Abstract
Three soluble conjugated polythiophenes, poly[3-(cyclohexylidenemethyl)-thiophene] (PTC), poly[3-(4-piperidinemethylene)-thiophene] (PTN), and poly[3-(4-tetrahydropyranmethylene)-thiophene] (PTO) have been synthesized successfully in chloroform using FeCl3 as an oxidizing agent. In PTC, PTN, and PTO, substituted side-chains are connected with the polythiophene backbone through carbon–carbon double bond. While in the three polythiophenes, the significant difference is the substituent linked to the methylene effectively. Structures of the monomers and the corresponding soluble polymers were elucidated by 1H NMR, 13C NMR, and IR. Average molecular weights have been determined by Gel permeation chromatography (GPC). Photoluminescence spectra and Ultraviolet–visible spectra of the three polythiophenes in both solution and solid state were also investigated, and all peaks were bathochromically shifted when they were measured in the solid state. In addition, the polymers were also characterized by cyclic voltammetry (CV) and scanning electron microscopy (SEM).
1. Introduction
Conjugated polymers (CPs) have attracted great attention since the discovery of conducting polyacetylene in 1977 Citation[1]. The CPs exhibit high conductivity, good optical properties, and environment stability, and there has been tremendous interest in CP technology for various applications, such as solar cells Citation[2,3], organic light-emitting diodes Citation[4,5], organic field-effect transistors Citation[6–8], and sensors Citation[9,10]. It is well known that the solubility and processability of CPs are vital for these applications. Most of unsubstituted CPs exhibit poor solubility, low mechanical flexibility, and poor processability, attributed to the rigid conjugated backbone and intermolecular aggregation Citation[11]. To overcome these problems and fulfill different application requirements, chemical modifications of CPs have been performed actively in recent years. One work focuses on attaching the side-chains with special functional groups. The side-chain functionalization improves not only the solubility and processability in organic solvents but also the electrical, electrochemical, and optical properties of the CPs Citation[12–15]. For instance, unsubstituted polythiophenes have poor solubility, resulting in the limitations of promising applications Citation[16]. Poly(3-hexylthiophene) is soluble in common organic solvents and it has become one of the most utilized active layer materials both in high-performance organic field-effect transistors and organic photovoltaic devices Citation[17–20].
In addition, the properties of CPs are closely related to the conformations of polymer chain Citation[21–23]. The substituents on the polymer backbone play a crucial role in polymer conformation. For instance, the maximum absorption in Ultraviolet–visible (UV–vis) spectrum of poly(3-alkoxythiophene)s is red-shifted about 120 nm compared to that of poly(3-alkylthiophene)s in solid state Citation[24,25]. Therefore, design and synthesis of new CPs with special functional substituents for promising applications in many fields are highly desirable.
Herein, we first report the synthesis and characterization of a series of polythiophene derivatives, and the molecular structures of the three novel side-chain substituted conjugated polythiophenes are shown in Scheme .
With the aim of examing the influence of substituents on the polymerization and properties of the polymers, we report, herein, properties of three polymers. It was shown that the three substituents influence the electronic properties of the backbone (band gap, electrochemical behavior). Furthermore, the appearance of the absorption band and emission band influenced by the side-chain demonstrates that the three moieties effectively contribute electronically to material.
In this paper, the resulting polythiophenes were prepared by stoichiometric oxidative polymerizations of corresponding monomers with FeCl3, which generally give regiorandom polythiophenes. This is a very simple polymerization method but the drawback with the method is that the polymer must be carefully purified after polymerization to remove the residual iron.
2 Experimental
2.1 Materials
All chemical reagents were common commercial level and used as received without further purification. An Ag/AgCl (3 M NaCl) electrode served as reference electrode, and it was bought from Gaoss Union. 3-(bromomethyl)thiophene (1) and (thiophene-3-ylmethyl)phosphonic acid diethyl ester (2) were prepared as reported previously Citation[26].
