Abstract
Poly(diethyleneglycol 1,1′-ferrocene dicarboxylate) (PDEFD) was synthesized by solution polycondensation reaction of diethylene glycol with 1,1′-ferrocenyl chloride. The synthesized polymer was characterized via the measurement of its 1H NMR spectrum, UV–vis spectrum, and FTIR spectrum. X-ray diffraction pattern was measured to investigate the crystallinity of the synthesized polymer and it was found that the polymer was mostly amorphous. The molecular weight of the polymer was determined by gel permeation chromatography. In addition, the electrochemical properties of the synthesized polymer were examined and the influence of many parameters, including the solvent, the scan rate, and the electrolyte concentration was studied. The electrode processes were found to be controlled by both electrode reaction and the mass diffusion. In addition, the electrochemical processes of PDEFD in dimethyl sulfoxide (DMSO) were confirmed neither to be totally reversible nor completely irreversible. Moreover, the thermogravimetric analysis and differential thermal analysis were employed to examine the thermal properties of the polymer. Finally, the liquid crystalline properties of the synthesized polymer were investigated via the polarizing optical microscope technique.
Notice of correction
This article was originally published with errors. This version has been corrected. Please see Corrigendum (dx.doi.org/10.1080/15685551.2012.743700) for further information.
1 Introduction
The introduction of metal atoms in the structure of polymers is a promising method to prepare new processable materials due to their unusual physical and chemical properties that are different from the original ones and thus these new materials can be used in wide applications in many areas Citation[1,2]. In addition, the presence of metal atom imparts the polymer chains new physical and chemical properties including redox, electronic, optical, and magnetic properties Citation[3,4]. There are three arrangements of the metal atom relative to the polymer backbone Citation[5]. In the first type, the metal atom is attached to the polymer with a linker Citation[6]. In the second type, the metal atom is attached electronically to the polymer i.e. the metal and the polymer backbone are electronically coupled and can affect each others’ properties Citation[7]. In the third type, the metal atom is located in the backbone itself Citation[8].
Ferrocene is an aromatic double decker organometallic compound that contains two cyclopentadienyl rings which sandwich an iron atom Citation[9–12]. In addition, ferrocene, its derivatives, complexes, and polymers are hot areas of research from its discovery till now. Furthermore, ferrocene is characterized by its good redox properties, thermal, and photochemical stability Citation[13–16] and thus a huge number of ferrocene-based polymers have been synthesized.
Ferrocene-based polymers have wide applications in different fields like optical display technologies Citation[17], amperometric biosensors Citation[18,19], DNA and protein sensors Citation[20,21], carbon monoxide sensors, Citation[22] and in the construction of optical logic gates Citation[23]. Additionally, ferrocene-based photocross-linkable redox polymers have been synthesized for the design of enzyme-based sensors and fuel cells Citation[24]. Some ferrocene-based polymers have exhibited excellent charge/discharge properties which assume their use as a new class of cathode active and charge storage materials for rechargeable batteries Citation[25]. Recently, linear poly(ethylenimine) has been modified with ferrocene groups for the use as an anodic mediator in glucose/O2 biofuel cells Citation[26]. Moreover, ferrocene has been used in the end decoration of a homopolymer for the construction of voltage-responsive vesicles Citation[27].
Many ferrocene-based polyesters were synthesized few decades ago Citation[28–31]. Since ferrocene-based polymers hold tremendous potential applications in many areas, a detailed investigation of their properties is highly required. Despite the aforementioned study on the synthesis of poly(diethyleneglycol 1,1′-ferrocene dicarboxylate) (PDEFD) Citation[32], a detailed investigation concerning the characterization of the polymer, its electrochemical, thermal, and liquid crystalline properties is lacking in the literature. On the whole, the current study provides the synthesis of PDEFD using solution polycondensation method. Afterwards, the polymer was characterized via the measurement of its 1H NMR spectrum, FTIR spectrum, UV–vis spectrum, and molecular weight. Moreover, the electrochemical, the thermal, and the liquid crystalline properties of PDEFD were investigated.
2 Experimental
2.1 Materials
The reagent 1,1′-ferrocene dicarboxylic acid (Shanghai Chemical reagent Co.) was purified via dissolution in sodium hydroxide solution, filtered, precipitated with hydrochloric acid and dried under vacuum before use.Citation[33]. Oxalyl chloride (Sinopharm Chemical Reagent Co., Ltd) was used without further purification. Pyridine (Shanghai Lingfeng Chemical Reagent Co., Ltd) was distilled in the presence of molecular sieves. Triethyl amine (TEA) was used after distillation over CaH2. Diethylene glycol was purchased from Alfa Aesar and it was used as received. Dichloromethane (CH2Cl2, AR) was distilled twice in the presence of CaH2. Tetrahydrofuran (THF, AR) was distilled twice in the presence of potassium. The Bu4NBF4 (AR) was directly used.
