Abstract
A terpolymer poly (vanillin-co- furfural-co-4-methylacetophenone) has been prepared by polycondensation technique in the presence of an acid catalyst. The structure of terpolymer has been elucidated on the basis of various physico-chemical techniques like FT-IR, 1H NMR, Pyrolysis (Py) (gas chromatography/mass spectroscopy, GC/MS), nitrogen adsorption/desorption analysis, gel permeation chromatography, thermogravimetry – derivative of thermogravimetry (TG-DTG), and differential scanning calorimetry. The apparent activation energies (E a) of each step (0.1 ⩽ α ⩽ 0.9) during thermal degradation have been determined using Flynn-Wall-Ozawa method. The degradation through random scission and unzipping mechanism is discussed based on the kinetics by nonisothermal kinetics by TG-DTG and products obtained by Py (GC/MS). The type of solid-state mechanism is three-dimensional diffusion.
Introduction
Considering our depleting fossil resources and the increasing emission of greenhouse gases, the utilization of renewable feed stocks is one necessary step towards synthesis of many useful products. The chemistry involved in the synthesis of biomass-based polymers is much more frequently associated with the modification of natural polymers (cellulose, starch, and chitin, etc.) or oligomers (lignin, tannins, and vegetable oils, etc.) rather than with the more classical fashion involving monomers and their polymerization Citation[1].
Furan resin systems have long been used as thermoset binders for aggregates in the foundry and refractory industries. The success of furan resins in these industries has been sustained by their high temperature resistance in such demanding applications. For example, furan resins are used in a variety of binder systems in the foundry industry, including ‘furan no-bake’ resins, heat-curing and gas-hardened systems. A number of resins have been synthesized by condensing cardanyl acrylate with furfural and selective organic compounds in the presence of acid as catalyst Citation[2].
Recently, ‘reworkable’ resins, which are thermosets have thermally or chemically degradable properties under a given condition. These reworkable resins have attracted much attention in terms of not only environmental aspects, i.e. recycling of the underlying materials, but also interesting application to nanosized patterned materials.
Among the reworkable resins, cleavable furan resins have been studied most extensively due to their high reactivity and wide variety of monomer structures. Renewable source-based monomers, such as furfural or furfuryl alcohol, can be prepared by hydrolysis and acid-dehydration conversion of polysaccharides, including hemicelluloses, cellulose abundant bagasse, and corncob Citation[3,4]. Furan resins have been made using phenol or formaldehyde by aldehyde condensation in the presence of an acid or alkali Citation[5,6]. Furfural-based resins are an important class of thermosets that have been widely used as high-performance materials in diverse applications such as high toughness and self-repairing ability, adhesives, matrices for fiber-reinforced composites, surface coatings, remendable polyurethane, and for electronic encapsulation Citation[7–15].
Enzymatic polymerization of phenolic monomers possessing electron-withdrawing aldehyde side-groups, protocatechualdehyde (PCA), and pyrogallolaldehyde (PGA) was reported Citation[16]. A new series of polyamides and co-polyamides were synthesized via reaction of a newly synthesized bipyridine containing diamine, 4,6-bis(4-aminophenyl)-2,2′-bipyridine, with commercial diacides Citation[17]. Polyester–glycidyl methacrylate (GMA)-based poly(high internal phase emulsion)s (polyHIPEs) with 85% internal phase were prepared by using unsaturated polyester resin (UPR), glycidyl metahacrylate, and divinylbenzene (DVB) or styrene (St) with triethanolamine (TEA) as an emulsifier in the presence of a porogen Citation[18]. The isoconversional method permits the effective activation energy of a process to be unambiguously estimated as a function of the extent of conversion. Our laboratories have been already reported microbial resistant terpolymers from renewable sources, because of its high mechanical and physical characteristics Citation[19,20]. Therefore, in our continuous efforts, we have synthesized a terpolymer from vanillin and 4-methylacetophenone with furfural as condensing agent in 1:1:2 M proportions by using hydrochloric acid as a catalyst. This terpolymer resin has extensively characterized by FT-IR, 1H NMR, Pyrolysis (Py) (gas chromatography/mass spectroscopy, GC/MS), gel permeation chromatography (GPC), nitrogen adsorption/desorption analysis, differential scanning calorimetry (DSC), and TG-DTG. The purpose of this study is to provide trustworthy kinetic data complemented by mechanistic interpretations. The thermal stability of the as-prepared VFUMA is higher than that of earlier reported VFUBA and VFUCA Citation[21].
