Abstract
Novel Schiff base polymer of N-(3-aminopropyl) pyrrole-salicylaldehyde and its copolymers with pyrrole were synthesized by interfacial polymerization, ultrasonic method, and electrochemical polymerization with the aim of producing and characterizing a new class of polymeric materials which could be used as separator of heavy metals such as Cu2+ and Ni2+. For this purpose, Schiff base structure was synthesized by condensation reaction of N-(3-aminopropyl) pyrrole with salicylaldehyde and that N-(3-aminopropyl) pyrrole was prepared from reduced product of pyrrole with 3-chloropropionitrile by lithium aluminum hydride (LiAlH4). The copolymers were synthesized with various molar ratios of pyrrole to N-(3-aminopropyl) pyrrole-salicylaldehyde (30:70, 40:60, and 50:50), via interfacial polymerization and also molar ratio of pyrrole to N-(3-aminopropyl) pyrrole-salicylaldehyde 30:70, via ultrasonic technique. The chemical structures of synthesized polymers and precursors were investigated by FT-IR and 1H NMR. Thermal behavior of polymers was investigated by thermal gravimetric analysis. Morphology of synthesized polymers was studied by scanning electron microscopy (SEM), the conductivity of obtained polymers was investigated by four-probe conductivity measurement technique and the electroactivity behavior of synthesized polymers was investigated by cyclic voltammetry (CV) method. In proof, the ability of polymers in absorption of Ni(II) and Cu(II) was obtained from atomic absorption analysis. Secondly, the homopolymer of N-(3-aminopropyl) pyrrole and its copolymers with pyrrole were prepared by electrochemical polymerization CV in various molar ratios and the electroactivity behavior of synthesized polymers was investigated.
1. Introduction
In recent years, conducting polymers, such as polyaniline, polypyrrole, and polythiophene, have received a great deal of attention owing to their high π-conjugated length, unusual conducting mechanism Citation[1], reversible redox doping/de-doping process Citation[2], and application in diverse emerging technologies such as light emitting diodes Citation[3], photoconductors Citation[4], static dissipaters Citation[5], and so forth. Among these polymers, a great deal of work was devoted to polypyrrole, prepared by chemical and electrochemical polymerization Citation[6–10], due to its interesting properties: redox activity Citation[11], the ability to form super capacitors in combination with multi-walled carbon nanotubes Citation[12], ion-exchange, ion discrimination capacities Citation[13,14], strong absorption properties toward gasses Citation[15], proteins Citation[16], DNA Citation[17], catalytic activity Citation[18,19], corrosion inhibitors Citation[20,21], and so forth.
The characteristics of conducting polymers strongly depend on their polymerization condition such as the film growth rate, the nature of solvent, the kind, and the concentration of dopant anion Citation[22–24]. Another possible way to give variation to polymer properties is to modify the chemical structure of monomer Citation[25]. Poly-N-alkylpyrrole has attracted attention as a possible alternative to polypyrrole for technological applications in spite of its lower conductivity Citation[26]. Diaz et al. Citation[27] prepared the series of poly(n-alkyl pyrrole) and showed that the nature of substituent affects the various properties such as redox potential, degree of oxidation, density, and conductivity. The class of conjugated polymers, polyazomethines, or poly (Schiff base)s are of considerable interest due to noticeable properties such as thermal and mechanical resistance; environmental stability; semiconducting, magnetic, and luminescence properties; and dissipaters Citation[28,29]. The first poly (azomethines) have been synthesized by Adams and co-workers from terphethalaldehyde and benzidine and dianisidine in 1923 Citation[30]. These polymers are produced by the polycondensation of amines with various carbonyl compounds via chemical or electrochemical oxidation Citation[29]. Moreover, the coordination ability of the metal with Schiff base polymer permits these materials to act as sensors Citation[18] and building blocks for supramolecular structures Citation[19]. The objectives of this ongoing study are to develop novel N-substituted Schiff base polymers of pyrrole (Py). For this purpose, firstly we have synthesized a novel Schiff base Py, N-(3-aminopropyl) Py-salicylaldehyde. Then, we have synthesized homopolymer of (N-3-aminopropyl) Py-salicylaldehyde and its copolymers with Py in various molar ratios, via interfacial polymerization, ultrasonic technique, and electrochemical polymerization with the aim of producing and characterizing a new class of polymeric materials.
