Abstract
A series of bismaleimide monomers (BMI) with various structures containing flexible linkages was prepared and structural characterization of these compounds was carried out by elemental analysis, Fourier transform infrared spectroscopy, and 1H−NMR spectroscopy. The thermal behavior of these monomers (BMI(1–4)) was investigated by differential scanning calorimetry, polarized light microscopy and thermogravimetric analysis. The reactivity of the bismaleimide compounds is influenced by chemical nature of the bridge unit between the maleimide rings. The monomers were soluble in several organic solvents, the 10% weight loss temperatures were above 500 °C, and char yield at 700 °C ranged between 46 and 58% in nitrogen atmosphere. Birefringence in melting state was observed for two bismaleimides (BMI-3 and BMI-4) which could indicate a possible thermotropic liquid crystalline behavior.
1. Introduction
Bismaleimide compounds are among the most important resins employed in the aerospace/aircraft, nuclear and electrics/electronics industries because of their tractability, high thermal and thermooxidative stability, good retention of the mechanical properties even after long aging times at 250 °C, excellent chemical, radiation and corrosion resistance, good water resistance, fire resistance, and relatively low cost. Also, these resins are of great interest because of their easy processability by resin transfer molding, without formation of volatile by-products Citation[1–7].
The application areas for bismaleimide resins are reinforced composites for printed circuit boards (with glass fabrics) and structural laminates (with glass, carbon, and aramide fibers) for aero-engines and military aircraft parts Citation[8–12]. Some recent studies show that polybismaleimides have potential applications in second-order nonlinear optical (NLO) materials due to their glass transition temperatures, which maintain the critical orientation of the NLO chromophore Citation[13,14], or in fabrication process of photo-alignment layers Citation[15]. Unfortunately, these resins show some disadvantages, such as brittleness (due to their high crosslink density), poor solubility in ordinary solvents, and high melting and curing temperatures which considerably restrict their applications Citation[16,17]. The rigidity based on symmetry and aromaticity of these compounds would have contribution on the thermal and chemical stability Citation[11]. Much effort has been made to improve the mechanical and physical properties of polyaspartimides, e.g. to find new solutions systems and to modify their structures by introducing bulky substituents, incorporation of flexible linkages into the backbone, or by copolymerization Citation[18,19].
A large variety of bismaleimide monomers (BMI) having various structures and bearing end-capped reactive maleimide has been developed in recent years to improve the processability of polyaspartimides and also the physical (solubility, melting, and crosslinking temperature) and mechanical properties of the cured polymers. The reactivity of bismaleimides is determined by the ability of their double bond to add monomers bearing an active hydrogen atom, to act as a dienophyle in Alder reactions, and to enter reactions of radical and anionic homopolymerization and copolymerization with various monomers containing both unsaturated (vinyl, allyl) and saturated (propyl) bonds or with cyclic compounds (cyclobutane derivatives or heterocycles). At the same time, BMI and diamines were varied systematically with the target of identifying new systems with the lowest possible dielectric constant, without compromising the thermal properties.
Taking into consideration all these facts, we found interesting to develop new BMI containing tetrahedral carbon and/or oxygen kinking groups, bulky bromine substituents, and rigid linkages as amide or ester units in order to improve their solubility, melting, and curing temperature. Usually, the polymers (polyaspartimides) based on these BMI possess film-forming capability. Moreover, using kinking groups and amide/ester linkages in the same molecule facilitates the mesomorphic behavior, a very important property to obtain high resistant materials by processing from ordered molten.
2 Experimental
2.1 Materials
The materials used in this research were maleic anhydride, 4-aminobenzoic acid, 3,3′-dimethoxybenzidine, triethylamine, hexane, palladium (II) chloride (Pd: 60%), hydrazine monohydrate (Fluka), thionyl chloride, acetic anhydride (Merck), (3,3′,5,5′-tetrabromobisphenol A, 4,4′-(1-phenylenethylidene)bisphenol, 2,2′-bis[4-(4-amino)phenoxyphenyl]propane (Aldrich), and sodium carbonate (Chimopar SA). 1-Chloro-4-nitrobenzene (Fluka) was recrystallized from ethanol. Commercial solvents such as acetone, 1,2-dichloroethane (Chimopar SA), N,N-dimethylformamide, ethanol (Fluka), petroleum ether, and chloroform (Chemical Company) were dried and purified by standard methods. Magnesium acetate (Fluka) and potassium carbonate (Chimopar SA) were dried at 120 °C in vacuum before use.
