Abstract
Novel amphiphilic graft copolymers of polysulfone (PSU) possessing hydrophilic poly(2-oxazoline) (POx) side chains were synthesized via the ‘grafting from’ method. The cationic ring-opening polymerizations of 2-methyl-2-ozaxoline, 2-ethyl-2-ozaxoline, and 2-propyly-2-ozaxoline were initiated by chloromethylated polysulfone macroinitiator (with 15% benzylchloride content), synthesized by chloromethylation of PSU, in the presence of sodium iodide. The effects of reaction time and type of monomer on the yield, and degree of polymerization were investigated. The obtained polymers were characterized by proton nuclear magnetic resonance spectroscopy, Fourier transform infrared spectroscopy, and differential scanning calorimetry analyses. Thin films of graft copolymers were investigated by atomic force microscopy and contact angle measurements. The observed morphologies of thin films were consistent with the amphiphilic nature of the graft copolymers. The films showed temperature dependent surface hydrophobicity due to thermo responsiveness of POx.
1. Introduction
Polysulfones (PSU) are industrially important high-performance thermoplastics with attractive features such as high strength and stiffness even at elevated temperatures, high continuous use and heat deflection temperatures, excellent resistance to hydrolysis, acids and bases as well as good dimensional stability even in complex geometric shapes Citation[1]. These materials also offer further desirable mechanical properties such as low susceptibility to environmental stress cracking and extremely high-notched impact strength on the level of polycarbonate. Combined with these outstanding properties, PSUs are frequently used as biomaterials since they can endure all sterilization techniques (steam, ethylene oxide, and gamma radiation) Citation[2,3]. More recently, research on PSUs has focused on membrane technologies and biomedical applications such as hemodialysis, water purification, gas separation, cell culture, drug delivery, bioartificials, and fuel cells that require easy manufacturing processes allowing reproducible properties and controllable pore size Citation[2–10]. However, the hydrophobic nature of PSUs restricts their usage as biomaterials in the filtration of protein containing solutions and blood contacting applications. In order to overcome problems associated with the hydrophobicity, it is necessary to modify the PSU structure with hydrophilic groups or segments Citation[11,12]. There is a variety of modification techniques for imparting amphiphilic character to PSUs. Many reports for the modification have been based on the reactive functionalization Citation[13–19] followed by polymerization through these functional groups. Typically, sulfonation or halogenation reactions were used to introduce initiating or reactive sites for blocking and grafting of hydrophilic monomers or oligomers, respectively Citation[20–23].
Polyoxazolines (POx) have aroused considerable interest in recent years and have been the subject of a number of studies concentrating on thermally activated living cationic ring-opening polymerization (LCROP) of 2-substituted derivatives with mostly ethyl, methyl, propyl, or isopropyl substitutions Citation[24–29]. The living cationic ring-opening polymerization of 2-alkyl-2-oxazolines can be initiated by electrophilic species like alkyl halides, Lewis and protonic acids. The polymerization proceeds without chain breaking reactions and enables to synthesize well-defined macromolecular structures including block or graft copolymers Citation[30–34]. Poly(2-alkyl-2-oxazolines) particularly with ethyl or methyl side chains have been regarded as biocompatible material similar to the well-studied poly(ethylene oxide) Citation[35–37]. Some critical properties of the polymer like crystallinity, hydrophilicity, solubility, and chirality can be designated by the nature of the alkyl substituents in the side chain. Although POx with long alkyl side chains are hydrophobic and soluble only in organic solvents, shorter side chain substituents make the polymer hydrophilic and soluble in water under a lower critical solution temperature Citation[38–41]. For instance, poly(2-ethyl-2-oxazoline) (PEtOx) shows a lower critical solution temperature of ∼60 oC. It is hereby considered as a thermoresponsive, nontoxic, and biocompatible material Citation[42–45].
Amphiphilic graft or block copolymers offer integration of distinctive properties of different polymers into one macromolecular structure. Copolymers involving hydrophilic POx chains can be found in several self-organized formations like micelles, microemulsions, and adsorbed polymer layers which make them potential candidates for biodegradable drug delivery systems and biocompatible membrane applications Citation[25,46,47]. Preparation of graft copolymers with hydrophilic POx graft arms and hydrophobic backbone can be carried out via grafting-from methods in which the ring-opening polymerization of 2-oxazoline monomers is started by a polymeric macroinitiator Citation[48–50].