2.2 Characterization
The UV–vis spectra were taken with a Perkin-Elmer Lamda 900 UV–vis-near-infrared spectrophotometer. Infrared spectra were recorded using Bruker Vertex 70 FT-IR spectrometer with KBr pellets. 1H NMR and 13C NMR spectra of the polymers were recorded on a Bruker AV 400 NMR spectrometer and CDCl3 was used as solvent. Gel permeation chromatography (GPC) measurements were performed with a Waters 2410 refractive-index detector at 35 °C, and THF was used as eluent at a flow rate of 1.0 mL/min. The GPC data were calibrated with polystyrene standards. The thermogravimetric analysis (TGA) was performed with a thermal analyzer of Diamond TG/DTA. The fluorescence properties were determined with Hitachi F-4500 fluorescence spectrophotometer. Scanning electron microscopy (SEM) measurements were carried out made with a cold field emission scanning electron microscope PEG 250. The electrochemical cyclic voltammetry (CV) was conducted on a Zahner IM6e Electrochemical Workstation.
2.3 Synthesis of monomers and polymers
2.3.1 Synthesis of [3-(N-Boc-4-piperidinemethylene)-thiophene] (3)
A mixture of N-BOC-4-Piperidone (1.99 g, 0.01 mol), 60% NaH (0.74 g, 0.03 mol), and THF (20 ml) was stirred for 30 min under an ice-water bath, and the flow of dry N2 gas allowed for an inert atmosphere. Then, compound 2 (2.34 g, 0.01 mol) was slowly dropped at 0 °C. After completion, the reaction mixture was warmed to room temperature and stirred further for 12 h. Then, the mixture was washed with water and extracted with ether. The organic layer was dried over MgSO4, filtered, and concentrated. The crude product was purified by chromatography on silica gel with hexane/ethyl acetate = 6:1 as an eluent; this afforded the pure substance as a colorless oil (1.97 g, yield: 80%). Ms: m/z = 279[M+]. 1H NMR (CDCl3, ppm, δ): 7.25 (d, J = 6.8 Hz, 1H, Th–C5–H), 7.10 (s, J = 6.0 Hz, 1H, Th–C2–H), 7.04 (d, J = 6.0 Hz, 1H, Th–C4–H), 6.29 (s, 1H, CH=C), 3.51 (t, J = 10.4 Hz, 4H, 2 × CH2), 2.53 (t, J = 10.4 Hz, 4H, 2 × CH2), and 1.49 (s, J = 4.4 Hz, 9H, 3 × CH3).
2.3.2 Synthesis of [3-(4-piperidinemethylene)-thiophene] (4)
A mixture of compound 3 (3.23 g, 0.013 mol) and anhydrous formic acid (25 ml) was stirred overnight at room temperature. After completion, the mixture was concentrated under reduced pressure. A saturated NaHCO3 solution was added to the mixture until the pH of the solution was over 10–11. The solution was extracted with chloroform and dried over anhydrous MgSO4. The solvent was removed and the crude compound was further purified by column chromatography [silica gel; eluent: ethyl acetate/methanol/triethylamine = 4/1/1] and isolated as viscous colorless oil (0.8 g, yield: 83%). Ms: m/z = 179[M+]. 1H NMR (CDCl3, ppm, δ): 7.24 (d, J = 6.0 Hz, 1H, Th–C5–H), 7.18 (s, J = 6.4 Hz, 1H, Th–C2–H), 7.07 (d, J = 6.4 Hz, 1H, Th–C4–H), 6.20 (s, 1H), 2.73 (t, J = 8.8 Hz, 4H, 2 × CH2), 2.67 (t, J = 8.8 Hz, 4H, 2 × CH2), and 2.56 (s, 1H, secondary amine–H). 13C NMR (CDCl3, ppm, δ): 153.4, 131.3, 125.6, 124.9, 122.3, 120.4, 50.3, 50.3, 43.7, and 35.8.