2 Experimental
2.1 Materials and instrumentations
Pyrrole (97%, Merck) was distilled under reduced pressure before using and stored in 0 °C. The 3-chloropropionitrile, sodium hydride, tetrabutylammonium bromide, lithium aluminum hydride (LiAlH4), ammonium persulfate, magnesium sulfate, nickel(II) acetate, cu(II) acetate and salicylaldehyde (Merck), FeCl3·6H2O (Aldrich), and LiClO4 (Fulika) were used as received.
The solvents were reagent grade and, when necessary, were dried by standard techniques before using. The FT-IR spectra of samples were taken in KBr pellet using Bruker Tensor 27 spectrophotometer instrument. The chemical composition of the synthesized precursors was determined by 1H NMR using Bruker spectroscopy 400 MHz in CDCl3. Thermo gravimetric analyses (TGA) were performed using a SDTA, 852. The conductivity of polymers was measured at room temperature via a standard four-probe apparatus (Azar Electric Co., Iran). Scanning electron microscopy (SEM) images were collected by a LEO440I SEM.
2.2 Synthesis of monomer
The 2-cyanoethylpyrrole was prepared from 3-chloropropionitrile and Py by following the literature procedures Citation[29]. Yield (79%), FT-IR (cm−1): 2900(C–H aliphatic), 2250 CN. The 2-cyanoethylpyrrole in the presence of LiAlH4 was reduced to obtain N-(3-aminopropyl) Py Citation[30]. Yield (80%), FT-IR (cm−1): 3400 (NH2). 1H NMR (CDCl3) σ (ppm): 1.44 (s, 2H, NH2), 1.96 (m, 2H, Py–CH2–CH2–CH2–NH2, 2.71 (t, 2H, Py–CH2–CH2–CH2–NH2), 4 (t, 2H, Py–CH2–CH2–CH2–NH2).
2.2.1 Synthesis of N-(3-aminopropyl) pyrrole-salicylaldehyde Schiff base (N3APS) and its complexes with Ni(II) and Cu(II)
2.2.1.1 Synthesis of Schiff base
Salicylaldehyde (8 × 10−3 mol, 0.976 g) and N-(3-aminopropyl) Py (8 × 10−3 mol, 1.03 g) solutions in anhydrous ethanol (50 ml) were heated for 2 h under reflux. After cooling, the mixture was poured in ice water to obtain oil viscous. The crude product was purified by chromatography (pet. Petroleum ether: acetone 2:1) on silica to give the product in 83% yield. FT-IR (cm−1): 1645 (C=N), 3400 (O–H).1H NMR (CDCl3) σ (ppm): 2.18 (m, 2H, Py–CH2–CH2–CH2–N=CHAr), 3.54 (t, 2H, Py–CH2–CH2–CH2–N=CHAr), 4.01 (t, 2H, CH2–CH2–CH2–N=CHAr), 6.61 (d, 2H, β Py), 6.66 (d, 2H, α Py), 6.94 (m, 1H, Ar), 7.21 (d, 1H, Ar), 7.35 (m, 2H, Ar), 8.31(s, 1H, N=CH), 13.33 (s, 1H, OH).
2.2.1.2 Synthesis of complexes of Schiff base with Ni(II) and Cu(II)
A solution of N3APS (1.823 g, 8 × 10−3mol) and Ni acetate hydrates (85 × 10−2 g, 4 × 10−3 mol) in ethanol (70 ml) was heated for 2 h under reflux. Then, the mixture was cooled, filtered, and washed with methanol to give the colored metal complexes, and then the dark green color precipitate was obtained after recrystallization from petroleum ether in 72% yield. Complex of Schiff base with Cu(II) was prepared according to the similar procedure as mentioned above for the synthesis of polySchiff base complex with Ni(II). The oil complex was recrystallized with carbon tetrachloride to obtain dark brown precipitate in 78% yield.