In this work, we report a novel approach to prepare amphiphilic graft copolymers of an engineering polymer, PSU with POx side chains by chemical modification followed by cationic ring-opening polymerization. The obtained graft copolymers were characterized and properties were evaluated on the basis of monomer type.
2 Experimental
2.1 Materials
Solutions of 2-Methyl-2-oxazoline (MeOx) (Aldrich, 98%), 2-ethyl-2-oxazoline (EtOx) (Aldrich, 99%), and 2-propyl-2-oxazoline (PrOx) (TCI, 98%) were distilled twice over calcium hydride under vacuum and stored under a dry nitrogen atmosphere. Diethyl ether and methanol were distilled before used. Potassium iodide (KI) (Aldrich) was used as received. Polysulfone, PSU UDEL P-1700 (M n: 29,000) was obtained from Solvay Advanced Polymers. Chloroform (Alfa-Aesar, 99.5%), paraformaldehyde (Aldrich, 95%), tin(IV)chloride (SnCl4) (Aldrich, 99.995%), and chlorotrimethylsilane ((CH3)3SiCl) (Merck, 99%) were used as purchased.
2.2 Characterization
Proton nuclear magnetic resonance (1H NMR) spectra of 5–10% (w/w) solutions of the intermediates and final polymers in CDCl3 with Si(CH3)4 as an internal standard were recorded at room temperature at 250 MHz on a Bruker DPX 250 spectrometer. Fourier transform infrared (FT-IR) spectra were recorded on a Perkin-Elmer FT-IR Spectrum One B spectrometer. A heatable single reflection ZnSe crystal was used for sampling. The samples were directly dispensed on the ZnSe crystal and analyses were performed at room temperature. Differential scanning calorimetry (DSC) analyses were performed on a Perkin-Elmer Diamond DSC in the range of 30–300 °C with a heating rate of 10 °C min −1 under nitrogen flow.
For morphological investigations, thin films of graft copolymers were spin coated on silicon substrates from 1 mg/ml solutions in THF (tetrahydrofuran). The morphology of the films was characterized by atomic force microscopy (AFM) in tapping mode. AFM measurements were carried out at room temperature in air using NT-MDT Solver P47 instrument. NT-MDT Etalon HA_NC type silicon cantilevers were used.
Contact angle (CA) measurements were performed by Dataphysics contact angle meter OCA20. The instrument had a video-based system with CCD camera for the observation of water drop. CA was determined by SCA20 software. The instrument was equipped with temperature control chamber with a Peltier system for the software controlled temperature setting.
2.3 Synthesis of macroinitiator (chloromethylated PSU)
Chloromethylated PSU was prepared by the modification of PSU according to the described procedure Citation[51]. Under inert atmosphere, PSU (5.0 g, 11.3 mmol of repeating units) was dissolved in 150 mL of chloroform in a round-bottom flask. Subsequently paraformaldehyde (1.5 g, 50 mmol), the catalyst SnCl4 (0.15 mL, 1.30 mmol), and the chloromethylation agent (CH3)3SiCl (6.4 mL, 50 mmol) were charged into the reaction vessel. The mixture was kept at 40 °C for 2 days under stirring. Afterward, the product was precipitated in methanol and the separated polymer was then filtered and washed several times with methanol and lastly dried under vacuum at 50 °C for 24 h. A 5.1 g of the product was obtained as white powder.
FTIR (ATR, cm −1): 3200–3000 (Ar), 2975 (–CH3 asym-), 2845 (CH3 sym-), 1322 and 1293 (O=S=O asym-), 1235 (C–O–C), 1151 and 1175 (O=S=O sym–), 1011 (Ar), 750 (C–Cl).
1H NMR (CDCl3, ppm): δ = 7.76 (O2S–Ar–H meta position), 7.21 (Me2C–Ar–H ortho position), 7.00 (O2S–Ar–H ortho position) 6.94 (Me2C–Ar–H meta position), 4.50 (CH2–Cl), 1.65 (C–Me2).
2.4 Synthesis of graft copolymers PSU-g-poly(2-alkyl-2-oxazoline)s
All graft copolymerization reactions were performed in bulk. For every graft copolymerization, 0.050 g of the macroinitiator, 0.05 g of potassium iodide, and 2 g of 2-alkyl-2-oxazoline were placed into a round-bottom flask under inert atmosphere and stirred at 120 °C. At end of the polymerization, the reaction mixture was diluted with 3 mL of chloroform and subsequently poured in 100 mL of diethyl ether to precipitate the graft copolymer. Finally, the polymer was dried under vacuum at 50 °C for 24 h.
