- Insel T, Cuthbert B, Garvey M, Heinssen R, Pine DS, Quinn K, Sanislow C, Wang P. 2010. Research Domain Criteria (RDoC): toward a new classification framework for research on mental disorders. Am J Psychiatry. 167(7):748–751.
- Jongs N, Jagesar R, van Haren NEM, Penninx BWJH, Reus L, Visser PJ, van der Wee NJA, Koning IM, Arango C, Sommer IEC, et al. 2020. A framework for assessing neuropsychiatric phenotypes by using smartphone-based location data. Transl Psychiatry. 10(1):211.
- Jongs N, Penninx B, Arango C. submitted. Effect of disaese related biases on the subjective assessment of social functioning in neuropsychiatric patients.
- Kaiser RH, Andrews-Hanna JR, Wager TD, Pizzagalli DA. 2015. Large-scale network dysfunction in major depressive disorder: a meta-analysis of resting-state functional connectivity. JAMA Psychiatry. 72(6):603–611.
- Kas MJ, Penninx B, Sommer B, Serretti A, Arango C, Marston H. 2019. A quantitative approach to neuropsychiatry: the why and the how. Neurosci Biobehav Rev. 97:3–9.
- Kas MJH, Fernandes C, Schalkwyk LC, Collier DA. 2007. Genetics of behavioural domains across the neuropsychiatric spectrum; of mice and men. Mol Psychiatry. 12(4):324–330.
- Kay SR, Fiszbein A, Opler LA. 1987. The Positive and Negative Syndrome Scale (PANSS) for schizophrenia. Schizophr Bull. 13(2):261–275.
- Laird AR, Fox PM, Eickhoff SB, Turner JA, Ray KL, McKay DR, Glahn DC, Beckmann CF, Smith SM, Fox PT, et al. 2011. Behavioral interpretations of intrinsic connectivity networks. J Cogn Neurosci. 23(12):4022–4037.
- Lefort-Besnard J, Bassett DS, Smallwood J, Margulies DS, Derntl B, Gruber O, Aleman A, Jardri R, Varoquaux G, Thirion B, et al. 2018. Different shades of default mode disturbance in schizophrenia: subnodal covariance estimation in structure and function. Hum Brain Mapp. 39(2):644–661.
- Li S, Hu N, Zhang W, Tao B, Dai J, Gong Y, Tan Y, Cai D, Lui S. 2019. Dysconnectivity of multiple brain networks in schizophrenia: a meta-analysis of resting-state functional connectivity. Front Psychiatry. 10:482.
- Li W, Mai X, Liu C. 2014. The default mode network and social understanding of others: what do brain connectivity studies tell us. Front Hum Neurosci. 8:74.
- Lorenzi M, Beltramello A, Mercuri NB, Canu E, Zoccatelli G, Pizzini FB, Alessandrini F, Cotelli M, Rosini S, Costardi D, et al. 2011. Effect of memantine on resting state default mode network activity in Alzheimer’s disease. Drugs Aging. 28(3):205–217.
- Maresh EL, Allen JP, Coan JA. 2014. Increased default mode network activity in socially anxious individuals during reward processing. Biol Mood Anxiety Disord. 4(1):7–12.
- Mars RB, Neubert FX, Noonan MAP, Sallet J, Toni I, Rushworth MFS. 2012. On the relationship between the “default mode network” and the “social brain". Front Hum Neurosci. 6:189.
- Mazza M, Catalucci A, Pino MC, Giusti L, Nigri A, Pollice R, Roncone R, Casacchia M, Gallucci M. 2013. Dysfunctional neural networks associated with impaired social interactions in early psychosis: an ICA analysis. Brain Imaging Behav. 7(3):248–259.