2.2 Synthesis of bismaleimides
Bismaleimides BMI(1–2) were synthesized according to the methods given in the literature Citation[20] (Scheme ). General procedure: 0.02 mol of diamine in 50 mL acetone was reacted with maleic anhydride (0.04 mol) in 40 mL acetone to give the corresponding bismaleamic acid. The mixture was stirred for 30 min at 20–25 °C, and then the temperature was raised to 40 °C. Over a period of 10 min, triethylamine (TEA) (0.02 mol), acetic anhydride (0.15 mol), and magnesium acetate (0.04 g) were added. The mixture was refluxed for 1.0 h under nitrogen atmosphere. The hot solution was filtered, cooled, and poured into cold water. The product was washed with a solution of sodium carbonate (10%) until free from acetic acid. Finally, it was washed with water and dried in vacuum.
BMI-1 was recrystallized from acetone/ethanol; yield 71%; mp 117 °C; Fourier transform infrared spectroscopy (FTIR) (KBr): ν (cm−1) = 1773 and 1716 (C=O imide), 1253 (–O–). Anal. calcd for C35H22N2Br4O6 (886.210) (%): C 47.43, H 2.50, N 3.16, Br 36.06; found: C 47.11, H 2.76, N 2.95, Br 35.73.
1 H NMR δ (ppm): 7.78–7.68 (m, Ar, 4H, ortho to C(CH3)), 7.38–7.29 (m, Ar, 4H, ortho to = N–), 7.16 (s, 4H, olefinic protons), 6.91–6.89 (d, Ar, 4H, ortho to –O–), 1.74 (s, 6H, CH3).
Bismaleimide BMI-2 was recrystallized from DMF; yield 68%; mp 137 °C; FTIR (KBr) cm−1: ν (cm−1) = 1765 and 1710 (C=O imide), 1256 (–O–). Anal. calcd. for C22H16N2O6 (404.382) (%): C 65.34, H 3.98, N 6.92; found: C 65.73, H 4.15, N 6.73. 1H NMR δ (ppm): 7.45 (s, Ar, 4H, meta to = N–), 7.37–7.29 (s, Ar, 4H, ortho to = N– and ortho to OCH3), 7.22 (s, 4H, olefinicprotons), 3.86 (s, 6H, CH3).
2,2′-Bis[4-(4-nitrophenoxy)-3,5-dibromophenyl]propane, bearing both flexible ether and isopropylidene bridges between the phenylene units, and tetrabromo substituents on the phenylene rings, was synthesized according to Ref. Citation[21] by the reaction of 1-chloro-4-nitrobenzene with 3,3′,5,5′-tetrabromobisphenol A (Scheme ). After recrystallization from acetone/ethanol, the nitroderivative was reduced with a 5% Pd/C catalyst and hydrazine hydrate. The diamine obtained was recrystallized from ethanol (yield 68%, mp 254–256 °C).
Brominated bisphenols were prepared according to the literature Citation[22] as follows: in a 250 mL flask, 0.04 mol bisphenol was dissolved in 200 mL acetic acid. To this solution, 0.14 mol of bromine was added slowly, under stirring. The reaction was carried out at room temperature for 20 min, and then the temperature was raised up to 60 °C. Finally, the reaction temperature was maintained at 80–85 °C for 1.5 h to ensure complete bromination. After cooling, the product was filtered and washed with a sodium bicarbonate solution to remove the excess of bromine. The final product was recrystallized from acetic acid.
4-Maleimido benzoic acid (4-MBA) was prepared as described in the literature Citation[23], mp 225–230 °C (recrystallized from toluene); Ref. Citation[24], mp 227–230 °C.
4-Maleimidobenzoyl chloride (4-MBC) was obtained as described in the literature Citation[25], mp 168–171 °C; Ref. Citation[26], mp 170 °C.