2.3 Synthesis of homopolymer and copolymer
2.3.1 Synthesis of poly N-(3-aminopropyl) pyrrole-salicylaldehyde (PN3APS) by interfacial polymerization (I)
The synthesized Schiff base (2 × 10−3 mol and 0.5 g) from method 2.2.1 was dissolved in chloroform (15 ml) as organic phase and ammonium persulfate (5 × 10−3 mol and 0.0114 g) was dissolved in H2SO4 solution (1.7 M, 15 ml) as aqueous phase. In the beaker, the aqueous phase was slowly poured to the organic phase and allowed to stand for 48 h. Prepared polymers were filtered and washed several times by methanol and then distilled water in order to eliminate the oligomers and unreacted materials. Resulted nanostructure polymers were immersed in NH3 solution (5%) for 3 h to be reduced chemically to the neutral state completely Citation[31]. For comparison, polypyrrole was synthesized similar to the mentioned method. The polySchiff base structure was characterized by FT-IR, SEM, TGA, and four-probe conductivity methods.
2.3.2 Synthesis of PN3APS by interfacial polymerization
Copolymers were synthesized according to the similar procedure as mentioned above for the synthesis of homopolymers. Copolymers were synthesized in various molar ratios of Py:3-aminopropylpyrrole-salicylaldehyde (30:70, 40:60, and 50:50). Mixture of monomers (0.01 mol) was dissolved in 25 ml chloroform and then ammonium persulfate (2.5 × 10−3 mol and 0.57 g) dissolved in an aqueous H2SO4 (1.7 M and 25 ml) was poured to the organic phase slowly. After 24 h, resulted copolymers were purified in a similar way that was mentioned previously for homopolymer (I). The structure of nano copolymer (30:70) was characterized by FT-IR, SEM, TGA, and four-probe conductivity methods.
2.3.3 Synthesis of PN3APS and its copolymer with Py in mole ratio of 70:30 by ultrasonic method
Amount of materials in ultrasonic method is similar to the interfacial polymerization. In order to the preparation of homo and copolymers, monomers were added to aqueous H2SO4 and fixed in ultrasonic system and then ammonium persulfate solution in aqueous H2SO4 was added dropwise to the monomers mixture. Temperature was maintained at 25 °C during the reaction. After 30 min, obtained polymers were filtered, washed with methanol, and reduced similar to interfacial method. The structure of nanopolymers was characterized by SEM, TGA, and four-probe conductivity methods.
2.4 Electrochemical polymerization of Schiff base and its copolymers with pyrrole
Electrochemical polymerization of the Schiff base was performed by cycling the potential between −0.4 and + 1 V in acetonitrile solution containing LiClO4 as the reagent electrolyte. Moreover, the electrochemical copolymerization of the mixture of Py and Schiff base was carried out in various molar ratios of the Py:Schiff base (10:90, 25:75, 50:50, and 75:25). For comparison, polypyrrole was synthesized similar to the mentioned method. All experiments were performed with a conventional three-electrode system having glassy carbon (GC) electrode as a working electrode, a platinum wire as an auxiliary electrode, and an Ag/Ag+ as a reference electrode. Electrodepositions were carried out in 0.1 M solution of monomers in electrolyte at a scan rate of 10 mV s−1 for 30 cycles.
3 Result and discussion
The synthesis route of N-(3-aminopropyl) Py has been shown in Scheme . In stage 1, Py was deprotonized in the presence of alkaline solution, then the N–Na ionic bond in the presence of tetrabutylammonium bromide was transferred into an organic phase. The choice of a suitable tetralkylammonium cation enables transfer of its ion pair with the Py anion from the aqueous phase into an organic one of low polarity, where it can react with the alkyl halide at the harder (N) center Citation[32]. In the stage, 2,2-cyanoethylpyrrole was reduced by LiAlH4 to give amino compound.
Schiff base was synthesized by coupling reaction of N-(3-aminopropyl) Py with salicylaldehyde according to Scheme .
Schiff base ligands can be used as synthetic separators for inorganic cations. In order to confirm the structure of Schiff base, we prepared Schiff base complexes with Cu(II) and Ni(II) according to Scheme .
In general chemical method, polymerization of Schiff base was not carried out in the presence of FeCl3 and at room temperature which may be concerned with the coordination of Fe3+ with Schiff base. We have synthesized homopolymer of Schiff base and its copolymer with Py in various mole ratios via ultrasonic, interfacial, and electrochemical polymerization and then, we investigated the ability of polymers in absorption of Ni(II) and Cu(II) with atomic absorption analysis.