2.4.1 PSU-g-poly(2-methyl-2-oxazoline)
FTIR (ATR, cm−1): 3470 (OH), 2936 (CH), 1634 (C=O), 1416 (CH, PolyMeOx).
1H NMR (CDCl3, ppm): δ = 7.83 (PSU), 7.23 (PSU), 7.01 (PSU) 6.93 (PSU), 3.3–3.7 (N–CH2, PolyMeOx), 2.0 and 2.3 (C(O)CH3, PolyMeOx), 1.69 (PSU).
2.4.2 PSU-g-poly(2-ethyl-2-oxazoline)
FTIR (ATR, cm−1): 3465 (OH), 2936 (CH), 1630 (C=O), 1420 (CH, PolyEtOxa).
1H NMR (CDCl3, ppm): δ = 7.83 (PSU), 7.23 (PSU), 7.01 (PSU) 6.93 (PSU), 3.3–3.7 (N–CH2, PolyEtOxa), 2.2 and 2.6 (C(O)CH2, PolyEtOxa), 1.69 (PSU), 1.1 (CH3, PolyEtOxa).
2.4.3 PSU-g-poly(2-propyl-2-oxazoline)
FTIR (ATR, cm−1): 3465 (OH), 2936 (CH), 1635 (C=O), 1410 (CH, PolyPrOxa).
1H NMR (CDCl3, ppm): δ = 7.83 (PSU), 7.23 (PSU), 7.01 (PSU) 6.93 (PSU), 3.3–3.7 (N–CH2, PolyPrOxa), 2.2 and 2.6 (C(O)CH2, PolyPrOxa), 1.7 (PSU), 1.64 (–CH2–, PolyPrOxa), 0.96 (CH3, PolyPrOxa).
3 Results and discussion
The synthetic approach for the preparation of amphiphilic graft copolymers involves two steps. Scheme shows the overall synthetic route of graft copolymers composed of PSU backbone and poly(2-alkyl-2-oxazoline) side chains.
In the first step, chloromethylated PSU (PSU–CH2Cl) macroinitiator was prepared from commercially available PSU by chloromethylation using SnCl4 and (CH3)3SiCl as catalyst and chloromethylation agent, respectively and paraformaldehyde. Successful chloromethylation was evidenced by the presence of the peak at δ 4.50 ppm in the 1H NMR spectrum (Figure ). The proportion of chloromethylated repeating units on PSU chains (degree of chloromethylation) was determined by the ratio of the integration values of the signals corresponding to the peak at 4.50 (f) to the peak at 7.76 (e) belonging to meta protons on the benzene ring near to the sulfonyl group. The degree of chloromethylation was determined to be 0.15.
In the second step, graft copolymers, PSU-g-POx were synthesized by LCROP of three different alkyl substituted Ox monomers, namely of MeOx, EtOx, and PrOx using carefully dried PSU–CH2Cl and KI as macroinitiator and activator, respectively, under suitable conditions. The polymerization was carried out at 120 °C in bulk. Throughout the polymerization, the solution remained homogeneous as the graft copolymers thus formed were soluble in the polymerization medium. Control experiments under identical experimental conditions in the absence of macroinitiator failed to produce any precipitable polymer. The results are summarized in Table . Although at lower rate, the polymerization was also started by benzylchloride functions and proceeded by a covalent mechanism since the nucleophilicity of the chloride anions was higher than that of oxazolines Citation[33,49,52]. The mechanism of the polymerization was converted to the ring-opening polymerization by the addition of KI as the iodides have lower nucleophlicity Citation[26,53]. Notably, compared to EtOx or PrOx, polymerization of MeOx was much slower due to the contribution of covalent mechanism even in the presence of KI.
Table 1. Synthesis of PSU-g-poly(2-alkyl-2-oxazoline)s by cationic ring-opening polymerization in bulk at 120 oC in the presence of KI (8 × 10−3 mol/L) using PSU-CH2Cl (M n = 29,000).