2.2 Synthesis of 1,1′-ferrocenedicarbonyl chloride
A solution of 1,1′-ferrocenedicarbonyl chloride was prepared from 1,1′-ferrocenedicarboxylic acid according to the method described by Knobloch and Rauscher Citation[33] using oxalyl chloride as a chlorinating agent in CH2Cl2 and employing pyridine as a catalyst. In typical procedures, 3.16 g (11.53 mmol) of 1,1′-ferrocenedicarboxylic acid was dissolved in 48.7 mL of freshly distilled CH2Cl2 and stirred well under Ar atmosphere. A 4.95 mL (57.65 mmol) of oxalyl chloride (the molar ratio of oxalyl chloride to the diacid is 5) and three drops of pyridine were added to the previous solution. The solution was stirred in the dark for 12 h at room temperature and then allowed to reflux for 6 h. The contents of the reaction flask were evaporated to dryness under reduced pressure and the residue was extracted with petroleum ether at 80 °C. The product was kept under Ar atmosphere for the next experiment.
2.3 Synthesis of PDEFD
PDEFD was synthesized by solution polycondensation reaction of diethylene glycol with 1,1′-ferrocenedicarbonyl chloride according to the reported method Citation[34]. In typical procedures, 1.5745 g (5.06 mmol) of 1,1′-ferrocenedicarbonyl chloride was dissolved in 10.6 mL freshly distilled THF. Afterwards, a solution of 0.64 mL (6.733 mmol) diethylene glycol in 10.5 mL THF and 1.4 mL (0.01 mol) of TEA were added (the molar ratio of TEA: diethylene glycol: 1,1′-ferrocenedicarbonyl chloride is 2:1.33:1). The solution was then stirred for 16 h at 10 °C and filtered. PDEFD was finally obtained via precipitation of the filtrate in ethanol.
2.4 Instruments
1H NMR spectrometry was performed using a 500 MHz AVANCE NMR spectrometer (Model DMX400). The chemical shifts were relative to tetramethylsilane at δ = 0 ppm. Gel permeation chromatography (GPC) analysis was carried out with a Waters 1515 GPC instrument and monitored by thermal conductivity detector. The mobile phase was THF eluent at a flow rate of 1.0 mL/ min, and the samples were calibrated against polystyrene at 30 °C.
The cyclic voltammetry (CV) measurements were carried out with a CHI-630 A electrochemical analyzer (CH Instruments, Inc., Austin, Texas) in an undivided three-electrode cell. All electrodes were from CH Instruments. The working electrode is a Teflon-shrouded platinum disk electrode with a diameter of 2 mm for the solution test. The working electrode was polished to a mirror finish with 0.05 μm Al2O3 paste on felt, cleaned by ultrasonication successively in 0.1 M NaOH, 1:1 HNO3, anhydrous ethyl alcohol, and double distilled water, then dried and used for the electrochemical measurements. A platinum wire electrode was used as the counter electrode. The reference electrode is an Ag wire electrode for solution test. Every CV experiment was done three times to get an average result and in every experiment, the electrolyte is Bu4NBF4 (0.1 M), the scan rate is 0.1 V/s, and the concentration of the polymer is 0.5 mM, except for the specially indicated ones.
UV–vis spectra were carried out using 3802 UV/VIS unico spectrophotometer. Fourier transform infrared (FT-IR) spectra were measured within the 400–4000 cm−1 region on a Jasco IR-700 infrared spectrophotometer with the KBr pellet technique. Powder X-ray diffraction (XRD) pattern was obtained with a D/max-3BX diffractometer using Cu Kα radiation (40 kV, 80 mA). The diffraction pattern for structure determination was measured using a Siemens D5000 powder diffractometer with monochromatic CuKα1 radiation and position sensitive detector.
Thermogravimetric analysis (TGA) was performed with a Perkin-Elmer Pyris 1 thermogravimetric instrument using a heating rate of 10 °C/min in a nitrogen flow. The differential scanning calorimetry (DSC) thermogram was measured using differential scanning calorimeter (Model TAQ 200) under nitrogen atmosphere with the sample placed in closed lid aluminum pans and a heating and a cooling rate of 10 °C/min were implemented.