3.1 FT-IR spectra
FT-IR spectra of precursors and polymers have been shown in . In Figure , the peak at 2250 cm−1 was attributed to the CN group of 2-Cyanoethylpyrrole and also in Figure , N-(3-aminopropyl) Py has presented characteristic peak at 3400 cm−1 due to NH2 group. In Figure , Schiff base showed characteristics peaks at 1645 and 3400 cm−1 due to imine group (C=N) and OH of aromatic ring, respectively. The FT-IR spectrum of Schiff base showed a strong peak at 1645 cm−1, which was assigned to the C=N stretching, and in the case of metal complexes, this peak was shifted to lower frequency at 1618 cm−1 (Figure ), due to metal ions coordination through imines nitrogen Citation[33]. A band at 1645 cm−1 is assigned to C=O stretching frequency in the spectrum of Schiff base which is also shifted to lower frequency at 1617 cm−1 in metal complexes. This shows the involvement of oxygen atom of hydroxyl group in bonding with metal ions Citation[33]. Additional peaks at 620–662 and 540–550 cm−1 were found in the spectra of metal complexes corresponding to metal–O and metal–N bonds, respectively Citation[34].
Figure 1 FT-IR spectra of (a) 1-cyanoethylpyrrole, (b) N-3-aminopropylpyrrole, (c) N3APS, (d) complex of N3APS with Ni(II), and (e) complex of N3APS with Cu(II).
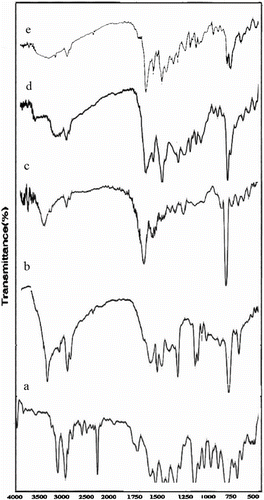
Figure 2 FT-IR spectra of (a) polypyrrole, (b) PN3APS, (c) copolymer N3APS/Py (70/30), (d) copolymer of N3APS/Py (60/40), and (e) copolymer of N3APS/Py (50/50).
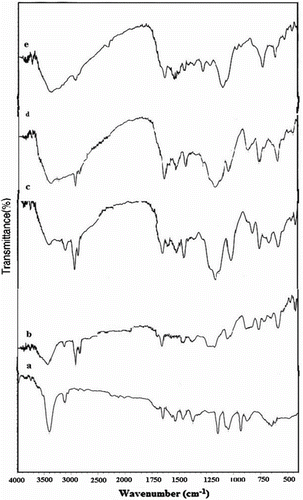
PolySchiff base showed characteristics peaks at 2900 and 3427 due to C–H aliphatic of polymer and OH group, respectively (Figure ). The FT-IR of copolymers with various molar ratios of Py has been shown in Figure . The signals at 3400, 1450–1500, 2900, and 1641–1647 cm−1 are attributed to N–H stretching vibration, N–H bending vibration, C–H aliphatic, and CH=N, respectively. Comparison of C–H aliphatic band shows that intensity of bands was decreased with increasing of mole ratio from 30 to 50% Py. The other peaks in 3100, 1450–1463 cm−1, and 1020–1066 cm−1 are, respectively, due to the C–H, C = C, and C–N bonds of Py in copolymer.
3.2 Investigation of 1H NMR spectra of N-(3-aminopropyl) pyrrole and N3APS
The 1H NMR spectra of N-(3-aminopropyl) Py and N3APS were obtained in CDCl3. The numbers of protons corresponding to each resonance based on integration of the resonances of the 1H NMR spectra are in good agreement with the proposed structure. Figure shows the 1H NMR spectrum of N-(3-aminopropyl) Py. The relatively wide peak at 1.44 ppm was attributed to the protons of NH2 group in N-(3-aminopropyl) Py. The signals at 1.96, 2.71, 4, 6.16, and 6.66 ppm in the spectrum of N-(3-aminopropyl) Py are attributed to the protons of Py ring, Ha, Hb, Hc, Hd, and He, respectively.
Figure shows the 1H NMR spectrum of N3APS. The signals at 2.8, 3.54, and 4.01 ppm are attributed to the aliphatic protons, Ha, Hb, and Hc, respectively. The peaks of Hd and He are appeared at 6.61 and 6.94–7.35 ppm, respectively. The signals of –OH (phenolic) and –CH=N (imine) groups of Schiff base are observed at 13.33 and 8.31 ppm, respectively, which prove the structure of the prepared Schiff base.