The 1H NMR spectra of the graft copolymers exhibited characteristic signals of both PSU backbone and POx side chains (Figure ). Typically, signals of methylene protons of the POx main chain appeared between 3.3 and 3.7 ppm. Notably, the chemical shifts of alkyl protons of the oxazoline repeating units appeared relatively up fields as stronger signals due to the relatively longer chains compared to the PSU backbone. It is also noted that no peak corresponding to the benzylchloride protons at 4.5 ppm was detected in the graft copolymer obtained after the cationic ring-opening polymerization, implying the formation of the initiating sites from the all chloromethyl groups. By comparing the intensity of the meta protons of PSU (e) at 7.83 ppm with that of the methylene protons of POx (k) at 3.5 ppm, the mol ratio of the segments was calculated. These results are also presented in the table and close to those obtained from the yield.
Successful grafting was further confirmed by FT-IR analysis of the precursor macroinitiator and graft copolymer (e.g. G4). In the spectrum of the graft copolymer, new bands at 1630 cm−1, and 1370 and 1470 cm−1 corresponding to amide carbonyl and methylene groups of POx, respectively are clearly detectable (Figure ).
Differential scanning calorimetry studies exhibited single T g values for the graft copolymers of PSU-g-PEtOx. This T g corresponds to glass transition of PEtOx and varied from 62.1 to 42.7 °C for G3 to G6, respectively. The bulk T g of PEtOx is 40–45 °C and of PSU is 185 °C. As PEtOx side chain length was increased from G3 to G6, T g of PEtOx in graft copolymers decreased from 62.1 °C towards the bulk T g value. This indicates a decreased mobility for shorter PEtOx side chains resulting from grafting to the rigid PSU backbone. As PEtOx side chain length increased, the effect of grafting on the mobility became less dominant and T g value approached to that of bulk PEtOx.
For further physicochemical investigations, we have spin-coated thin films of PSU-g-PAOx on silicon substrates and characterized the films by atomic force microscopy to understand the effect of alkyl side chains on the morphology of the graft copolymers. Neither of the graft copolymers was soluble in water at room temperature, but they formed initially homogenous dispersions indicating their amphiphilic nature. All graft copolymers dissolved in THF and formed homogeneous solutions. Figure shows the AFM height images of graft copolymers spin coated from 1 mg/ml solutions in THF. Film thicknesses were ∼8–12 nm as measured by ellipsometry.
Figure 3 AFM height images of graft copolymer films spin coated from 1 mg/ml solutions in THF. Film thicknesses were ∼10 nm. (a) G1, (b) G2, (c) G3, (d) G5, (e) G6, (f) G7, (g) G8.
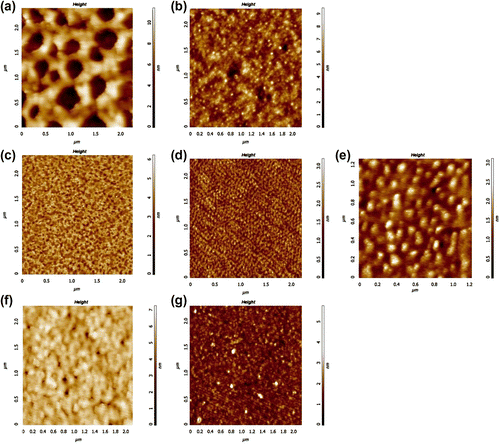
Films of PSU-g-PMeOx (Figures and 3(b)) did not cover the whole substrate surface and contained holes. The mesh-like morphology in Figure (G1, PMeOx/PSU mol ratio = 1.8) improved significantly with increasing mole fraction of PMeOx resulting in less and smaller holes in Figure (G2, PMeOx/PSU mol ratio = 7.6). Among the all graft copolymers, the ones containing PMeOx side chains (G1, G2) had the smallest POx/PSU mole ratio and the largest volume fraction of PSU to which the observed mesh-like morphology can be attributed.
Graft copolymers consisting of PEtOx and PPrOx (G3-G8) side chains all formed uniform films with significantly smaller surface roughness compared to PSU-g-PMeOx films. Figures show the morphology of PSU-g-PEtOx thin films having PEtOx/PSU mol ratio of 10.4 (G3), 24.5 (G5), and 68.1 (G6), respectively. Figure clearly shows a two-layered film. The incomplete top layer has an average thickness of ∼1.5 nm. The underlying layer is nearly complete with some holes having depth of ∼1.5 nm. A layer thickness of 1.5 nm is consistent with the degree of polymerization of the PEtOx side chains and this layered morphology shows the amphiphilic nature of the graft copolymers. Although the molecules are still amphiphilic at higher mole fractions of PEtOx, the volume fraction of PSU goes down to ∼0.06 at PEtOX/PSU mol ratio of 68.1. The larger volume fraction of PEtOx dominates and the top surface of the films becomes smoother. In the case of PPrOx side chains (G7, G8), the volume fraction of PSU was also less than 0.15 and the similar smooth surfaces were observed in Figures .