2.3.3 Synthesis of [3-(cyclohexylidenemethyl)-thiophene] (5)
The preparation process was the same as that for compound 3, except that N-BOC-4-Piperidone was used instead of cyclohexanone (1.18 g, 12 mmol), the crude compound 5 was further purified by column chromatography [silica gel; hexane] and isolated as viscous colorless oil (0.94 g, yield 53%). Ms: m/z = 178[M+]. 1H NMR (CDCl3, ppm, δ): 7.16 (d, J = 6.0 Hz, 1H, Th–C5–H), 7.01 (s, J = 4.8 Hz, 1H, Th–C2–H), 6.98 (d, J = 5.6 Hz, 1H, Th–C4–H), 6.10 (s, 1H), 2.38 (t, J = 5.12 Hz, 2H, CH2), 2.26 (t, J = 8.8 Hz, 2H, CH2), and 1.52–1.61 (m, 6H, 3 × CH2). 13C NMR (CDCl3, ppm, δ): 159.6, 131.2, 125.6, 124.7, 120.8, 120.4, 40.2, 27.6, 27.6, and 26.5.
2.3.4 Synthesis of [3-(4-tetrahydropyranmethylene)-thiophene] (6)
The same procedure for the synthesis of compound 6 was applied, except that tetrohydro-4H-pyran-4-one was used instead of cyclohexanone. The crude product was purified by column chromatography (silica gel, hexane/ethyl acetate = 6/1) to afford 6 as a colorless oil (0.20 g, yield 11%). Ms: m/z = 180[M+]. 1H NMR (CDCl3, ppm, δ): 7.15 (d, J = 3.2 Hz, 1H, Th–C5–H), 7.01 (s, J = 5.2 Hz, 1H, Th–C2–H), 6.99 (d, J = 5.2 Hz, 1H, Th–C4–H), 6.20 (s, 1H), 3.72 (t, J = 12.4 Hz, 2H, CH2), 3.68 (t, J = 12.4 Hz, 2H, CH2), 2.52 (t, J = 8.4 Hz, 2H, CH2), and 2.40 (t, J = 8.4 Hz, 2H, CH2). 13C NMR (CDCl3, ppm, δ): 152.8, 129.2, 125.5, 124.8, 121.1, 118.4, 70.8, 70.8, 26.1, and 19.8.
2.3.5 Synthesis of poly[3-(4-piperidinemethylene)-thiophene]
Under nitrogen atmosphere, 1.63 g (10.0 mmol) of anhydrous ferric trichloride and 20 ml of dry chloroform was quickly added to a reaction mixture and the mixture was cooled to 0–5 °C. The monomer 4 (0.42 g, 2.3 mmol) in 15 ml of chloroform was added to the reaction mixture dropwise, for 30 min. The mixture was stirred at room temperature for a period of two days. Treatment of the reaction mixture with methanol resulted in the precipitation of the polymer which was carefully dedoped by repeatedly treating a chloroform solution of the polymer with concentrated ammonia. Then, evaporated to dryness, use a Soxhlet apparatus to remove low molecular weight and irregular materials from polymer by extraction with chloroform. The pure poly[3-(4-piperidinemethylene)-thiophene] (PTN) was obtained as a red solid (0.28 g, yield 67%). Mn = 8310, Mw /Mn = 1.73. 1H NMR (CDCl3, ppm, δ): 7.12–6.91 (s, 2H), 6.21 (s, 1H), 3.12–2.81 (m, 4H), 2.80–2.60 (m, 4H), and 2.49–2.52 (s, 4H). FT-IR (KBr pellet, cm−1): 3301, 2938, 1613, 1414, 1269, 994, and 766.
2.3.6 Synthesis of poly[3-(cyclohexylidenemethyl)-thiophene]
Poly[3-(cyclohexylidenemethyl)-thiophene] (PTC) was prepared by the same procedure for the preparation of PTN using monomer 5 (0.50 g, 2.8 mmol) in 47% yield as a dark yellow-brown solid. Mn = 6791, Mw /Mn = 1.25. 1H NMR (CDCl3, ppm, δ): 6.99–6.91 (s, 1H), 6.29 (s, 1H), 2.41–2.62 (t, 4H), 1.67–1.75 (m, 4H), and 1.62 (s, 2H). FT-IR (KBr pellet, cm−1): 2926, 2854, 1636, 1448, 945, and753.