The polarizing microscopic studies were performed using a Nikon Eclipse E 600 POL polarizing optical microscope (POM). The film sample was prepared by direct casting solution of the polymer on a clean cover glass to obtain uniform film and a rate of 10 °C/min were implemented for heating and cooling processes.
3 Results and discussion
3.1 Synthesis and characterization of PDEFD
A solution of 1,1′-ferrocenedicarbonyl chloride was prepared from 1,1′-ferrocenedicarboxylic acid using oxalyl chloride as a chlorinating agent in CH2Cl2 employing pyridine as a catalyst. PDEFD was then synthesized via the solution polycondensation reaction of diethylene glycol with 1,1′-ferrocenedicarbonyl chloride using THF as a solvent and TEA as a proton acceptor. The reaction pathway is given in Scheme .
The structure of PDEFD was elucidated by conventional methods including 1H NMR, UV–vis, and FTIR spectra. The 1H NMR spectrum of PDEFD is shown in Figure . It shows peaks with the following shifts (δ, ppm): 3.28–4.03 (–OCH2– and –CH2–), 4.08–4.69, and 4.71–5.03 (–C5H4–). This spectrum indicates that PDEFD was synthesized successfully. PDEFD was found to possess high molecular weight as compared to the data presented in the literature Citation[35]. GPC represented the molecular weight of the polymer to be 11,587, the poly dispersity index to be 3.8 and the GPC curve is shown in Figure . In addition, the UV–vis spectrum of PDEFD in dimethyl sulfoxide (DMSO) is shown in Figure . The absorption peak at 264 nm is characteristic to the ester group Citation[36].
The polycondensation reaction and the chain structure of PDEFD were confirmed via the measurement of FTIR. The FTIR spectrum of the synthesized polymer is shown in Figure . The existence of the band at 3100 cm−1 is associated with the existence of C–H in the ferrocenyl group. The aliphatic C–H stretching vibration appears at 2963 cm−1. In addition, the band at 1715 is assigned to the stretching vibration of the carbonyl (C=O) group. The bands at 1261 and 1096 cm−1 are attributed to C–O–C stretching vibration and C–O stretching vibration, respectively. Furthermore, the aromatic C–H bending vibration and Fe-Cp stretching vibration appear at 802 and 492 cm−1, respectively. The FTIR spectrum shows the characteristic peaks of a ferrocene-based polyester and the spectrum coincides with the literature Citation[2,37–43].
The crystallinity of PDEFD was investigated via the measurement of XRD in the region of 2θ = 5–70 ° at room temperature. As shown in Figure , the X-ray diffractography of PDEFD exhibits only one broad peak at 2θ = 11.9 ° indicating that PDEFD is mostly amorphous. This amorphous nature of the polymer is reflected in its good solubility in THF, DMF, and DMSO. On the other hand, PDEFD has a very low solubility in other solvents such as CHCl3 and the solvents with low polarity like CH2Cl2.
3.2 Electrochemical properties
The electrochemical behavior of ferrocene compounds and ferrocene-based polymers was found to be affected by many factors including the type and the concentration of the supporting electrolyte, the potential scan rate, and also the polarity of the solvent Citation[34,36,44–46].
3.2.1 Influence of solvents on the electrochemical behavior of PDEFD
PDEFD has a special dissolubility as it can dissolve in THF, DMF, and DMSO but it has a poor solubility in solvents with low polarity. Therefore, THF, DMF, and DMSO were employed as solvents to investigate the electrochemical behavior of PDEFD. The typical cyclic voltammogram of PDEFD in the previously mentioned solvents is represented in Figure .
Figure 4 CV curves of PDEFD at [Fe] = 0.5 mM (a) in different solvents; supporting electrolyte: 0.1 M Bu4NBF4, scan rate: 0.1 V/s, (b) at different scan rates in DMSO; supporting electrolyte: 0.1 M Bu4NBF4, and (c) at different concentrations of the electrolyte in DMSO, scan rate: 0.1 V/s.