To check the presence of any layering for G5 and G6, even thinner films having incomplete surface coverage were spin coated and annealed at 80 °C for 3 h. The indication of any layering is possible to observe at the periphery of such isolated islands. The films were ∼5 nm thick on the average. Figure shows the AFM height image of G5 film after annealing. Two layers are clearly seen around the regions where the substrate was not covered: a ∼2 nm thick smooth film on the substrate and a ∼3 nm thick film on top. In case of G6, ∼5–6 nm thick islands were seen on the substrate (Figure ). Due to rather low volume fraction of PSU in G6, the layered morphology was not stable on the substrate. A different phase was evident at the perimeter of the islands in the form of slightly elevated circular regions in Figure which can be attributed to PSU.
Figure 4 AFM height images of spin coated G5 and G6 films after annealing at 80 oC for 3 h. (a) ∼5 nm thick G5 film and (b) ∼5 nm thick G6 film.
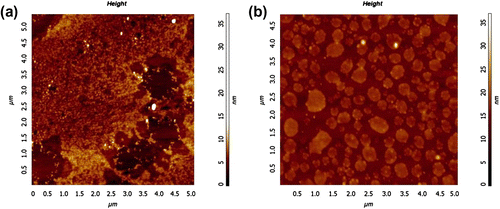
Water contact angle (CA) measurements were done on the films as a function of temperature to observe the thermoresponsive behavior of PEtOX side chains. The water CA was observed to increase with increasing temperature on PSU-g-PEtOx films as shown in Figure . At 22 °C, CA was ∼19o for G3, ∼13o for G5, and ∼11o for G6. This decrease in CA is expected as PEtOx mole fraction increased in the copolymers from G3 to G6. The CA increased gradually for G3 and G5 to ∼25o and 19o, respectively, as temperature increased to 80 °C. This increase is due to increased hydrophobicity of PEtOx with temperature.
On G6 films, the water CA increased from 10o to 11o between 22 and 50 °C and the increase was steeper starting from 60 °C. The cloud point temperature of aqueous PEtOx solutions is ∼60 °C Citation[54]. Therefore, we interpret the kink in CA data at 50 °C as the indication of thermoresponsiveness of PEtOx.
4 Conclusion
In conclusion, amphiphilic graft copolymers of PSUs with well-defined POx chains as lateral substituents, were synthesized by combination of chemical modification of PSU backbone and cationic ring-opening polymerization. The structure, morphology, and surface properties of such complexed macromolecules have been fully characterized. When the PSU volume fraction was ∼0.15 and above, the copolymer films showed layered morphology at the film/substrate interface indicating the amphiphilic nature of copolymers. The temperature dependent surface hydrophobicity of PEtOx films was also observed by water CA measurements. The successful combination of the properties of both backbone PSU and side chain POx at various mole ratios implies that these graft copolymers may find many applications as promising biomaterials in various areas.
Acknowledgment
The authors (Y.Y. and S.A.) thank Istanbul Technical University and DPT (Project no: 2005K120920) and the authors (P.T.G. and A.L.D.) thank TÜBİTAK (Project no: 109M542) for financial supports.
References
- Olabisi , O . 1997 . Handbook of thermoplastics , New York , NY : M. Dekker .
- Malchesky PS. In: Ratner BD, Hoffman AS, Schoen FJ, Lemons JE, editors. Biomaterials science: an introduction to materials in medicine. San Diego: Elsevier Academic Press; 2004. p. 514.
- Park , JY , Acar , MH , Akthakul , A , Kuhlman , W and Mayes , AM . 2006 . Polysulfone-graft-poly(ethylene glycol) graft copolymers for surface modification of polysulfone membranes . Biomaterials , 27 : 856 – 65 .
- Zornoza , B , Irusta , S , Tellez , C and Coronas , J . 2009 . Mesoporous silica sphere-polysulfone mixed matrix membranes for gas separation . Langmuir , 25 : 5903 – 9 .