3.3 Study of ability of prepared homo poly Schiff base in absorption of metals
One of the main purposes of this research work is to produce a new class of polymeric materials which could be used as a separator of heavy metals such as Cu2+ and Ni2+ from their solutions. To investigate the ability of prepared homo poly Schiff base in the absorption of metals, a solution containing specified amount of Cu2+ and Ni2+ (50 ppm) was prepared in the aqueous phase, then a certain amount of poly Schiff base (0.01 g) was added to the above solution, which was stirred for 3 h. Concentration of metal ions was measured by atomic absorption spectrophotometry and the results are shown in Table . The experimental results show that concentration of ions has been decreased after interaction with polySchiff base. It is certified that prepared polymer could be used as Schiff base in the absorption of metals. Also, the ability of polySchiff base in absorption of Ni2+ is more than Cu2+.
Table 1. Metal absorption ability of PN3APS.
3.4 Study of thermal analysis of homo and co PN3APS with Py
The TGA thermograms of polySchiff base and its copolymer with Py prepared by interfacial method in ratio of 70:30 are presented in Figure , respectively. The first weight loss (about 10%) in all thermograms observed up to 300 °C can be due to loss of water and also removal of volatile compounds from the homo and copolymers of Schiff base structure. The 60% weight loss between 300 and 400 °C in Figure can be attributed to the N-substituents of Py. Figure shows TGA of polySchiff base and its copolymer with Py in ratio of 70:30. In this figure, weight loss of 20% in temperature range of 300–400 °C is assigned to the N-substituent of Py. As seen in the TGA curves in Figure , thermal stability in the case of copolymer increased compared to that of homo polymer of Schiff base. Thus, it may be concluded that the presence of Py monomer in the chain of copolymer improved the thermal stability of polyShiff base.
3.5 Investigation of morphology of PN3APS and its copolymer with molar ratio of Py to N3APS 30:70
The SEM images of PN3APS and its copolymers are shown in Figure . Figure illustrate scanning electron micrographs of homo and copolymers prepared by interfacial polymerization. It is clear from these images that the particles have well-defined spherical shape with approximate sizes of 300 nm. While comparing Figure , it was observed that the size of polymers (100 nm) prepared by ultrasonic method is smaller than that of polymers (300 nm) prepared by interfacial polymerization. In the sonicated method, the size of particles is smaller than other used methods. In this method, the aggregation of polymer particles occurs less than others. Furthermore, it is concluded that both the methods lead to prepare homo- and copolymers with nano sizes.
3.6 Investigation of electrical conductivity of PN3APS and its copolymer with different molar ratios of Py
Electrical conductivity of PN3APS and its copolymers with various molar ratios of Py:Schiff base have been summarized in Table . Electrical conductivity of homo- and copolymers was measured by standard four-probe method. The comparison of results reveals that the least and the most conductivity values related to homopolymer N3APS and PPy were prepared by interfacial polymerization, respectively. The electrical conductivity of copolymers increases with an increase in Py molar ratio. Also, the results show that electrical conductivity of polymers prepared by ultrasonic method was much better than those of polymers prepared by interfacial polymerization. It is attributed to the small particle size for polymers prepared by ultrasonic method. It is clear from Table that conductivity of N-(3-aminopropyl) Py-salicylaldehyde Schiff base N3APS is lower than that of PPy. It is due to a planar structure of PPy in which electrons can transform easily in intra chain of polymers.
Table 2. Electrical conductivity (S cm−1) of PN3APS and its copolymers with various molar ratios of Py.
3.7 Study of solubility of PN3APS and its copolymers with various molar ratios of Py:Schiff base
The solubility of PN3APS and its copolymers with various molar ratios of Py:Schiff base in organic solvents have been summarized in Table . In contrast to a complete insolubility of PPY, N3APS exhibits good solubility in some solvents. The increase of the solubility could be attributed to an incorporation of isopropyl substituent in the Py. The lower the Py content is, the better the solubility of the copolymer in these solvents is. Anyhow, it is concluded that the insolubility of PPy has been overcome greatly by a copolymerization of Py with N3APS. Preparation of conductive polymers with Schiff base structure soluble in organic solvents is one of the main purposes of this research work. The test solubility was done in 0.001 g polymer in 3 ml solvent.
Table 3. Solubility of PN3APS and its copolymers with various molar ratios of Py:Schiff base.