![Figure 4 CV curves of PDEFD at [Fe] = 0.5 mM (a) in different solvents; supporting electrolyte: 0.1 M Bu4NBF4, scan rate: 0.1 V/s, (b) at different scan rates in DMSO; supporting electrolyte: 0.1 M Bu4NBF4, and (c) at different concentrations of the electrolyte in DMSO, scan rate: 0.1 V/s.](/cms/asset/3263ae40-be83-42e8-aa1b-23691789c195/tdmp_a_705504_o_f0004g.jpg)
The organic solvent affects the shape of the CV largely because the shape of the CV of PDEFD was found to be deformed on decreasing the polarity of the solvents. In DMSO, PDEFD exhibited a pair of well-defined redox peaks with E pa and E pc values of 0.461 and 0.306 V vs. Ag/Ag+. This indicates the presence of only one kind of electroactive centers in the polymer which corresponds to its structure. In addition, the presence of only one pair of well-defined redox peaks indicates the coplanar structure which makes the polymer tightly contacted with the surface of the electrode Citation[47]. The shape of the CV of PDEFD was deformed in DMF and THF.
3.2.2 Influence of the potential scan rate on the electrochemical behavior of PDEFD
The typical cyclic voltammogram of PDEFD at different potential scan rates of 0.1, 0.2, 0.3, 0.4, and 0.5 V/s in DMSO, is shown in Figure and the electrochemical data are shown in Table .
Table 1. Electrochemical data of PDEFD at different scan rates.
It was found that the wave shape of PDEFD is sensitive to the scan rate. Additionally, the peak current values of PDEFD solution, i pa and i pc, increase with increasing the scan rate. Furthermore, the rate of increasing the reduction peak current (i pc) is higher than the rate of increasing the oxidation peak current (i pa). According to the electrochemical theory Citation[48], these results indicate that at room temperature the charge transport of the electrode processes follows Fick’s law and also the electrode processes are diffusion controllable over the detected scan rate range.
Although the electrode processes of PDEFD solution are diffusion controllable, the rates of electron transport between PDEFD and electrode, and the electrode reaction are slow. The electrode reaction can occur completely and the electrochemical behaviors of PDEFD exhibit nearly reversible characteristics at slow scan rates due to the slow rates of electron transport. On the other hand, the electrode reaction cannot reach completion on time upon scanning at high scan rates, and hence the CVs exhibit quasi-reversible or irreversible characteristics, and the electrode processes are controlled by both electrode reaction and the mass diffusion Citation[48–50].
In addition, the peak potential (E p) in reversible electrode processes is independent on the scan rate and ΔE p is less than 60 mV Citation[48], while the peak potential in irreversible electrode processes has a linear relationship with the logarithm of the scan rates (ν 1/2) and ΔE p is more than 60 mV. The experimental results indicate that the electrochemical processes of PDEFD solution are relatively complicated and thus it can be concluded that the electrochemical processes of PDEFD solution are neither reversible nor totally irreversible.
3.2.3 Influence of electrolyte concentration on the electrochemical behavior of PDEFD
The typical cyclic voltammogram of PDEFD at different concentrations of the electrolyte (0.01, 0.025, 0.05, 0.075, and 0.1 M) in DMSO is represented in Figure and the electrochemical data are shown in Table .
Table 2. Electrochemical data of PDEFD at different concentrations of the electrolyte.
From Figure and Table , it was found that both anodic peak current i pa and the cathodic peak current i pa increases with increasing the concentration of the electrolyte. This can be attributed to the resistance decrease of the polymer solution with increasing the concentration of the electrolyte which results in a fast transport of the electroactive substances and the electrode reaction becomes easy. As mentioned above, the peak-to-peak potential separation ΔE P and the ratio of peak current i pa/i pc can indicate the reversibility of the electrode processes Citation[48]. Figure and Table show that the ratio of the peak current i pa/i pc increases (approached to 1 gradually) with increasing the concentration of the electrolyte. However, no regular behavior was found between the concentration of the electrolyte and the peak-to-peak potential separation ΔE P. The previous findings assure that the electrochemical processes of PDEFD in DMSO are neither totally irreversible nor completely reversible.
Table shows the relationships among the peak currents, the peak potentials, and the scan rates for PDEFD at different concentrations of the electrolyte. Most of those relations were obtained with a good correlation coefficient. Additionally, the peak current values of PDEFD solution, i pa and i pc, have a linear relationship with the square root of the scan rate (ν 1/2). The results of the linear correlation between i p (i pa and i pc) and ν 1/2 are listed in Table .
Table 3. Relationships among the peak currents, the peak potentials, and the scan rates for PDEFD at different concentrations of the electrolyte.
3.3 Thermal and liquid crystalline properties of PDEFD
The thermal properties of PDEFD were examined by TGA and differential thermal analysis (DTA) under nitrogen. The curves of TGA and DTA are shown in Figure . The initial temperature at which cumulative weight change has a magnitude that can be detected by the thermobalance known as T in Citation[41], the temperature of 5% weight loss (T 5), the temperature of 10% weight loss (T 10), the temperature at the end of the curve (T f), the temperature of maximum weight loss (T s), and the char yield are mentioned in Table .