2.3.7 Synthesis of poly[3-(4-tetrahydropyranmethylene)-thiophene]
Poly[3-(4-tetrahydropyranmethylene)-thiophene] (PTO) was prepared by the same procedure for the preparation of PTN using monomer 6 (0.50 g, 2.8 mmol) in 69% yield as a dark red–violet solid. Mn = 11,788, Mw /Mn = 1.80. 1H NMR (CDCl3, ppm, δ): 7.01 (s, 1H), 6.29 (s, 1H), 3.29–3.98 (m, 4H), and 2.21–2.69 (m, 4H). FT-IR (KBr pellet, cm−1): 3067, 2922, 2877, 1741, 1424, 1097, 919, and 772.
3 Results and discussion
3.1 Synthesis and characterization of the monomers and polymers
Scheme displays the synthetic routes of PTN, PTC, and PTO. Monomer 4, 5, and 6 were polymerized using FeCl3 in chloroform to afford PTN, PTC, and PTO, respectively. The resulting three polymers are readily soluble in common organic solvents such as chloroform and THF.
Figure shows the 1H NMR spectra of monomer 4, 5, and monomer 6 in CDCl3. The d proton of the three monomers is at the same position about 6.2 ppm, which is the proton of carbon–carbon double bond. And, the other peaks of the protons are all correct, all above, confirm that the monomer 4, 5, and 6 had formed.
Figure shows the 1H NMR spectra of polymer PTC, PTN, and PTO in CDCl3. The 1H NMR spectra of the three polymers all revealed a singlet peak in the aromatic region at δ 7.0, which is attributable to the thiophene ring proton. And, the spectra also revealed a singlet peak at about δ 6.2, attributed to the proton of CH=C in the side-chains. Moreover, the other peaks of the protons are all correct, all above, confirm that the three polymers had formed successfully.
The FT-IR spectra of monomers and the resulting polymers are shown in Figure , which indicates the C–H and C=C stretching vibrations on the thiophene ring of the polythiophenes can be found at 2928 and 1452 cm−1, respectively. The C=C stretching vibrations in the side-chains can be found at 1639 cm−1 in Figure . The sharp and strong absorption band at about 849 cm−1 (C–H out-of-plane vibration), characteristics of 2,3,5-trisubstituted thiophene rings, is in agreement with α–α coupled monomers and it confirms the polymerization Citation[27]. The FT-IR spectra of monomer and polymer PTN show characteristic bands at 3301 cm−1, attributed to the N–H stretching, and at characteristic bands at 1097 cm−1 due to C–O–C in polymer PTO or monomer TO. The molar mass of the three polymers were determined by GPC in THF toward polystyrene standards. The PTC has a molar mass Mn = 6791 and a polydispersity D = Mw /Mn = 1.25, PTN also has a molar mass Mn = 8310 and polydispersity D = Mw /Mn = 1.73, and PTO has a molar mass Mn = 11,788 and polydispersity D = Mw /Mn = 1.80.
3.2 Thermal properties
Thermal properties of the three polymers were investigated by TGA. Decomposition temperature (T d) defined as the temperature at which 5% weight lose was detected by TGA. As shown in Figure , the T d of PTC, PTN, and PTO are around 254, 155, and 330 °C in nitrogen, respectively. The results indicate that the thermal stability of PTN is lower than that of PTN and PTO, which is mainly because of the easily oxidized secondary amine in the side-chain. Obviously, the thermal properties of the polymers are still adequate for further application in photovoltaic solar cells and other optoelectronic devices.
3.3 Optical properties
Figure displays UV–vis spectra of the polymers in different solutions and in the solid state. For PTC, the absorption maximum had red-shifted from 398 nm in chloroform to 413 nm in the solid state; for PTN, the red-shift was from 442 to 451 nm; and for PTO, the red-shift was from 475 to 507 nm. The absorption in the visible region should be attributed to π–π ∗ transition of the CP main chains. These data indicate the efficient π stacking and intermolecular interactions occurred in the film. In comparison with the absorption spectra of the polymer solutions in chloroform, the visible absorptions become red-shifted, similar to the case of poly(3-hexylthiophene) where a π-stacked structure was formed in the solid stateCitation[28]. For these polymers containing big side-chains, probably, the steric hindrance of side-chains make the polymer main chains distorted in solution, so that the main chain absorptions are weak and appear at short wavelength. In the solid film, the interchain interactions make the main chain extended and the conjugation degree increased which enhance the visible absorption and make the absorption red-shifted Citation[29].