3.8 Investigation of electrochemical behavior of PN3APS and its copolymers with various molar ratios of Py
To investigate the electrochemical behavior, PN3AS and its copolymers with various molar ratios of Py:Schiff base (100:0, 10:90, 25:75, 50:50, 75:25, and 0:100) were prepared and then were casted on a GC electrode. The reference electrode was Ag/AgCl and a platinum wire was used as auxiliary electrode. The 0.1 M LiClO4 aqueous solution was used as the supporting electrolyte. Potential range for voltammograms was chosen between −400 and 900 mV at a scan rate of 10 mV s−1. Thirty cycles were used for electropolymerization. All experiments were carried out in room temperature (Figure ). Figure shows cyclic voltammogram of PPy. In the voltammograms of PPy, the oxidation and reduction peaks are appeared at + 0.23 and −0.2 V, respectively. However, in the cyclic voltammogram of PN3APS (Figure , no detectable redox peak was observed. It may mean that doping and undoping in polySchiff base and copolymer were not carried out easily due to slow transport of ion pair in and out of polymer chain because of twisted structure of polymer chains and the presence of N-substituent alkyls Citation[35]. Lallemand et al. Citation[36] have reported the electrochemical synthesis of N-substituted polypyrrole. They demonstrated that no detectable redox peak was observed in the cyclic voltammogram of N-Dodecylpyrrole, indicating that the prepared film on the electrode is not electroactive. They have found that it is difficult for N-substituted polypyrrole to be oxidized than Py. The anodic and cathodic peak currents of N-substituted polypyrrole are shorter than anodic and cathodic peak currents of Py. However, electrochemical behavior of copolymers increases with the increase of Py molar ratios in chain polymer.
Figure 7 Cyclic voltamograms of (a) polypyrrole, (b) copolymer of Py/N-3-aminopropylpyrrole-samlicylaldehyde (10/90), (c) copolymer of Py/N-3-aminopropylpyrrole-salicylaldehyde (25/75), (d) copolymer of Py/N-3-aminopropylpyrrole-salicylaldehyde (50/50), (e) copolymer of Py/N-3-aminopropylpyrrole-salicylaldehyde (75/25), and (f) Poly(N-3-aminopropyl Py-salicylaldehyde).
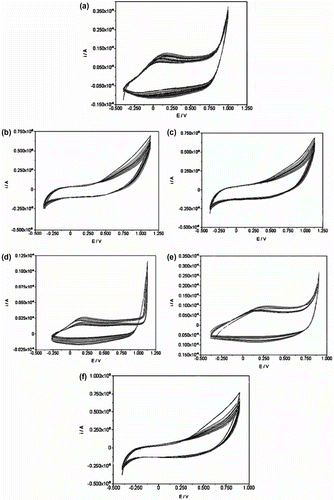
Conclusions
In this paper, N-(3-aminopropyl) pyrrole-salicylaldehyde Schiff base was prepared, with high yield and purity, from Py monomer. Then, polySchiff base N-(3-aminopropyl) Py-salicylaldehyde and its copolymers with Py were synthesized by interfacial, electrochemical polymerization, and ultrasonic method. Surface morphology, electrical conductivity, and solubility of prepared homopolymer and its copolymers were investigated. The SEM micrographs revealed that the resulting polymers have spherical morphology with approximate sizes of 200–500 nm. The results showed that the polySchiff base N-(3-aminopropyl) Py-salicylaldehyde and its copolymers containing 30 mol% of Py exhibited good solubility in some solvents such as toluene and THF. One of the most important uses of prepared copolymers was their ability in the absorption of metals and formation of complex with metal ions. Also, homopolymer of N-(3-aminopropyl) Py and its copolymers with Py were prepared by electrochemical polymerization CV in various molar ratios and their electroactivity behavior was confirmed by cyclic voltammograms. The results showed that the electroactivity behavior of homopolymer of N-(3-aminopropyl) Py and its copolymers increased with the increase of Py molar ratios in the chain of polymer. Based on analytical data, the prepared copolymers have Schiff base structure. They are electroactive, electrical conductive, and soluble in some solvents. The results showed that the ultrasonic method of polymerization was concluded to be better than other methods.
References
- Ansari , R . 2006 . Polypyrrole conducting electroactive polymers: synthesis and stability studies . E-Journal Chemistry , 3 : 186 – 201 .