Bismaleimide BMI-3 was synthesized following the procedure described in the literature Citation[27] (Scheme ). 4-MBC (0.04 mol) in 50 mL acetone was cooled in an ice bath. To this solution, TEA (0.04 mol) as an acid acceptor and a solution of (4,4′-(1-phenylethylidene)diphenol (0.02 mol) in 40 mL acetone were added. After stirring for 1.0 h, the mixture was filtered and precipitated in petroleum ether. The product was filtered and treated with a sodium bicarbonate solution and washed thoroughly with water. The filtered precipitate was dried in a vacuum oven at 100 °C. The product was recrystallized from acetone/ethanol; yield 69%; mp 225–230 °C; FTIR (KBr) cm−1: ν (cm−1) = 1776 and 1717 (C=O imide), 1732 (C=O ester). Anal. calcd. for C42H28N2O8 (688.799) (%): C 73.23, H 4.09, N 4.07; found: C 72.68, H 3.96, N 4.31.
Bismaleimide BMI-4 was prepared in a similar manner as BMI-3. Diamine (2,2-bis[4-(4-amino)phenoxyphenyl]propane (0.01 mol) in 50 mL CHCl3 was cooled in an ice bath. TEA (0.03 mol) and a solution of 4-MBC (0.02 mol) in 50 mL CHCl3 were added. After stirring for 1.0 h, the mixture was filtered and precipitated in hexane. The product was filtered and treated with a sodium bicarbonate solution, and then washed thoroughly with water. The precipitated product was recrystallized from acetone/water; yield 73%; mp 230–234 °C; FTIR (KBr) cm−1: ν (cm−1) = 1775 and 1718 (C=O imide), 1226 (–O–), 3376 (–NH), 1657 and 1500 (C=O amide). Anal. calcd for C49H36N4O8 (808.853) (%): C 72.76, H 4.48, N 6.92; found: C 72.13, H 4.73, N 6.65.
2.3 Measurements
The FTIR spectra were recorded on a Bruker Vertex 70 spectrophotometer and the 1H−NMR spectra were recorded on a Bruker NMR spectrometer Avance DRX 400 MHz, using DMSO-d 6 as solvent and tetramethylsilane as internal standard. Differential scanning calorimetry (DSC) measurements were done with a Du Pont Instruments 912 DSC apparatus, at a heating rate of 10 °C/min in air. Melting points were determined with a Gallenkamp hot-blok melting point apparatus. Polarized light microscopy (PLM) investigations were performed with an Olympus BH-2 polarized light microscope fitted with a THMS 600/HSF9I hot stage, magnification 400x. Thermogravimetric analysis (TGA) was carried out using a Mettler Toledo TGA/SDTA851e-thermogravimetric analyzer, under nitrogen flow (20 mL/min) at a heating rate of 10 °C/min from room temperature to 700 °C, and at 3–5 mg of sample mass.
3 Results and discussion
The structure of the bismaleimide compounds was confirmed by elemental analysis, FT-IR and 1H−NMR spectroscopies. The elemental analysis values are in good agreements with the calculated ones.
The FTIR spectra of monomers BMI(1–4) showed characteristic carbonyl doublets in the 1776–1765 and 1718–1710 cm−1 range. Bismaleimides BMI(1–2) and BMI-4 showed an absorption band at 1256–1226 cm−1 attributed to the ether group, whereas BMI-3 and BMI-4 exhibited bands at 1732 and 3379 cm−1 due to the ester carbonyl and NH units, respectively. In addition, BMI-4 showed absorption bands at 1656 and 1500 cm−1 ascribed to amide C=O. Figure presents the BMI-2 spectrum as an example.
All bismaleimides BMI(1–4) were characterized by 1H-NMR spectra, which confirmed their chemical structure. show 1H−NMR spectra of compounds BMI-3 and BMI-4, respectively. The aromatic protons of monomer BMI-3 appeared as doublets in the 8.26–8.24 ppm range (attributed to the protons ortho to ester C=O) and 7.65–7.64 ppm range (due to the protons ortho to maleimido). The signals in the 7.29–7.26 ppm range were ascribed to the aromatic protons ortho to ester –O– and para to –C(CH3)–, and olefinic protons. Other aromatic protons were evidenced in the 7.20–7.18 (meta to ester –O–), 7.38–7.35 (meta to –C(CH3)–), and 7.15–7.13 ppm range (ortho to –C(CH3)–). The singlet at 2.22 ppm was attributed to the protons of the methyl group.