Experimental
Materials
Vanillin (Ranbaxy, Mumbai, India), 4-chloroacetophenone (SRL, Mumbai, India), 4-methyl acetophenone (Merck, India), and furfuraldehyde AR grade (Merck, India) were used as received. All other chemicals, solvents, and indicators were of analytical grade procured from Thomas baker, Mumbai, India. Double distilled water was used for all experiments.
Synthesis of terpolymer (VFUMA)
A terpolymer resin was prepared by the condensation polymerization of vanillin (0.1 mol) and 4-methylacetophenone (0.1 mol) with furfuraldehyde (0.2 mol) in hydrochloric acid medium at 120 ± 2 °C in an oil bath for 8 h. The solid product obtained was immediately removed from the flask as soon as the reaction period was complete. Further, it was washed with cold water, dried, and powdered. The powder was repeatedly washed with hot water to remove excess of vanillin-co- furfuraldehyde (VFU) and 4-methylacetophenone-co-furfuraldehyde (4-MAFU) copolymers, which might be present along with the o-APMF copolymers. The dried resin was further purified by dissolving in 12% NaOH and regenerated in 1:1(v/v) HCl/water. The resulting terpolymer sample was washed with boiling water and dried in vacuum by using decicator room temperature. The purified terpolymer resin was finally ground well to pass through a 300 mesh size sieve and kept in a vacuum over silica gel. It is further characterized by GPC, which clearly indicates unimodal and broad peak with high polydispersity index (PDI) suggesting large fraction of terpolymer in resin mixture (Figure ). The yield of terpolymer is found to be 82%. The sieved resin was used for further characterization. The proposed structure of the synthesis of terpolymer VFUMA is shown in Scheme .
Characterization
The infrared (FT-IR) spectrum was recorded at the frequency range of 4000–400 cm−1 on Perkin-Elmer 2000 FT-IR spectrometer. 1H NMR (300 MHz) spectrum was recorded on Bruker DPX-300 spectrometer using DMSO-d6 as the solvent and TMS as internal standard. The qualitative and quantitative information of synthesized terpolymer was obtained from Py GC/MS, which consists of GC (Autosystem XL) and MS (Perkin Elmer Turbo mass). Sample of approximately 100 μg was analyzed. Sample preparation consisted of simply placing the sample into a quartz tube, which was introduced into the Pyroprobe automatically. No solvent is used, so there is no need for a solvent delay Citation[22].
Model Perkin-Elmer-2500 Pyrolyzer; oven: 300 °C; transfer line: 300 °C; pyrolysis temperature: 300 °C; pyrolysis time: 15 s; heating rate: 10 °C/ms.
The surface area of the terpolymer was determined by nitrogen adsorption/desorption analysis. The instrument utilized for nitrogen sorption analysis was a Micromeritics (Gemini 2370), USA Surface Area Analyzer. Before each experiment, the sample (0.2 g) was degassed at 50 °C for 24 h. The specific surface area of the dry terpolymer VFUMA was determined using the nitrogen sorption BET technique Citation[23].
The average molecular weight and its polydispersity index were determined with GPC analysis. It was performed with a setup consisting of a Waters 600 pumps and 2 ultra styragel columns (104, 500 Ao) with THF as the eluent at a flow rate of 1 mL/min equipped with Waters differential refractometer and calibrated with the standard linear polystyrene (PSt). The DSC curves were recorded with a Mettler Toledo DSC star system apparatus. A sample was heated from 30 to 250 °C with different heating rate of 20, 30, and 40 °C/min under nitrogen atmosphere. Thermogravimetric analysis (TG) was carried out using a Mettler Toledo Star System AG 2007 Module. The results were acquired with heating rates of 10, 20, 30, and 40 °C/min under nitrogen gas from 30 to 600 °C at constant flow rate of 80 mL/min.