- Lanese , DM , Alfrey , PS and Molitoris , BA . 1989 . Markedly increased clearance of vancomycin during hemodialysis using polysulfone dialyzers . Kidney International , 35 : 1409 – 12 .
- Susanto , H , Stahra , N and Ulbricht , MJ . 2009 . High performance polyethersulfone microfiltration membranes having high flux and stable hydrophilic property . Membrane Science , 342 : 153 – 64 .
- Unger , RE , Peters , K , Huang , Q , Funk , A , Paul , D and Kirkpatrick , CJ . 2005 . Vascularization and gene regulation of human endothelial cells growing on porous polyethersulfone (PES) hollow fiber membranes . Biomaterials , 26 : 3461 – 9 .
- Silva , AI and Mateus , MJ . 2009 . Development of a polysulfone hollow fiber vascular bio-artificial pancreas device for in vitro studies . Biotechnology , 139 : 236 – 49 .
- Yang , YS , Shi , ZQ and Holdcroft , S . 2004 . Synthesis of sulfonated polysulfone-block-PVDF copolymers: enhancement of proton conductivity in low ion exchange capacity membranes . Macromolecules , 37 : 1678 – 81 .
- Yilmaz , G , Toiserkani , H , Demirkol , DO , Sakarya , S , Timur , S , Yagci , Y and Torun , L . 2011 . Polysulfone based amphiphilic graft copolymers by click chemistry as bioinert membranes . Materials Science and Engineering: C , 31 : 1091 – 7 .
- Ates , S , Dizman , C , Aydogan , B , Kiskan , B , Torun , L and Yagci , Y . 2011 . Synthesis, characterization and thermally activated curing of polysulfones with benzoxazine end groups . Polymer , 52 : 1504 – 9 .
- Jouanneau , J , Mercier , R , Gonon , L and Gebel , G . 2007 . Synthesis of sulfonated polybenzimidazoles from functionalized monomers: preparation of ionic conducting membranes . Macromolecules , 40 : 983 – 90 .
- Stephen , R , Gibon , CM , Weber , M and Gaymans , RJ . 2009 . Synthesis and properties of poly(sulfone-arylate) copolymers . Journal of Polymer Science Part A: Polymer Chemistry , 47 : 3904 – 13 .
- Pospiech , D , Haubler , L , Meyer , E , Jehnichen , D , Janke , A , Böhme , F and Kricheldorf , HR . 1998 . Tailoring of polymer properties by the synthesis of multiblock copolymers . Designed Monomers Polymers , 1 : 103 – 9 .
- Dizman , C , Ates , S , Torun , L and Yagci , Y . 2010 . Synthesis, characterization and photoinduced curing of polysulfones with (meth)acrylate functionalities . Beilstein Journal of Organic Chemistry , 6 : 56 – 63 .
- Sangermano , M , Roppolo , I , Camara , VHA , Dizman , C , Ates , S , Torun , L and Yagci , Y . 2011 . Polysulfone/metal nanocomposites by simultaneous photoinduced crosslinking and redox reaction . Macromolecular Materials and Engineering , 296 : 820 – 5 .
- Dizman , C , Demirkol , DO , Ates , S , Torun , L , Sakarya , S , Timur , S and Yagci , Y . 2011 . Photochemically prepared polysulfone/poly(ethylene glycol) amphiphilic networks and their biomolecule adsorption properties . Colloids and Surfaces B: Biointerfaces , 88 : 265 – 70 .
- Dizman , C , Ates , S , Uyar , T , Tasdelen , MA , Torun , L and Yagci , Y . 2011 . Polysulfone/clay nanocomposites by in situ photoinduced crosslinking polymerization . Macromolecular Materials and Engineering , 296 : 1101 – 6 .
- Pospiech , D , Häubler , L , Eckstein , K , Komber , H , Voigt , D , Jehnichen , D , Meyer , E , Janke , A and Kricheldorf , HR . 1998 . Design of poly (ester imide)/polysulfone multiblock copolymers and their property profiles . Designed Monomers Polymers , 1 : 187 – 206 .
- Ulbricht , M and Belfort , G . 1996 . Surface modification of ultrafiltration membranes by low temperature plasma. II. Graft polymerization onto polyacrylonitrile and polysulfone . Journal of Membrane Science , 111 : 193 – 215 .
- Hickner , MA , Ghassemi , H , Kim , YS , Einsla , BR and McGrath , JE . 2004 . Alternative polymer systems for proton exchange membranes (PEMs) . Chemical Reviews , 104 : 4587 – 611 .