Figure shows that the aromatic protons of bismaleimide BMI-4 appeared as five separated doublets in the 8.07–8.05 (4H, ortho to amide –C=O), 7.81–7.79 (4H, ortho to –NH–), 7.54–7.52 (Ar, 4H, ortho to –maleimido), 7.06–7.04 (Ar, 4H, para to –NH–), and 6.93–6.91 ppm ranges (Ar, 4H, ortho to –O–). Another doublet in the 7.25–7.24 ppm ranges was attributed to olefinic protons and aromatic protons ortho to –C(CH3)2–). A singlet at 1.65 ppm was due to methyl protons and another singlet at 10.35 ppm appeared due to the –NH proton.
The solubility of the BMI in various solvents was evaluated (Table ). Bismaleimides BMI(1–3) exhibited good solubility in NMP, DMF, DMSO, and CHCl3, while BMI-4 is less soluble. All BMIs are soluble in DMF, NMP, and CHCl3, but some differences appeared in the case of DCE, DMSO, toluene, and acetone. Introduction of flexible ether linkages, isopropylidene bridge between phenylene rings, bulky bromine, and other substituents decreases the interchain interaction and backbone rigidity, reduce the crystallinity and enhance the solubility of these compounds. The reduced solubility of BMI-4 is explained by the presence in its structure of rigid amide groups.
Table 1. Solubility of monomers BMI(1–4)a.
The thermal behavior of monomers BMI(1–4) was investigated by DSC, hot-stage PLM, and TGA measurements. DSC curves are presented in Figure , while thermal data are enclosed in Table . Figure shows that the BMI(1–3) samples exhibited both endothermic and exothermic peaks in the first heating scan. The DSC curves for BMI(1–3) were characterized by a melting endotherm plotted in the 123–230 °C range, due to the melting of these monomers (Table ).
Table 2. Thermal properties of bismaleimides BMI(1–4).
As a rule, the melting point of a compound increases with its molar mass, although this was apparently not the case of BMI-1 (the presence of bromine led to a decrease of the melting point). At the same time, melting is influenced by the molecular chemical structure. It was observed that the presence of electron withdrawing groups in bismaleimide affected the melting and curing temperature of the compounds BMI-3 and BMI-4. The reactivity of these compounds was in the 170–243 °C temperature range.
BMI contain functional reactive end units that can undergo homo and/or copolymerization by thermal or catalytic means. The reactivity of the C=C double bond is a consequence of the electron withdrawing nature of the two adjacent carbonyl groups which create a very electron-deficient double bond and therefore it is susceptible to homo and copolymerization reactions. But it has to be mentioned that the reactivity of bismaleimides is also influenced by the chemical structure of the residue between the maleimide end groups and its rigidity Citation[1,27,28]. The higher the electron-withdrawing capacity of the maleimide substituents, the higher the curing temperature and slower the curing rate, and the opposite effects in the case of groups. Hence, the high onset of BMI-3 curing might be due to the high electron-withdrawing nature of the ester group. The maximum of the exothermic peak of BMI(1–3) was observed in the 199–286 °C region. While samples BMI(1–3) exhibited only one endothermic and one exothermic peak, the sample BMI-4 showed two pairs of endo–exo peaks before melting.
Two endothermic peaks at 146 and 186 °C and two exothermal peaks at 164 and 192 °C were observed (Figure ). This behavior could be explained by crystal to crystal transitions. After heating up to 220 °C, cooling down to room temperature and then resuming the heating cycle up to 340 °C, the DSC curve evidenced one endothermic peak at 253 °C and an exothermal peak at 304 °C. This complex thermal behavior of the sample BMI-4 could be due to a possible thermotropic liquid crystalline behavior, and therefore we investigated the samples BMI-3 and BMI-4 by PLM. The results are presented in Table and Figure .
Table 3. Some PLM data for bismaleimides BMI-3 and BMI-4.
Figure 5 Space fill models of bismaleimides BMI-3 and BMI-4 after modeling using molecular dynamic. The rigid core part of the two mesogenic branches was evidenced.