Results and discussion
Spectral analysis
The structure of the terpolymer is elucidated and confirmed by FT-IR and 1H NMR spectra. As shown in Figure , the characteristic absorption band of phenolic hydroxyl group for both monomer vanillin and 4-hydroxyacetophenone appeared with numerous bands ranging 3450–3800 cm−1. A broadband appeared in the region 3448.5 cm−1 is assigned to the hydroxyl group of vanillin. The bands appeared at 1654.4–1667.7 cm−1 is due to –C–O stretching (formyl groups and acetyl groups of monomer and comonomer moieties) vibrations. A peak appeared at 1603.8 cm−1 may be assigned to C–H stretching of aromatic protons. Appearance of band at 1400 cm−1 may be ascribed to –CH–linkage present in the terpolymer resin. A signal observed at 1273 due to C–O stretching of the furan ring. The 1,2,3,5 tetra substitution of aromatic benzene ring by sharp, medium/weak absorption bands appeared between 1200 and 800 cm−1.
The 1H NMR spectrum of terpolymer VFUMA is depicted in Figure . The signals in the region at 7.6–8.1 (d) ppm may be assigned to the protons in the aromatic ring. The broad signal appeared at 3.4–3.6 ppm could be assigned to methine hydrogen atoms in the main chain of terpolymer. A highly deshielded aldehyde proton at 10.2 ppm was appeared with comparatively less signal intensity. A singlet observed in the region 2.4 ppm may be attributed to the protons in methyl protons of comonomer moiety. The furan ring (–O–CH=) is characterized with resonance signals at 7.4 ppm. An intense signal appeared at 4.3 ppm is attributed to –OCH3 protons of in the terpolymer.
Pyrolysis (GC/MS)
Py GC/MS is used to characterize materials by breaking down polymer chains with high temperature in an inert atmosphere and sending these resulting gases on line into a GC–MS system for identification and quantification Citation[19]. In this technique, a terpolymer sample is introduced as a solid so that no solvent peak is generated and all peaks in the chromatogram are the result of constituents of the sample material. The total ion chromatogram for pyrolysis of terpolymer VFUMA (pyrogram) is shown in Figure . It has shown four peaks in the program, which is due to oligomer furyl diphenyl methane (4.33), 2-vinylfuran (9.12), 1-(4-methylphenyl)-ethanone (13.52), and 1-(4-hydroxyphenyl)-ethanone (18.63). These results indicate that the pyrolysis mechanism may involve random scission by which it simply forms monomers and oligomers and also unzipping into monomers. The most abundant peak assigned for furyldiphenylmethane (oligomer), which elutes at about 4.33 min, is shown in Figure . Results from Py (GC–MS) also concluded that during thermal reaction, side groups elimination takes place and these side groups as a radicals further reacted with monomers to form some new compounds like 2-vinylfuran and 1-(4-hydroxyphenyl)-ethanone.
Specific surface area
A plot between relative pressure ranging from 0.05 to 0.30 bar to 1/[V A(P o/P − 1)] is depicted in Figure . The nitrogen adsorption properties of the microporous terpolymers in the solid state were obtained at 77 K with Brunauer-Emmett-Teller (BET) surface area of 0.5974 ± 0.0849 m2/g and total pore volume of 0.1372 cm3/g. Additionally, VFUMA is considered a ‘soft material’ with significant conformational motility of the VFUMA backbone. A contributing factor for the attenuated surface area may originate from the disordered and collapsed copolymer framework in the anhydrous state, and the reduced accessibility of the micropores in the terpolymer framework.
Molecular weight
The average molecular weight of the VFFMA terpolymer is determined by GPC. A unimodal gel permeation chromatogram is shown in Figure . The number average molecular weight () and weight average molecular weights (
) are 5415 and 17,544.6, respectively. The polydispersity index (
) is found to be 3.24.
DSC analysis
An amount of 6 mg of terpolymer VFUMA was put into a DSC sample pan and covered with an aluminum lid and closed tightly under pressure. DSC experiments were carried out over the temperature range from 30 to 250 °C at different scanning rates (Figure ). At scanning rate 20 °C min, the first endothermic peak at 102.4 °C is due to the evaporation of water which requires enthalpy of evaporation (ΔH e) value of 56.5 J/g and the second endothermic broad endothermic peak between 170.1 and 238.8 °C corresponds to melting zone. A peak temperature at 208.1 °C obtained from second endotherm is attributed to softening temperature of VFUMA, giving a ΔH m value of 22.6 J/g. As seen from DSC curves, the intensity and position of the endotherm gradually increases with increase in heating rates.