- Paul , M , Park , HB , Freeman , BD , Roy , A , McGrath , JE and Riffle , JS . 2008 . Synthesis and crosslinking of partially disulfonated poly(arylene ether sulfone) random copolymers as candidates for chlorine resistant reverse osmosis membranes . Polymer , 49 : 2243 – 52 .
- Bai , Z , Houtz , MD , Mirau , PA and Dang , TD . 2007 . Structures and properties of highly sulfonated poly(arylenethioethersulfone)s as proton exchange membranes . Polymer , 48 : 6598 – 604 .
- Levy , A and Litt , M . 1968 . Polymerization of cyclic iminoethers. V. 1,3-Oxazolines with hydroxy-, acetoxy-, and carboxymethyl-alkyl groups in the 2 position and their polymers . Journal of Polymer Science Part A: Polymer Chemistry , 6 : 1883 – 94 .
- Adams , N and Schubert , US . 2007 . Poly(2-oxazolines) in biological and biomedical application contexts . Advanced Drug Delivery Reviews , 59 : 1504 – 20 .
- Nuyken , O , Rueda-Sanchez , J and Voit , B . 1997 . Synthesis of graft copolymers by ring-opening polymerization of 2-nonyl- and 2-phenyl-2-oxazoline initiated by macroinitiators containing benzylchloride functions . Polymer Bulletin , 38 : 657 – 64 .
- Kagiya , T , Narisawa , S , Maeda , T and Fukui , K . 1966 . Ring-opening polymerization of 2-substituted 2-oxazolines . Journal of Polymer Science. Polymer Letters Edition , 4 : 441 – 5 .
- Saegusa , T and Kobayashi , S . 1986 . Cyclic imino ethers – polymerization chemistry and polymer characteristics . Makromolekulare Chemie, Macromolecular Symposia , 1 : 23 – 37 .
- Schlaad , H , Diehl , C , Gress , A , Meyer , M , Demirel , AL , Nur , Y and Bertin , A . 2010 . Poly(2-oxazoline)s as smart bioinspired polymers . Macromolecular Rapid Communications , 31 : 511 – 25 .
- Hoogenboom , R , Fitjen , MWM and Schubert , US . 2004 . Parallel kinetic investigation of 2-oxazoline polymerizations with different initiators as basis for designed copolymer synthesis . Journal of Polymer Science Part A: Polymer Chemistry , 42 : 1830 – 40 .
- Dworak , A . 1998 . The role of cationic and covalent active centers in the polymerization of 2-methyl-2-oxazoline initiated with benzyl bromide . Macromolecular Chemistry and Physics , 199 : 1843 – 9 .
- Aoi , K and Okada , M . 1996 . Polymerization of oxazolines . Progress in Polymer Science , 21 : 151 – 208 .
- Rueda-Sanchez , J , Zschoche , S , Komber , H , Schmaljohann , D and Voit , B . 2005 . Synthesis and characterization of thermoresponsive graft copolymers of NIPAAm and 2-alkyl-2-oxazolines by the ‘grafting from’ method . Macromolecules , 38 : 7330 – 6 .
- Hoogenboom , R , Fijten , MWM , Thijs , HML , van Lankvelt , BM and Schubert , US . 2005 . Microwave-assisted synthesis and properties of a series of poly(2-alkyl-2-oxazoline)s . Designed Monomers Polymers , 8 : 659 – 71 .
- Litt , M , Rahl , F and Roldan , LG . 1969 . Polymerization of cyclic imino ethers. VI. X-ray study of some polyaziridines . Journal Polymer Science. Polymer Physics Edition , 7 : 463 – 73 .
- Wiesbrock , F , Hoogenboom , R , Leenen , M , van Nispen , SFGM , van der Loop , M , Abeln , CH , van den Berg , AMJ and Schubert , US . 2005 . Microwave-assisted synthesis of a 42-membered library of diblock copoly(2-oxazoline)s and chain-extended homo poly(2-oxazoline)s and their thermal characterization . Macromolecules , 38 : 7957 – 66 .
- Park , JS , Akiyama , Y , Winnik , FM and Kataoka , K . 2004 . Versatile synthesis of end-functionalized thermosensitive poly(2-isopropyl-2-oxazolines) . Macromolecules , 37 : 6786 – 92 .