Table 4. Thermal properties of PDEFD.
Furthermore, it can be observed that the weight loss is a multistage process that corresponds to three peaks in the DTA curve which is in accordance with the literature Citation[36,44]. The first stage of weight loss appeared at 320 °C and it is assigned to the breakage of the ester bonds (C=O and C–O) connected to the ferrocene units producing a large amount of carbon dioxide which is responsible for this weight loss. The Fe–C bonds in the ferrocene units is broken up to 406 °C forming a lot of Fe atoms which constitute the second stage of weight loss. These Fe atoms have a good catalytic effect and catalyze the degradation of the polymer. The dramatic weight loss in the third stage represented the degradation of the ferrocene units in the polymer. The weight loss stopped after 600 °C and the main components of the residue (29%) are carbon and iron. The DSC thermogram of PDEFD, with a heating and a cooling rate of 10 °C/min was measured. T g and T m of 42.09 and 69.43 °C were detected, respectively.
Many ferrocene-based compounds and polymers exhibited thermotropic liquid crystalline properties. To investigate the liquid crystalline behavior of PDEFD, POM micrographs were measured with a heating and cooling rate of 10 °C/min. The liquid crystalline micrographs of the polymer, during heating and cooling, are shown in Figure . The synthesized polymer presented liquid crystalline properties with characteristic textures. The mesophase type was estimated by visual comparison (under the microscope) with the phases reported previously in the literature Citation[17,51–54]. PDEFD possessed a nematic phase during heating and the polymer exhibited a texture with schlieren desclinations as shown in Figure . On cooling, the polymer sample exhibited the same texture as shown in (Figure ). Additionally, the synthesized polymer possessed high phase duration (ΔT) and the clearing point is above the decomposition temperature of the polymer and thus no isotropization process was observed for the liquid crystalline phase of PDEFD. Similar behaviors were observed by many authors Citation[55,56].
Figure 6 POM micrographs of the texture displayed by PDEFD (a) at 152 °C and (b) 9.2 °C (magnification × 100).
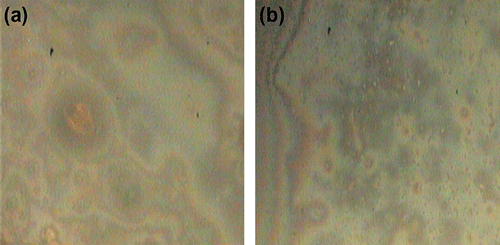
As can be seen from the chemical structure of PDEFD, it does not contain any mesogenic group in the main chain of the polymer; nevertheless, it exhibited crystalline order and melting birefringence in POM. These findings assure the previously obtained conclusion by Singh et al. which states that the presence of the ferrocene units in the polymers contributed to their liquid crystallinity and also the ferrocene units were mesogenic Citation[2,57].
4 Conclusion
Liquid crystalline PDEFD was prepared successfully by the solution polycondensation reaction of diethylene glycol with 1,1′-ferrocenyl chloride. PDEFD was characterized via the measurement of its 1H NMR spectrum, UV–vis spectrum, and FTIR spectrum. The XRD measurement shows that PDEFD is mostly amorphous. The electrochemical behavior of PDEFD was investigated and the influence of many parameters, including the solvent, the scan rate, and the electrolyte concentration was studied. The cyclic voltammograms of PDEFD indicate its coplanar structure which makes the polymer tightly contacted with the surface of the electrode. Furthermore, the electrode processes were found to be controlled by both electrode reaction and the mass diffusion. In addition, the electrochemical processes of PDEFD in DMSO were confirmed neither to be totally reversible nor completely irreversible. Moreover, the measurement of TGA and DTA of PDEFD indicated its relative thermal stability. Finally, the liquid crystalline properties of the polymer were assigned via POM technique. PDEFD possessed nematic phases during heating and cooling and it exhibited textures with schlieren disclinations. The ordered structures were permanently retained in the cooling and the heating scans. The presence of the ferrocene units in the polymer contributed to its liquid crystallinity and also the ferrocene units were mesogenic.
Acknowledgments
Financial supports from the National Natural Science Foundation of China (21072715 and 20802067), the International Science and Technology Cooperation Project of Ministry of Science and Technology of China (2009DFR40640), the Doctoral Foundation of Ministry of Education of China (200803350118), and the Science and Technology Program of Ningbo (2009D10005) are gratefully acknowledged.