- McKhann GM, Knopman DS, Chertkow H, Hyman BT, Jack CR, Kawas CH, Klunk WE, Koroshetz WJ, Manly JJ, Mayeux R, et al. 2011. The diagnosis of dementia due to Alzheimer's disease: recommendations from the National Institute on Aging-Alzheimer's Association workgroups on diagnostic guidelines for Alzheimer's disease. Alzheimers Dement. 7(3):263–269.
- Menon V. 2011. Large-scale brain networks and psychopathology: a unifying triple network model. Trends Cogn Sci. 15(10):483–506.
- Mills BD, Pearce HL, Khan O, Jarrett BR, Fair DA, Lahvis GP. 2016. Prenatal domoic acid exposure disrupts mouse pro-social behavior and functional connectivity MRI. Behav Brain Res. 308:14–23.
- Missault S, Anckaerts C, Ahmadoun S, Blockx I, Barbier M, Bielen K, Shah D, Kumar-Singh S, De Vos WH, Van der Linden A, et al. 2019. Hypersynchronicity in the default mode-like network in a neurodevelopmental animal model with relevance for schizophrenia. Behav Brain Res. 364:303–316.
- Mohan A, Roberto AJ, Mohan A, Lorenzo A, Jones K, Carney MJ, Liogier-Weyback L, Hwang S, Lapidus KAB. 2016. The significance of the Default Mode Network (DMN) in neurological and neuropsychiatric disorders: a review. Yale J Biol Med. 89(1):49–57.
- Morris SE, Cuthbert BN. 2012. Research domain criteria: cognitive systems, neural circuits, and dimensions of behavior. Dialogues Clin Neurosci. 14(1):29–37.
- Mulders PC, van Eijndhoven PF, Schene AH, Beckmann CF, Tendolkar I. 2015. Resting-state functional connectivity in major depressive disorder: a review. Neurosci Biobehav Rev. 56:330–344.
- Nickerson LD, Smith SM, Öngür D, Beckmann CF. 2017. Using dual regression to investigate network shape and amplitude in functional connectivity analyses. Front Neurosci. 11:115–118.
- Padmanabhan A, Lynch CJ, Schaer M, Menon V. 2017. The default mode network in autism. Biol Psychiatry Cogn Neurosci Neuroimaging. 2(6):476–486.
- Pievani M, de Haan W, Wu T, Seeley WW, Frisoni GB. 2011. Functional network disruption in the degenerative dementias. Lancet Neurol. 10(9):829–843.
- Pievani M, Pini L, Ferrari C, Pizzini FB, Boscolo Galazzo I, Cobelli C, Cotelli M, Manenti R, Frisoni GB. 2017. Coordinate-based meta-analysis of the default mode and salience network for target identification in non-invasive brain stimulation of Alzheimer's disease and behavioral variant frontotemporal dementia networks. J Alzheimers Dis. 57(3):825–843.
- Porcelli S, Van Der Wee N, van der Werff S, Aghajani M, Glennon JC, van Heukelum S, Mogavero F, Lobo A, Olivera FJ, Lobo E, et al. 2019. Social brain, social dysfunction and social withdrawal. Neurosci Biobehav Rev. 97:10–33.
- Rosen WG, Mohs RC, Davis KL. 1984. A new rating scale for Alzheimer's disease. Am J Psychiatry. 141(11):1356–1364.
- Saris IMJ, Penninx BWJH, Dinga R, van Tol M-J, Veltman DJ, van der Wee NJA, Aghajani M. 2020. Default mode network connectivity and social dysfunction in major depressive disorder. Sci Rep. 10(1):194.
- Schilbach L, Bzdok D, Timmermans B, Fox PT, Laird AR, Vogeley K, Eickhoff SB. 2012. Introspective minds: using ALE meta-analyses to study commonalities in the neural correlates of emotional processing, social and unconstrained cognition. PLoS One. 7(2):e30920.
- Schilbach L, Eickhoff SB, Rotarska-Jagiela A, Fink GR, Vogeley K. 2008. Minds at rest? Social cognition as the default mode of cognizing and its putative relationship to the “default system” of the brain. Conscious Cogn. 17(2):457–467.