TG-DTG analysis
Thermogravimetry – derivative of thermogravimetry (TG-DTG) at different scanning rates (10, 20, 30, and 40 °C/min) of the terpolymer VFUMA is depicted in Figure , and the data are presented in Table which clearly suggest that VFUMA is more thermal stable as compared to earlier reported VFUCA and VFUBA. In halogen containing terpolymers (VFUBA and VFUCA), the carbon–halide bond is the weakest and hence, a halide-free radical is generated at relatively low temperatures, which is ultimately a reason for low thermal stability and also harmful for environmental problems. So, VFUMA is better choice than VFUCA and VFUBA.
Figure 6 TG-DTG thermograms of terpolymer VFUMA at different scanning rates (a) 10 °C min, (b) 20 °C min, (c) 30 °C min, and (d) 40 °C min.
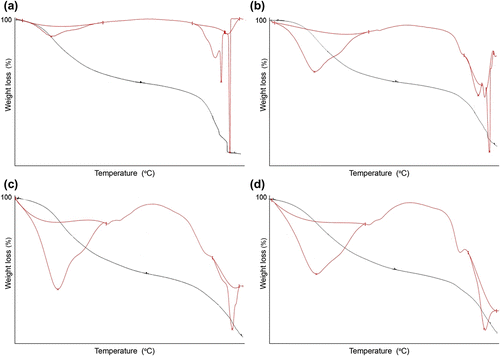
Table 1. TG-DTG data obtained from various heating rates for terpolymer VFUMA.
The analysis of the thermograms indicates that the decomposition of the terpolymers is a two-stage process after a loss of water molecule at 110 °C. In the first step, side chain attached to aromatic nucleus and hydroxyl, formyl, acetyl, methoxy, and methyl groups decompose. In the second step due to main chain scission, terpolymer simply unzips into monomers and also aromatic nucleus decompose and finally, there is no residue remained after completion of degradation. Decomposition is completed at about 600 °C in terpolymer.
Nonisothermal kinetic method
The application of TG-DTG methods holds great promise as a tool for unraveling the mechanisms of physical and chemical processes that occur during polymer degradation. In this study, Flynn-Wall-Ozawa (FWO) integral isoconversional method was used to analyze the nonisothermal kinetics of VFUMA.
The rate of solid-state nonisothermal decomposition reactions is expressed as
Rearranging Equation (1) and integrating both sides of the equation leads to the following expression:
Where,
FWO method Citation[24,25] is derived from the integral method. The technique assumes that A, f(α), and E are independent of T, while A and E are independent of α. Then Equation (2) may be integrated to give the following logarithmic form:
Using Doyle’s Citation[26] approximation for the integral, which allows for E/RT > 20, Equation (3) now can be simplified as
All TG curves show that the thermal decomposition takes places mainly in two stages, and the curves shift to the right-hand side with temperature.
Equation (4) has been used and the apparent activation energy of VFFHA can therefore be obtained from a plot of log β against 1000/T for a fixed degree of conversion, since the slop of such a line is given by −0.4567 E/RT. Figure illustrated the plots of log β against 1000/T at varying conversion in nitrogen. The values of E a at different conversion levels with linear correlation coefficients from 5 to 90% for VFUMA are given in Table . The linear correlation coefficients are all close to one, which means that the result is reliable. E a increases rapidly with the increase in conversion up to 5%, decreases moderately up to 70%, then gains to 90% conversion. Variations of E a values, therefore suggest a multistep reaction mechanism. Thermal decomposition of a polymeric material is a complex, heterogeneous process, consisting of several partial reactions. Basically two stages of decomposition of VFUMA terpolymer can be expected: the beginning of the thermal degradation has E a of about 2.7–13.9, which may be attributed to the side groups (methyl, acetyl, formyl, hydroxyl, and methoxy) elimination of weak links in the polymer chains, whereby the last step has E a of above 35.6 kJ/mol that can be associated with random scission reactions that lead to the formation of low molecular weight products.