- Mohsen-Nia , M and Seyed Mohammad Doulabi , F . 2012 . Preparation and characterization of CoFe2O4/poly vinyl acetate nanocomposite . Polymer-Plastics Technology and Engineering , 51 : 1122 – 1126 .
- Mohamad , SA , Javed , A , Lawrence , AD and Mohan , R . 2011 . Recent advances in conjugated polymers for light emitting devices . International Journal of Molecular Sciences , 12 : 2036 – 2054 .
- Kwang , SR , Kwang , MK , Young , SH , Yong , JP and Soon , HC . 2002 . The polyaniline electrode doped with li salt and protonic acid in lithium secondary battery . Bulletin of the Korean Chemical Society , 23 : 759 – 767 .
- Groenendaal , LB , Jonas , F , Freitag , D , Pielartzik , H and Reynolds , JR . 2000 . Poly (3,4-ethylenedioxyth-iophene) and its derivatives: past, present, and future . Advanced Materials , 12 : 481 – 494 .
- Henry , CM , Hsueh , CC , Timko , BP and Freund , MSJ . 2001 . Reaction of pyrrole and chlorauric acid a new route to composite colloids . Journal of the Electrochemical Society , 148 : D155 – D162 .
- Pringle , JM , Efthimiadis , J , Howlett , PC , Efthimiadis , J , Macfarlane , DR , Chaplin , AB , Hall , SB , Officer , DL , Wallace , GG and Forsyth , M . 2004 . Electrochemical synthesis of polypyrrole in ionic liquids . Polymer , 45 : 1447 – 1453 .
- Bazzaoui , M , Martins , JI , Reis , TC , Bazaoui , EA , Nunes , MC and Martin , LS . 2005 . Electrochemical synthesis of polypyrrole on ferrous and non-ferrous metals from sweet aqueous electrolytic medium . Thin Solids Films , 485 : 155 – 159 .
- Farid , A . 2006 . Electrochemical polymerization of pyrrole into nanostructured p-type porous silicon . Journal of the Electrochemical Society , 153 : C349 – C356 .
- Nilgün , K and Belkıs , U . 2005 . Chemical polymerization of pyrrole in the presence of ketone–formaldehyde resins . Journal of Applied Polymer Science , 96 : 618 – 624 .
- Heeger , AJ . 2001 . Semiconducting and metallic polymers: the fourth generation of polymeric materials . Reviews of Modern Physics , 73 : 681 – 700 .
- Khomenko , V , Frackowiak , E and Beguin , F . 2005 . Determination of the specific capacitance of conducting polymer/nanotubes composite electrodes using different cell configurations . Electrochimica Acta , 50 : 2499 – 2506 .
- Johanson , U , Marandi , M , Tamm , T and Tamm , J . 2005 . Comparative study of the behavior of anions in polypyrrole films . Electrochimica Acta , 50 : 1523 – 1528 .
- Weidlich , C , Mangold , KM and Jűttner , K . 2005 . EQCM study of the ion exchange behavior of polypyrrole with different counterions in different electrolytes . Electrochimica Acta , 50 : 1547 – 1552 .
- Chehimi , MM , Abel , ML , Perruchot , C , Delamar , M , Lascelles , SF and Armes , SP . 1999 . The determination of the surface energy of conducting polymers by inverse gas chromatography at infinite dilution . Synthetic Metals , 104 : 51 – 59 .
- Barisci , JN , Hodgson , AJ , Liu , L , Wallace , GG and Harper , G . 1999 . Electrochemical production of protein-containing polypyrrole colloids . Reactive and Functional Polymers , 39 : 269 – 275 .
- Nirmal , P , Kavita , A , Surinder , PS , Harpal , S and Bansi , DM . 2007 . DNA entrapped polypyrrole–polyvinyl sulfonate film for application to electrochemical biosensor . Analytical Biochemistry , 366 : 71 – 79 .
- Raoof , JB , Ojani , R and Rashid-Nadimi , S . 2004 . Preparation of polypyrrole/ferrocyanide films modified carbon paste electrode and its application on the electrocatalytic determination of ascorbic acid . Electrochimica Acta , 49 : 271 – 274 .
- Khomenko , VG , Barsukov , VZ and Katashinskii , AS . 2005 . The catalytic activity of conducting polymers toward oxygen reduction . Electrochimica Acta , 50 : 1675 – 1683 .