- Kobayashi , S and Uyama , H . 2001 . Polymerization of cyclic imino ethers: from its discovery to the present state of the art . Journal of Polymer Science Part A: Polymer Chemistry , 40 : 192 – 209 .
- Kobayashi , K , Igarashi , T , Moriuchi , Y and Saegusa , T . 1986 . Block copolymers from cyclic imino ethers: a new class of nonionic polymer surfactant . Macromolecules , 19 : 535 – 41 .
- Diab , C , Akiyama , Y , Kataoka , K and Winnik , FM . 2004 . Microcalorimetric study of the temperature-induced phase separation in aqueous solutions of poly(2-isopropyl-2-oxazolines) . Macromolecules , 37 : 2556 – 62 .
- Maeda , Y , Higuchi , T and Ikeda , I . 2000 . Change in hydration state during the coil-globule transition of aqueous solutions of poly(N-isopropylacrylamide) as evidenced by FTIR spectroscopy . Langmuir , 16 : 7503 – 09 .
- Maeda , Y , Nakamura , T and Ikeda , I . 2001 . Changes in the hydration states of poly(N-n-propyl methacrylamide) and poly(N-isopropyl methacrylamide) during their phase transitions in water observed by FTIR spectroscopy . Macromolecules , 34 : 8246 – 51 .
- Goddard , P , Hutchinson , LE , Brown , J and Brookman , LJ . 1989 . Soluble polymeric carriers for drug delivery. Part 2. Preparation and in vivo behavior of N-acylethylenimine copolymers . Journal of Controlled Release , 10 : 5 – 16 .
- Lin , P , Clash , C , Pearce , EM , Kwei , TK and Aponte , MA . 1988 . Solubility and miscibility of poly(ethyloxazoline) . Journal of Polymer Science Part B , 26 : 603 – 19 .
- Bloksma , MM , Paulus , RM , van Kuringen , HPC , van der Woerdt , F , Lambermont-Thijs , HML , Schubert , US and Hoogenboom , R . 2012 . Thermoresponsive poly(2-oxazine)s . Macromolecular Rapid Communications , 33 : 92 – 6 .
- Diehl , C , Dambowsky , I , Hoogenboom , R and Schlaad , H . 2011 . Self-assembly of poly(2-alkyl-2-oxazoline)s by crystallization in ethanol–water mixtures below the upper critical solution temperature . Macromolecular Rapid Communications , 32 : 1753 – 58 .
- Hoogenboom , R and Schlaad , H . 2011 . Bioinspired poly(2-oxazoline)s . Polymers , 3 : 467 – 88 .
- Liu , YL , Lin , GC and Wu , CS . 2008 . Preparation of polysulfone-g-poly(N-isopropylacrylamide) graft copolymers through atom transfer radical polymerization and formation of temperature-responsive nanoparticles . Journal of Polymer Science Part A: Polymer Chemistry , 46 : 4756 – 65 .
- Nuyken , O , Sanchez , JR and Voit , B . 1997 . Synthesis of amphiphilic graft copolymers by ring-opening polymerization of 2-methyl-2-oxazoline initiated by poly[isobutene-co-(p,m-chloromethylstyrene)] macroinitiators . Macromolecular Rapid Communications , 18 : 125 – 31 .
- Loontjens , T and Rique-Lurbert , L . 1999 . Synthesis of α-alkyl ω-trimethoxysilane polyoxazolines and their application as coatings on glass fibres . Designed Monomers Polymers , 2 : 219 – 29 .
- Avram , E , Butuc , E , Luca , C and Druta , I . 1997 . Polymers with pendant functional group. III. Polysulfones containing viologen group . Journal of Macromolecular Science A , 34 : 1701 – 14 .
- Rueda , J , Suica , R , Komber , H and Voit , B . 2003 . Synthesis of new polymethyloxazoline hydrogels by the ‘macroinitiator’ method. Macromol . Chemical Physics , 204 : 954 – 60 .
- Saegusa , T , Kobayashi , S and Yamada , A . 1976 . Kinetics and mechanism of the isomerization polymerization of 2-methyl-2-oxazoline by benzyl chloride and bromide initiators. Effect of halogen counteranions . Makromolekulare Chemie , 177 : 2271 – 83 .
- Tatar Güner , P , Miko , A , Schweinberger , F and Demirel , AL . 2012 . Self-assembled poly(2-ethyl-2-oxazoline) fibers in aqueous solutions . Polymer Chemistry , 3 : 322 – 24 .