Table 2. E a values of the thermal decomposition for a VFUMA from FWO expression.
Thermodegradation mechanism by Criado method
Thermodegradation mechanism was established by using Criado method Citation[27]. In order to find out the reaction mechanism, we have employed reference theoretical master curves. A master plot is a characteristic curve independent of the condition of the measurement. Knowing previously the activation energy by some other method, (in this case with the FWO method), where it is not necessary to know the reaction mechanism, and defining the function:
where π(x) is an approximate expression obtained by integration against temperature, which cannot be expressed by simple analysis formula. A reasonable relationship between π(x) and P(x) was proposed as shown in Equation (5),
The fourth rational expression of P(x)
when x > 20, the error of Equation (7) is less than 10−5%.
Combining Equations (2), (5), and (6), we obtain:
From Equations (3), (6), and (7), the following relationship can be derived:
The Z(α) − α master curves can be plotted using Equation (8) according to different reaction mechanism shown in Table . The experimental data at 10 °C min obtained by FWO method (Table ) were substituted into Equation (9). Figure shows the Z(α) − α master and experimental curve of the terpolymer VFUMA. The results for VFUMA show that the experimental curves of two steps of degradation belong to three-dimensional diffusion (D 3) reaction mechanism (three-dimensional diffusion; Jander Equation).
Table 3. Algebraic expressions for g(α) and f(α) for the most frequently used mechanisms of solid-state processes.
Conclusion
A terpolymer VFUMA based on the condensation reaction of vanillin and 4-methylacetophenone with furfural in the presence of acid catalyst was prepared. The structure of the terpolymer resin was proposed by spectral analyses. Py (GC–MS) and TG-DTG analysis suggest degradation mechanism was supposed to side group elimination at early stage and random chain scission at last. The mean activation energy of 17.35 k J/mol was determined by nonisothermal degradation kinetics. DSC scans at different heating rates was clearly demonstrated two-stage endothermic transition, which was probably due to expulsion of water and melting, respectively. The thermal decomposition model for the terpolymer was proposed as D3 using Craido method. Its ease of synthesis opens up routes for large volume applications in the mass transport, automotive, and construction industries. This newly developed environmental friendly terpolymeric furan resin could be an exciting intermediates material for the construction of 3D-designed structural products.
Acknowledgments
Authors are thankful to Mr. Ram Chandra Meghwal for artwork, Udaipur, HASETRI, JK Tyre, Rajsamand, SICART, Vallabh vidyanagar and CDRI, Lucknow for analytical and spectral data of terpolymer.
References
- Weissermel , K and Arpe , H . 2003 . Journal of Industrial and Organic Chemistry , Rev. 4th ed , 367 Weinheim : Wiley-VCH .
- Guru , BN , Das , TK and Lenka , S . 1999 . Polymers from renewable resources. XXVII. Studies on synthesis, characterization, and thermal properties of resins derived from cardanyl acrylate-furfural-organic compounds . Polymer-Plastic Technology , 38 : 179 – 187 .
- Palmieri , F , Adams , J , Long , B , Heath , W , Tsiartas , P and Willson , CG . 2007 . Design of reversible cross-linkers for step and flash imprint lithography imprint resists . ACS Nano , 1 : 307 – 312 .
- Corma , A , Iborra , S and Velty , A . 2007 . Chemical routes for the transformation of biomass into chemicals . Chemical Reviews , 107 : 241 – 250 .
- Dunlop , AP . 1948 . Furfural formation and behaviour . Industrial & Engineering Chemistry Research , 40 : 204 – 209 .
- Gandini , A . 2008 . Polymers from renewable resources: a challenge for the future of macromolecular materials . Macromolecules , 41 : 9491 – 9504 .
- Gandini , A and Belgacem , MN . 1997 . Furans in polymer chemistry . Progress in Polymer Science , 22 : 1203 – 1379 .
- Liu , YL and Chen , YW . 2007 . Thermally reversible cross-linked polyamides with high toughness and self-repairing ability from maleimide- and furan-functionalized aromatic polyamides . Macromolecular Chemistry and Physics , 208 : 224 – 232 .