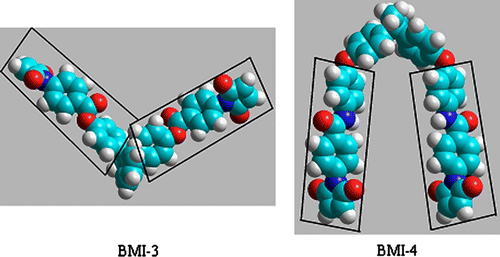
Sample BMI-4 showed no visible changes in optical microscopy up to 240 °C. This fact supported the assignment of the endo–exo thermal transitions from DSC curve (Figure ) as solid–solid transitions. At 240 °C a melting process led to a grainy texture. When sheared the upper lamella we observed a viscous birefringent fluid (Figure ). This fact allowed us to assign the endothermic peak at 242 °C (Figure b) to a solid–liquid crystalline phase transition. When temperature increased, no change in the aspect of texture was observed up to 250 °C, when an isotropic liquid occurred. Consequently, we assigned the small endothermic peak at 250 °C (Figure ) to a liquid crystal-isotropic phase transition. A similar thermal behavior was observed for sample BMI-3, except the solid–solid thermal transitions. The sample melted into a birefringent fluid at 230 °C and this birefringence disappeared slowly around 250 °C. Bismaleimides BMI-3 and BMI-4 contained two symmetric polar moieties (aromatic ester for BMI-3 and aromatic amide for BMI-4) into their structure, linked together by a nonpolar central unit (Figure ). As a result, the two symmetrical rigid branches could act as calamitic mesogens.
Figure 6 Optical polarized light microscopy images of: (a) BMI-3, 233 oC, magnification 400× and (b) BMI-4, 239 oC, magnification 400×.
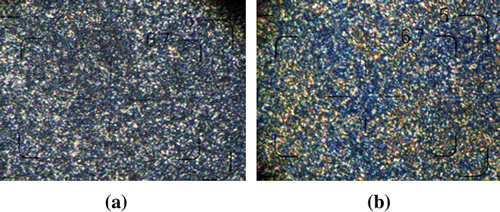
It was reported that the hydrogen bonds in amide calamitic liquid crystals led to rich smectic mesomorphism Citation(29,30). The granular texture observed for samples BMI-3 and BMI-4 was difficult to assign to a known classical liquid crystalline phase Citation(31). When the temperature increases, the tetrahedral carbon and ether linkages gain enough energy for rotational movements. To accommodate the movements, the mesogenic cores undergo rotational tilts about intermolecular forces, the order is retained by virtue of chain rigidity, but there is one component of rotational disorder, generating the birefringence and fluidity of the sample. The resulted mesophase, named plastic mesophase, was observed for many types of rigid or semiflexible polymers Citation(32–34). Besides, a systematic study on dimeric model compounds indicated the influence of polydispersity on its occurrence Citation(35). In the cooling score, the mesophase did not appear, the most probably because of crosslinking, which generated a large rigid network.
The thermal stability of the samples was investigated by TGA in nitrogen atmosphere. In addition, the thermal stability of compounds BMI-3 and BMI-4 was investigated by TGA in air. Figure presents TGA traces of bismaleimides BMI(2–4) in nitrogen atmosphere. Some TGA data for all monomers are listed in Table . The initial decomposition temperatures (IDT) ranged between 334 and 449 °C, and a rapid weight loss occurred in the 358–686 °C region. It was observed that BMI-3 had the highest IDT value and BMI-1 the lowest.
From these thermograms we observed that brominated aromatic compounds were less stable than unbrominated ones, but with a higher char yield (Table ). This was to be expected, because the substitution of the aromatic rings reduced their thermal stability due to the earlier decomposition of C-Br bond Citation[22]. The higher char yield of BMI-1 can be explained by generation of thermally stable rearranged products after releasing bromine from the polymer structure. DTG curves polymer show two maxima of decomposition (BMI-1, BMI-2, and BMI-4) for the degradation process. The multistage decomposition observed for these monomers is due to the combination of the chemically different segments in the polymer chain. The first (PDT1 max) occurring in the 350–448 °C range was probably due to the destruction of the groups more sensitive to degradation. The second maximum (PDT2 max) of decomposition was in the range of 475–680 °C, being due to the degradation of the polymer chain itself. The bismaleimide BMI-3 degrades in a single step with a maximal decomposition temperature of 460 °C. In addition, the thermal stability of BMI-3 and BMI-4 was investigated by TGA in air. The IDT values of cured compounds were about 20 and 45 °C lower, respectively. The bismaleimide BMI-1 showed the highest value of char yield at 700 °C in nitrogen, due to the presence of bromine in its structure.