References
- Amer , WA , Wang , L , Amin , AM , Ma , L and Yu , H . 2010 . Recent progress in the synthesis and applications of some ferrocene derivatives and ferrocene-based polymers . Journal of Inorganic and Organometallic Polymers and Materials , 20 : 605 – 15 .
- Cazacu , M , Vlad , A , Marcu , M , Racles , C , Airinei , A and Munteanu , G . 2006 . New organometallic polymers by polycondensation of ferrocene and siloxane derivatives . Macromolecules , 39 : 3786 – 93 .
- Cyr , P , Tzolov , M , Manners , I and Sargent , E . 2003 . Photooxidation and photoconductivity of polyferrocenylsilane thin films . Macromolecular Chemistry and Physics , 204 : 915 – 21 .
- Hudson , RDA . 2001 . Ferrocene polymers: current architectures, syntheses and utility . Journal of Organometallic Chemistry , 637–639 : 47 – 69 .
- Wolf , MO . 2001 . Transition-metal-polythiophene hybrid materials . Advanced Materials , 13 : 545 – 53 .
- Zotti , G , Zecchin , S , Schiavon , G , Berlin , A , Pagani , G and Canavesi , A . 1995 . Conductivity in redox modified conducting polymers. 2. Enhanced redox conductivity in ferrocene-substituted polypyrroles and polythiophenes . Chemistry of Materials , 7 : 2309 – 15 .
- Zhu , SS and Swager , TM . 1996 . Design of conducting redox polymers: a polythiophene-Ru(bipy)3 n+ hybrid material . Advanced Materials , 8 : 497 – 500 .
- Zhu , Y and Wolf , MO . 1999 . Electropolymerization of oligothienylferrocene complexes: spectroscopic and electrochemical characterization . Chemistry of Materials , 11 : 2995 – 3001 .
- Zhang , D , Zhang , Q , Su , J and Tian , H . 2009 . A dual-ion-switched molecular brake based on ferrocene . Chemical Communications , : 1700 – 1702 .
- Wang , X-B , Dai , B , Woo , H-K and Wang , L-S . 2005 . Intramolecular rotation through proton transfer: [Fe(η5-C5H4CO2 −)2] versus [(η5-C5H4CO2 −)Fe(η5-C5H4CO2H)] . Angewandte Chemie International Edition , 44 : 6022 – 4 .
- Abd-El-Aziz , AS and Todd , EK . 2003 . Organoiron polymers . Coordination Chemistry Reviews , 246 : 3 – 52 .
- Laskoski , M , Steffen , W , Smith , MD and Bunz , UHF . 2001 . Is ferrocene more aromatic than benzene? . Chemical Communication , : 691 – 692 .
- Alonso , B , Cuadrado , I , Moran , M and Losada , J . 1994 . Organometallic silicon dendrimers . Journal of the Chemical Society, Chemical Communications , : 2575 – 2576 .
- Casado , CM , Cuadrado , I , Moran , M , Alonso , B , Garcia , B , Gonzalez , B and Losada , J . 1999 . Redox-active ferrocenyl dendrimers and polymers in solution and immobilised on electrode surfaces . Coordination Chemistry Reviews , 185–186 : 53 – 79 .
- Nguyen , P , Gómez-Elipe , P and Manners , I . 1999 . Organometallic polymers with transition metals in the main chain . Chemical Reviews , 99 : 1515 – 48 .
- Fery-Forgues , S and Delavaux-Nicot , B . 2000 . Ferrocene and ferrocenyl derivatives in luminescent systems . Journal of Photochemistry and Photobiology A , 132 : 137 – 59 .
- Kannan , P , Senthil , S , Vijayakumar , R and Marimuthu , R . 2002 . Synthesis and characterization of liquid crystalline polymers containing aromatic ester mesogen and a nonmesogenic ferrocene unit in the spacer . Journal of Applied Polymer Science , 86 : 3494 – 501 .
- Chen , M and Diao , G . 2009 . Electrochemical study of mono-6-thio-β-cyclodextrin/ferrocene capped on gold nanoparticles: characterization and application to the design of glucose amperometric biosensor . Talanta , 80 : 815 – 820 .
- Yang , W , Zhou , H and Sun , C . 2007 . Synthesis of ferrocene-branched chitosan derivatives: redox polysaccharides and their application to reagentless enzyme-based biosensors . Macromolecular Rapid Communications , 28 : 265 – 70 .