Fluorescence spectra of the polymers were measured in solution and film state (Figure ). The PTC, PTN, and PTO in chloroform exhibited emission maximum wavelengths λ max s at 553, 565, and 589 nm, respectively. The PTC, PTN, and PTO films prepared from chloroform solution showed the maximum emission peak at 585, 603, and 660 nm, respectively, which are red-shifted compared with those in solution. These dates indicate that efficient π stacking and intermolecular interactions occurred in the films. The red shifts of the maximum emission peaks may also be attributed to the increase of effective conjugated length.
3.4. Electrochemical properties
The electrochemical property is one of the most important properties of the CPs, and many applications of the CPs depend on the electrochemical properties. We studied the electrochemical behavior of the PTC, PTN, and PTO by CV. Figure shows the cyclic voltammogram of PTC, PTN, and PTO films on Pt electrode. The highest occupied molecular orbital (HOMO) and lowest unoccupied molecular orbital (LUMO) energy levels of the polymer were calculated from the onset oxidation potentials (ϕ
ox), the onset reduction potentials (ϕ
red), and by assuming the energy level of ferrocene/ferrocenium (Fc/Fc+) to be −4.8 eV below the vacuum level Citation[30]. The energy level of Fc/Fc+ was measured as 0.09 eV against Ag/Ag+. The energy gap of the polymer was calculated from the HOMO and LUMO energy levels. The calculating equations are as follows Citation[31]:
Figure 7 Cyclic voltammograms of PTC (a), PTN (b), and PTO (c) films on platinum electrode in boron trifluoride ether solution with a scan rate of 0.1 V/s.
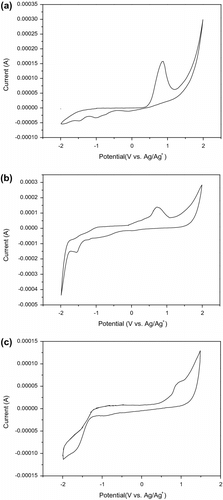
Where, the units of ϕ ox and ϕ red are V vs. Ag/Ag+. For comparing expediently, the electrochemical potentials and energy levels of PTC, PTN, and PTO are shown in Table . From Table , we can see that the HOMO energy level is decreased from PTC to PTO. The decease of the HOMO energy level could be attributed to the electronegativity in the side-chains. According to the early reported literature that the HOMO energy of P3HT is −4.79 eV Citation[32], the E HOMO are −5.20, 5.23 and −5.42 eV for PTC, PTN, and PTO. The lower HOMO energy levels of PTC, PTN, and PTO are desirable for a higher open circuit voltage of the polymer solar cells based on the polymers as donor materials. The onset oxidation potential (ϕ ox) of the three polymers are observed in 0.49, 0.52, and 0.71 V compared to the ϕ ox of P3HT (0.08 V) Citation[32] indicating that they have relatively higher air-stability than P3HT.
Table 1. Comparison of the optical and electrochemical properties of PTC, PTN, and PTO.
3.5. Scanning electron microscopy
Figure shows a SEM of thin film of (a) PTC, (b) PTN, and (c) PTO deposited onto ITO surface by spray coating. Surface morphologies of polymers were investigated by SEM. The SEM micrographs of the three polymers imply that they are good in film forming. Globule-like droplets were observed on surface of the polymer films, and the diameter of the globules is ranging from ca. 1 to 5 μm. The microspheres are of regular spherical shape.
4 Conclusions
Three soluble and highly conjugated polythiophene derivatives, PTC, PTN, and PTO, were synthesized by FeCl3 method. The monomers of the three polymers with side-chain containing CH=CH double bond were synthesized through Wittig–Horner reaction. In solid state, the emission maxima of PTC, PTN, and PTO are 585, 603, and 660 nm, respectively; it may be used in orange-red-light-emitting diodes. Electrochemical characterizations showed that the three polythiophenes possessed relatively higher oxidation potential and lower HOMO level than some other polythiophene derivatives, which is benefit for the air-stability. The TGA indicated good thermal stability. The three polythiophene derivatives possess good solution processibility and electrochemical properties, which may potentially lead to fabrication of high-performance organic electronic devices.