- Schmälzle R, Brook O'Donnell M, Garcia JO, Cascio CN, Bayer J, Bassett DS, Vettel JM, Falk EB. 2017. Brain connectivity dynamics during social interaction reflect social network structure. Proc Natl Acad Sci USA. 114(20):5153–5158.
- Seeley WW. 2019. The salience network: a neural system for perceiving and responding to homeostatic demands. J Neurosci. 39(50):9878–9882.
- Sheehan DV, Lecrubier Y, Sheehan KH, Amorim P, Janavs J, Weiller E, Hergueta T, Baker R, Dunbar GC. 1998. The Mini-International Neuropsychiatric Interview (M.I.N.I.): the development and validation of a structured diagnostic psychiatric interview for DSM-IV and ICD-10. J Clin Psychiatry. 59(SUPPL. 20):22–33.
- Shemesh Y, Forkosh O, Mahn M, Anpilov S, Sztainberg Y, Manashirov S, Shlapobersky T, Elliott E, Tabouy L, Ezra G, et al. 2016. Ucn3 and CRF-R2 in the medial amygdala regulate complex social dynamics. Nat Neurosci. 19(11):1489–1496.
- Smith DV, Sip KE, Delgado MR. 2015. Functional connectivity with distinct neural networks tracks fluctuations in gain/loss framing susceptibility. Hum Brain Mapp. 36(7):2743–2755.
- Smith SM, Fox PT, Miller KL, Glahn DC, Fox PM, Mackay CE, Filippini N, Watkins KE, Toro R, Laird AR, et al. 2009. Correspondence of the brain's functional architecture during activation and rest. Proc Natl Acad Sci USA. 106(31):13040–13045.
- Smith SMSM, Nichols TETE. 2009. Threshold-free cluster enhancement: addressing problems of smoothing, threshold dependence and localisation in cluster inference. Neuroimage. 44(1):83–98.
- Spreng RN, Andrews-Hanna JR. 2015. The default network and social cognition. In: Brain mapping: an encyclopedic reference. Vol 3. Saint Louis: Elsevier Science; p. 165–169.
- Spreng RN, Dimas E, Mwilambwe-Tshilobo L, Dagher A, Koellinger P, Nave G, Ong A, Kernbach JM, Wiecki TV, Ge T, et al. 2020. The default network of the human brain is associated with perceived social isolation. Nat Commun. 11(1):6393–6311.
- Uddin LQ. 2015. Salience processing and insular cortical function and dysfunction. Nat Rev Neurosci. 16(1):55–61.
- van der Wee NJA, Bilderbeck AC, Cabello M, Ayuso-Mateos JL, Saris IMJ, Giltay EJ, Penninx BWJH, Arango C, Post A, Porcelli S, et al. 2019. Working definitions, subjective and objective assessments and experimental paradigms in a study exploring social withdrawal in schizophrenia and Alzheimer's disease. Neurosci Biobehav Rev. 97:38–46.
- Vázquez Morejón AJ, Jiménez G, Bóveda R. 2000. Social functioning scale: new contributions concerning its psychometric characteristics in a Spanish adaptation. Psychiatry Res. 93(3):247–256.
- Whitfield-Gabrieli S, Ford JM. 2012. Default mode network activity and connectivity in psychopathology. Annu Rev Clin Psychol. 8:49–76.
- Winograd-Gurvich C, Fitzgerald PB, Georgiou-Karistianis N, Bradshaw JL, White OB. 2006. Negative symptoms: a review of schizophrenia, melancholic depression and Parkinson's disease. Brain Res Bull. 70(4-6):312–321.
- Zott B, Busche MA, Sperling RA, Konnerth A. 2018. What happens with the circuit in Alzheimer's disease in mice and humans? Annu Rev Neurosci. 41:277–297.