- Zhang , L , Sun , H , Li , D , Song , S , Fan , C and Wang , S . 2008 . A conjugated polymer-based electrochemical DNA sensor: design and application of a multi-functional and water-soluble conjugated polymer . Macromolecular Rapid Communications , 29 : 1489 – 94 .
- Wu , Y , Liu , S and He , L . 2009 . Electrochemical biosensing using amplification-by-polymerization . Analytical Chemistry , 81 : 7015 – 21 .
- Paul , S , Chavan , NN and Radhakrishnan , S . 2009 . Polypyrrole functionalized with ferrocenyl derivative as a rapid carbon monoxide sensor . Synthetic Metals , 159 : 415 – 18 .
- Matsui , J , Abe , K , Mitsuishi , M , Aoki , A and Miyashita , T . 2009 . Quasi-solid-state optical logic devices based on redox polymer nanosheet assembly . Langmuir , 25 : 11061 – 6 .
- Bunte , C and Rühe , J . 2009 . Photochemical generation of ferrocene-based redox-polymer networks . Macromolecular Rapid Communications , 30 : 1817 – 22 .
- Tamura , K , Akutagawa , N , Satoh , M , Wada , J and Masuda , T . 2008 . Charge/discharge properties of organometallic batteries fabricated with ferrocene-containing polymers . Macromolecular Rapid Communications , 29 : 1944 – 9 .
- Meredith , MT , Kao , D-Y , Hickey , D , Schmidtke , DW and Glatzhofer , DT . 2011 . High current density ferrocene-modified linear poly(ethylenimine) bioanodes and their use in biofuel cells . Journal of the Electrochemical Society , 158 : B166 – 74 .
- Yan , Q , Yuan , J , Zai , Z , Xin , Y , Kang , Y and Yin , Y . 2010 . Voltage-responsive vesicles based on orthogonal assembly of two homopolymers . Journal of the American Chemical Society , 132 : 9268 – 70 .
- Abd-Alla , MM , El-Zohry , MF , Aly , KI and Abd-El-Wahab , MMM . 1993 . Arylidene polymers. XVIII. Synthesis and thermal behavior of organometallic arylidene polyesters containing ferrocene derivatives in the main chain . Journal of Applied Polymer Science , 47 : 323 – 9 .
- Pittman , CU . 1968 . Ferrocene-containing polyesters and polyamides . Journal of Applied Polymer Science, Part A: Polymer Chemistry , 6 : 1687 – 95 .
- Carraher , CE , Jorgensen , S and Lessek , PJ . 1976 . Synthesis of oligomeric group IVA ferrocene polyesters . Journal of Applied Polymer Science , 20 : 2255 – 8 .
- Combs CS, Ashmore CI. Ferrocene polyesters. US Patents 3,898,254. 1975.
- Hehn R, Kanitz A, Roth W. Electrochromic formulations and uses therefor. US Patents 20100210807. 2010
- Knobloch , FW and Rauscher , WH . 1961 . Condensation polymers of ferrocene derivatives . Journal of Polymer Science , 54 : 651 – 56 .
- Yu , H , Wang , L , Huo , J , Li , C and Tan , Q . 2009 . Synthesis of glycidyl ether of poly(bisphenol-A 1,1’-ferrocene dicarboxylate) and its electrochemical behavior . Designed Monomers and Polymers , 12 : 305 – 13 .
- Furman , J and Makaruk , L . 1996 . Ferrocenic liquid crystal polymers . Polimery , 41 : 423 – 5 .
- Tan , Q , Wang , L , Ma , L , Yu , H , Liu , Q and Xiao , A . 2009 . Electrochemical behaviors and anion recognition of ferrocene modified hyperbranched polyether . Macromolecules , 42 : 4500 – 10 .
- Akhter , Z , Khan , M and Bashir , M . 2004 . Novel organometallic aromatic azo polyesters based on ferrocene . Journal of Inorganic and Organometallic Polymers , 14 : 253 – 67 .
- He , W , Deng , F , Jiang , YY , Wu , D , Fan , HL and Jian , XG . 2010 . Novel organometallic aromatic polyester based on ferrocene . Chinese Chemical Letters , 21 : 748 – 52 .
- Mehdipour-Ataei , S and Babanzadeh , S . 2007 . Synthesis, characterization and properties of novel polyamides containing ferrocene unit and flexible spacers . Applied Organometallic Chemistry , 21 : 360 – 7 .
- Nigar , A , Khan , MS , Akhter , Z and Butt , MS . 2007 . Some new organometallic polyamides based on ferrocene . Journal of Inorganic and Organometallic Polymers and Materials , 17 : 509 – 16 .