4 Conclusions
New bismaleimides with ether, ester, and amide units were prepared by reacting maleic anhydride with different diamines (BMI-1 and BMI-2), or by reacting 4-maleimidobenzoyl chloride with bisphenol (BMI-3) and diamine (BMI-4). The structure of these monomers was confirmed by spectroscopy (FTIR and 1H−NMR) and elemental analysis. Incorporation of ether and ester units into the aromatic main chain of these monomers gave products with improved solubility. The compounds had good thermal stability with decomposition temperatures above 400 °C, except BMI-1, which shows an IDT value at around 375 °C. BMI-3 and BMI-4 showed a plastic mesophase in the first melting scan, and strong birefringence was observed by PLM measurements.
Acknowledgment
The author expresses his sincere appreciation to Dr Vasile Cozan for his contributions in PLM investigations.
References
- Stenzenberger , HD . 1994 . Additional polyimides . Advances in Polymer Science , 117 : 165 – 220 .
- Dumont , F , Visseaux , M , Barbier-Baudry , D and Dormond , A . 2000 . New aromatic diamines containing a multiring flexible skeleton for the synthesis of thermally stable polyimides . Polymer , 41 : 6043 – 7 .
- Rozenberg , BA , Dzhavadyan , EA , Morgan , R and Shin , E . 2002 . High-performance bismaleimide matrices: cure kinetics and mechanism . Polymers for Advanced Technologies , 13 : 837 – 44 .
- Sava , M , Gaina , C , Gaina , V , Chiriac , C , Stoica , Gh and Hamciuc , C . 1998 . Preparation and characterization of bismaleimides containing ether bonds . Designed Monomers and Polymers , 1 ( 2 ) : 135 – 45 .
- Kriegel , RM , Saliba , KL , Jones , G , Schiraldi , DA and Collard , DM . 2005 . The rapid chain extension of anthracene-functional polyesters by the Diels-Alder reaction with bismaleimides . Macromolecular Chemistry and Physics , 206 : 1479 – 87 .
- Shu , W-J and Tsai , R-S . 2010 . Studies of silicon-containing bismaleimide resins part I: synthesis and characterization of model compounds and polyaspartimides . Designed Monomers and Polymers , 13 : 33 – 49 .
- Takeichi , T , Saito , Y , Agag , T , Muto , H and Kawauchi , T . 2008 . High-performance polymer alloys of polybenzoxazine and bismaleimide . Polymer , 49 : 1173 – 9 .
- Sava , M , Gaina , C , Gaina , V and Timpu , D . 2001 . Synthesis and characterization of some bismaleimide containing ether groups in the backbone . Macromolecular Chemistry and Physics , 202 : 1601 – 5 .
- Reghunadhan Nair , CP . 2004 . Advances in addition-cure phenolic resins . Progress in Polymer Science , 29 : 401 – 98 .
- Zhang , X , Li , Z-C , Li , K-B , Lin , S , Du , F-S and Li , F-M . 2006 . Donor/acceptor vinyl monomers and their polymers: synthesis, photochemical and photophysical behavior . Progress in Polymer Science , 31 : 893 – 948 .
- Szalai , ML , McGrath , DV , Wheeler , D , Zifer , T and McElhanon , JR . 2007 . Dendrimers based on thermally reversible furan-maleimide Diels-Alder adducts . Macromolecules , 40 : 818 – 23 .
- Mzani , O , Ben Ramdhane , H , Bakloutf , M and Mercier , R . 2010 . Preparation of new polyimides by Diels-Alder reaction of various bis-pyrones and bismaleimide of methylenedianiline (BMI-MDA) . Designed Monomers and Polymers , 13 : 143 – 56 .
- Wu , W , Wang , D , Zhu , P , Wang , P and Ye , C . 1999 . Thermally stable second-order nonlinear optical addition-type polyimides functionalized by diamine chromofore . Journal of Polymer Science Part A: Polymer Chemistry , 37 : 3598 – 605 .
- Mather , BD , Viswanathan , K , Miller , KM and Long , TE . 2006 . Michael addition reactions in molecular design for emerging technologies . Progress in Polymer Science , 31 : 487 – 531 .
- Muray , H , Nakata , T and Goto , T . 2002 . Liquid crystal photo-alignment layers made from aromatic bismaleimides . Liquid Crystals , 29 ( 5 ) : 669 – 73 .
- Cai , Y , Jiang , Z , Yang , D and Liu , P . 1998 . Structure and thermal properties of PU/P(BMIUBMI) IPNs . Journal of Applied Polymer Science , 68 : 1689 – 94 .