- Lehr , IL and Saidman , SB . 2007 . Corrosion protection of iron by polypyrrole coatings electro-synthesised from a surfactant solution . Corrosion Science , 49 : 2210 – 2225 .
- Denise , ML , Michel , D and Carlos , AF . 2003 . Application of polypyrrole/TiO2 composite films as corrosion protection of mild steel . Journal of Electroanalytical Chemistry , 540 : 35 – 44 .
- Gang , L , Vishal , S , Jinsong , H , Yan , Y , Tom , M , Keith , E and Yang , Y . 2005 . High-efficiency solution processable polymer photovoltaic cells by self-organization of polymer blends . Nature Materials , 4 : 864 – 868 .
- Varutt , K , Tetsuro , H , Hiroki , M , Hitoshi , K , Akihiko , F and Masanori , O . 2009 . Effect of solvent vapor treatment on photovoltaic properties of conducting polymer/C60 interpenetrating heterojunction structured organic solar cell . Thin Solid Films , 518 : 518 – 521 .
- Cortés , MT and Sierra , EV . 2005 . Effect of synthesis parameters in polyaniline: influence on yield and thermal behavior . Polymer Bulletin , 56 : 37 – 45 .
- Bedre , MD , Basavaraja , S , Deshpande , R , Balaji , DS and Venkataraman , A . 2010 . Preparation and characterization of polypyrrole silver nanocomposites via interfacial polymerization . International Journal of Polymeric Materials , 59 : 531 – 543 .
- Kim , JJ , Ameniya , TD , Tryk , A , Hashimoto , K and Fujishima , A . 1996 . Charge transport processes in electrochemically deposited poly(pyrrole) and poly(N-methylpyrrole) thin films . Journal of Electroanalytical Chemistry , 416 : 113 – 119 .
- Diaz , AF , Castillo , JI , Kanazawa , KK , Logan , JA , Salmon , M and Fajardo , O . 1982 . Conducting poly-N-alkylpyrrole polymer films . Journal of Electroanalytical Chemistry , 133 : 233 – 239 .
- Jun , Y , Weilin , S , Huajiang , J and Zhiquan , S . 2005 . Synthesis and properties of two novel poly(Schiff base)s and their rare-earth complexes . Polymer , 46 : 10478 – 10483 .
- Bingjing , H , Weilin , S , Miao , W and Zhiquan , S . 2004 . Synthesis and magnetic property of a novel SWNT-poly (Schiff base)-Nd3+ complex . Material Chemistry and Physics , 87 : 222 – 226 .
- Niu , HJ , Huanga , YD , Baib , XD and Lia , X . 2004 . Novel poly-Schiff bases containing 4,4′-diamino-triphenylamine as hole transport material for organic electronic device . Materials Letters , 58 : 2979 – 2983 .
- Tezuka , Y , Aoki , K and Ishii , T . 1999 . Alternation of conducting zone from propagation-control to diffusion-control at polythiophene films by solvent substitution . Electrochemi Acta , 44 : 1871 – 1877 .
- Makosza , M . 1975 . Two-phase reactions in the chemistry of carbanions and halocarbenes. An useful tool in organic synthesis . Pure and Applied Chemistry , 43 : 439 – 462 .
- Sece , JM , Quiros , M and Garmendia , MJG . 2000 . Synthesis, X-ray crystal structure and spectroscopic, magnetic and EPR studies of copper (II) dimers with methoxy-di-(2-pyridyl)methoxide as bridging ligand . Polyhedron , 19 : 1005 – 1013 .
- Mounika k, Anupama B, Pragathi J, Gyanakumari C. Synthesis¸ characterization and biological activity of a Schiff Base derived from 3-ethoxy salicylaldehyde and 2-amino benzoic acid and its transition metal complexes. Journal of Science and Research. 2010; 2:513–524.
- Jaymand , M . 2011 . Poly(4-chloromethyl styrene-g-4-vinylpyridine)/TiO2 thin films as templates for the synthesis of polypyrrole in the nanometer-sized domain . Designed Monomers and Polymers , 14 : 433 – 444 .
- Lallemand , F , Auguste , D , Amato , C , Hvesi , L , Delhalle , J and Mekhalif , Z . 2007 . Electrochemical synthesis and characterization of N-substituted polypyrrole derivatives on nickel . Electrochemica Acta , 52 : 4334 – 4341 .