- Akhter , Z , Bashir , MA and Khan , MS . 2005 . Synthesis, characterization and thermal degradation kinetics of ferrocene-containing aramids . Applied Organometallic Chemistry , 19 : 848 – 53 .
- Iqbal , N , Khan , MS , Saeed , MA and Akhter , Z . 2007 . Synthesis and physicochemical studies of ferrocene-containing materials . Applied Organometallic Chemistry , 21 : 862 – 9 .
- Fengjuan , X , Changxiao , Y , Erxin , W and Yunjun , L . 2010 . Thermal behaviors of ferrocenyl-terminated hyperbranched polyester . Journal of Inorganic and Organometallic Polymers and Materials , 20 : 278 – 83 .
- Ma , L , Wang , L , Tan , Q , Yu , H , Huo , J , Ma , Z , Hu , H and Chen , Z . 2009 . Study on synthesis and electrochemical properties of novel ferrocene-based compounds and their applications in anion recognition . Electrochimica Acta , 54 : 5413 – 5420 .
- Li , C , Wang , L , Deng , L , Yu , H , Huo , J , Ma , L and Wang , J . 2009 . Electrochemical assessment of the interaction of dihydrogen phosphate with a novel ferrocenyl receptor . Journal of Physical Chemistry B , 113 : 15141 – 4 .
- Tan , Q , Wang , L , Ma , L , Yu , H , Ding , J , Liu , Q , Xiao , A and Ren , G . 2008 . Study on anion electrochemical recognition based on a novel ferrocenyl compound with multiple binding sites . Journal of Physical Chemistry B , 112 : 11171 – 11176 .
- Huo , J , Wang , L , Yu , H-J , Deng , L-B , Zhou , J-F and Yang , Q . 2007 . Synthesis and electrochemical behavior of linear oligo(ferrocenylsilane) and hyperbranched poly(ferrocenylsilane) . Journal of Polymer Science Part B: Polymer Physics , 45 : 2880 – 9 .
- Bard , A and Faulkner , L . 2001 . Electrochemical methods fundamentals and applications , New York , NY : Wiley .
- Chen , T , Wang , L , Jiang , G , Wang , J , Dong , X , Wang , X , Zhou , J , Wang , C and Wang , W . 2005 . Electrochemical behavior of poly(ferrocenyldimethylsilane-b-dimethylsiloxane) films . Journal of Physical Chemistry B , 109 : 4624 – 30 .
- Wang , X , Wang , L , Wang , J and Chen , T . 2004 . Study on the electrochemical behavior of poly(ferrocenylsilane) films . Journal of Physical Chemistry B , 108 : 5627 – 33 .
- Senthil , S and Kannan , P . 2002 . Thermotropic main chain liquid crystalline polyesters containing ferrocene and phosphate units: synthesis and characterization . Liquid Crystals , 29 : 1297 – 303 .
- Gao , Y and Shreeve , JnM . 2005 . Main chain 1,1’-ferrocene-containing polyelectrolytes exhibiting thermotropic liquid-crystalline and fluorescent properties . Journal of Polymer Science Part A: Polymer Chemistry , 43 : 974 – 83 .
- Cârlescu , I , Scutaru , AM , Apreutesei , D , Alupei , V and Scutaru , D . 2007 . The liquid crystalline behaviour of ferrocene derivatives containing azo and imine linking groups . Liquid Crystals , 34 : 775 – 85 .
- Cârlescu , I , Scutaru , AM , Apreutesei , D , Alupei , V and Scutaru , D . 2007 . The liquid crystalline properties of some ferrocene-containing Schiff bases . Applied Organometallic Chemistry , 21 : 661 – 9 .
- Zhang , H , Yu , Z , Wan , X , Zhou , QF and Woo , EM . 2002 . Effect of molecular weight on liquid-crystalline bahavior of a mesogen-jacketed liquid crystal polymer synthesized by atom transfer radical polymerization . Polymer , 43 : 2357 – 61 .
- Ye , C , Zhanng , H-L , Huang , Y , Chen , E-Q , Lu , Y , Shen , D , Wan , X-H , Shen , Z , Cheng , SZD and Zhou , Q-F . 2004 . Molecular weight dependence of phase structures and transitions of mesogen jacketed liquid crystalline polymers based on 2-vinylterephthalic acids . Macromolecules , 37 : 7188 – 96 .
- Singh , P , Rausch , MD and Lenz , RW . 1989 . Ferrocene containing liquid crystalline copolyesters . Polymer Bulletin , 22 : 247 – 52 .