- Gaina , V , Ursache , O , Gaina , C and Buruiana , E . 2012 . New thermally-reversible epoxy-urethane networks . Designed Monomers and Polymers , 15 : 63 – 73 .
- Sarıbıyık , OY and Serin , S . 2011 . Synthesis, characterization and catalytic investigation of novel polymeric thiourea . Designed Monomers and Polymers , 14 : 133 – 142 .
- Patel , HS and Patel , JA . 2008 . Synthesis, characterization and glass reinforcement of poly(amido-imide)’s Part-3 . Polymer-Plastics Technology and Engineering , 48 : 102 – 109 .
- Patel , MN , Patel , JR and Patel , H . 1998 . Physico-chemical studies of a furfural copolymer and its polychelates . Journal of Macromolecular Science: Pure and Applied Chemistry , 25 : 211 – 218 .
- Moschiar , SM , Galego , N and Vazquez , A . 1995 . Composites of furan cured with acid catalyst . Polymer-Plastics Technology and Engineering , 34 : 65 – 78 .
- Metwally , MS and Metwally , NS . 1992 . Synthesis and properties of synthetic Egyptian-corncob-phenol-formaldehyde cationic exchanger . Polymer-Plastics Technology and Engineering , 31 : 773 – 785 .
- Bilicia , A , Kayab , I and Yıldırımb , M . 2011 . Peroxidase-catalyzed synthesis of polyphenols bearing aldehyde units . Designed Monomers and Polymers , 14 : 353 – 366 .
- Koohmareh , GA and Souri , Z . 2011 . Synthesis and characterization of some new thermally stable crystalline polyamides and co-polyamides bearing bipyridine side-chain groups . Designed Monomers Polymers , 14 : 475 – 485 .
- Mert , EH , Kaya , MA and Yıldırım , H . 2012 . Preparation and characterization of polyester–glycidyl methacrylate poly HIPE monoliths to use in heavy metal removal . Designed Monomers and Polymers , 15 : 113 – 126 .
- Haroun , AA , Taleb , EMA and Ab-El-Ghaffer , MA . 2010 . Synthesis and characterization of novel thermoplastic films for removal of heavy metal ions . Polymer-Plastics Technology and Engineering , 49 : 454 – 461 .
- Chauhan , NPS , Ameta , R and Ameta , SC . 2011 . Synthesis, characterization and thermaldegradation of substituted acetophenone based terpolymers having biological activities . Journal of Macromolecular Science: Pure and Applied Chemistry , 48 : 482 – 492 .
- Chauhan NPS. Terpolymerization of p-acetylpyridine oxime, p-methylacetophenone and formaldehyde, and its thermal studies. Journal of Thermal Analysis and Calorimetry. 2011. In press.
- Chauhan , NPS , Kataria , P , Chaudhary , J and Ameta , SC . 2012 . Synthesis, characterization, and thermal studies of terpolymers derived from vanillin, furfural, and halo- substituted acetophenones . International Journal of Polymeric Materials , 61 : 57 – 71 .
- Chauhan , NPS and Ameta , SC . 2011 . Preparation and thermal studies of self-crosslinked terpolymer derived from 4-acetylpyridine oxime, formaldehyde and acetophenone . Polymer Degradation and Stability , 96 : 1420 – 1429 .
- Chauhan , NPS , Ameta , R and Ameta , SC . 2011 . Synthesis, characterization, and thermal degradation of p-chloroacetophenone oxime based polymers having biological activities . Journal of Applied Polymer Science , 122 : 573 – 585 .
- Ozawa , T . 1965 . A new method of analyzing thermogravimetric data . Bulletin of Chemical Society of Japan , 38 : 1881 – 1886 .
- Flynn , JH and Wall , LA . 1966 . A quick, direct method for the determination of activation energy from thermogravimetric data . Journal of Polymer Science Part B: Polymer Letters , 4 : 323 – 328 .
- Doyle , CD . 1964 . Integral methods of kinetic analysis of thermogravimetric data . Makromolekulare Chemie , 80 : 220 – 224 .
- Craido , JM , Malek , J and Ortega , A . 1989 . Applicability of the master plots in kinetic analysis of a non-isothermal rate . Thermochimica Acta , 147 : 377 – 385 .