- Lowe , A , Fox , B and Otieno-Alego , V . 2002 . Interfacial ageing of high temperature carbon/bismaleimide composites . Composites: Part A , 33 : 1289 – 92 .
- Hsiao , SH and He , MH . 2001 . Synthesis and properties of novel cardo aromatic poly(etheroxadiazole)s and poly(etheramineoxadiazole)s . Macromolecular Chemistry and Physics , 202 : 3579 – 89 .
- Gillo , M , Ianelli , P , Laurienzo , P , Malinconico , M , Roviello , A , Mormile , P and Petti , L . 2002 . Alkoxy-substituted poly(p-phenylene 1,3,4-oxadiazole)s. Synthesis, chemical characterization, and electro-optical properties . Chemistry of Materials , 14 : 1539 – 47 .
- Takeda , S , Akiyama , H and Kakiuchi , H . 1988 . Synthesis and properties of bismaleimides resins containig ether bonds . Journal of Applied Polymer Science , 35 : 1341 – 50 .
- Feld , WA , Ramalingam , B and Harris , FW . 1983 . Polyimides containing oxyethylene units . Journal of Polymer Science-Polymer Chemistry Edition , 21 : 319 – 28 .
- Yang , CP and Hsiao , SH . 1988 . Flameproofed polyesters prepared by direct polycondensation of aromatic dicarboxylic acids and brominated bisphenols with tosyl chloride and N,N’-dimethylformamide in pyridine . Journal of Applied Polymer Science , 36 : 1221 – 32 .
- Park , JO and Jang , SH . 1992 . Synthesis and characterization of bismaleimide from epoxy resin . Journal of Polymer Science Part A: Polymer Chemistry , 30 : 723 – 9 .
- Hoyt , AE and Benicewicz , BC . 1990 . Rigid rod molecules as liquid crystal thermosets. I. Rigid rod amides . Journal of Polymer Science Part A: Polymer Chemistry , 28 : 3403 – 15 .
- Liu , FJ , Munukutia , S , Levon , K and Tesoro , GJ . 1992 . Synthesis and characterization of some imide end-capped amid-imides . Journal of Polymer Science Part A: Polymer Chemistry , 30 : 157 – 62 .
- Hoyt , FJ and Benicewicz , BC . 1990 . Rigid rod molecules as liquid crystal thermosets. Rigid rod ester . Journal of Polymer Science Part A: Polymer Chemistry , 28 : 3417 – 27 .
- Nagai , A , Takahashi , A , Suzuki , M , Katagiri , J and Mukoh , A . 1990 . Thermal behavior and cured products of mixtures of two different bismaleimides . Journal of Applied Polymer Science , 41 : 2241 – 50 .
- Rao , BS . 1989 . Synthesis, characterization and thermal stability of bismaleimides derived from maleimido benzoic acid . Journal of Polymer Science Part A: Polymer Chemistry , 27 : 2509 – 18 .
- Kajitani , T , Kohmoto , S , Yamamoto , M and Kishikawa , K . 2005 . Calamitic liquid crystalline molecules with lateral intermolecular hydrogen bonding . Molecular Crystals and Liquid Crystals , 439 : 173 – 7 .
- Damaceanu , MD , Marin , L , Manicke , T and Bruma , M . 2009 . Solid-state properties of mesomorphic copolymers containing oxadiazole and fluorene units . Soft Matter , 7 : 164 – 84 .
- Barbera , J , Oriol , L and Serrano , JL . 1992 . Hydroxy-functionalized liquid-crystalline polyazomethines I. Synthesis, characterization and structure-mezogenic behavior relationship . Liquid Crystals , 12 : 37 – 47 .
- Windle AH. Structure of thermotropic main chain polymers. In: Shibaev VP, Lam L, editors. Liquid crystalline and mesomorphic polymers. Springer-Verlag; 1994. p. 26–76.
- Marin , L , Cozan , V and Bruma , M . 2006 . Comparative study of new thermotropic polyazomethines . Polymers for Advanced Technologies , 17 : 664 – 72 .
- Marin , L , Damaceanu , MD and Timpu , D . 2009 . New thermotropic liquid crystalline polyazomethine containing luminescent mesogens . Soft Matter , 7 : 1 – 20 .
- Marin , L , Zabulica , A and Sava , M . 2011 . New symmetric azomethinic dimer: the influence of structural heterogenity on the liquid crystalline behavior . Liquid Crystals , 38 : 433 – 40 .