References
- Shirakawa , BH , Louis , EJ , MacDiarmid , AG , Chiang , C and Heeger , AJ . 1977 . Synthesis of electrically conducting organic polymers: halogen derivatives of polyacetylene, (CH)X . J. Chem. Soc. Chem. Commun. , 16 : 578 – 580 .
- Kim , Y , Cook , S , Choulis , SA , Nelson , J , Durrant , JR and Bradley , DC . 2004 . Organic photovoltaic devices based on blends of regioregular poly(3-hexylthiophene) and poly(9,9-dioctylfluorene-co-benzothiadiazole) . Chem. Mater. , 16 : 4812 – 4818 .
- Shi , CJ , Yao , Y , Yang , Y and Pei , QB . 2006 . Regioregular copolymers of 3-alkoxythiophene and their photovoltaic application . J. Am. Chem. Soc. , 128 : 8980 – 8986 .
- Akcelrud , L . 2003 . Electroluminescent polymers . Prog. Polym. Sci. , 28 : 875 – 962 .
- Perpichka , IF , Perpichka , DF , Meng , H and Wul , F . 2005 . Light-emitting polythiophenes . Adv. Mater. , 17 : 2281 – 2305 .
- Ong , BS , Wu , YL , Liu , P and Gardner , S . 2004 . High-performance semiconducting polythiophenes for organic thin-film transistors . J. Am. Chem. Soc. , 126 : 3378 – 3379 .
- Zou , YP , Wu , WP , Sang , GY , Yang , Y , Liu , YQ and Li , YF . 2007 . Polythiophene derivative with phenothiazine-vinylene conjugated side chain: synthesis and its application in field-effect transistors . Macromolecules. , 40 : 7231 – 7237 .
- Lu , K , Guo , YL , Liu , YQ , Di , C , Li , T , Wei , ZM , Yu , G , Du , CY and Ye , SH . 2009 . Novel functionalized conjugated polythiophene with oxetane substituents: synthesis, optical, electrochemical, and field-effect properties . Macromolecules. , 42 : 3222 – 3226 .
- Thomas , SW , Joly , GD and Swager , TM . 2007 . Chemical sensors based on amplifying fluorescent conjugated polymers . Chem. Rev. , 107 : 1339 – 1386 .
- Bao , Z . 2000 . Materials and fabrication needs for low-cost organic transistor circuits . Adv. Mater. , 12 : 227 – 230 .
- Wang , MF , Zou , S , Guerin , G , Shen , L , Deng , KQ , Jones , M , Walker , GC , Scholes , GD and Winnik , MA . 2008 . A water-soluble pH-responsive molecular brush of poly(N,N-dimethylaminoethyl methacrylate) grafted polythiophene . Macromolecules. , 41 : 6993 – 7002 .
- Wang , F and Lai , YH . 2003 . Conducting azulene-thiophene copolymers with intact azulene units in the polymer backbone . Macromolecules. , 36 : 536 – 538 .
- Tonzola , CJ , Alam , M and Jenekhe , SA . 2002 . New soluble n-type conjugated copolymer for light-emitting diodes . Adv. Mater. , 14 : 1086 – 1090 .
- Ashraf , RS and Kelmm , E . 2005 . Synthesis and properties of poly(heteroaryleneethynylene)s consisting of electron-accepting benzothiadiazole/quinoxaline units and electron-donating alkyl thiophene units . J. Polym. Sci., Part A: Polym. Chem. , 43 : 6445 – 6454 .
- Wong , MS , Li , ZH , Tao , Y and D’Iorio , M . 2003 . Synthesis and functional properties of donor−acceptor π-conjugated oligomers . Chem. Mater. , 15 : 1198 – 1203 .
- Yamoto , T , Sanechika , K and Yamomoto , A . 1980 . Preparation of thermostable and electric-conducting poly(2,5-thienylene) . J. Polym. Sci. Polym. Lett. Ed. , 18 : 9 – 12 .
- Huang , JS , Li , G and Yang , Y . 2005 . Influence of composition and heat-treatment on the charge transport properties of poly(3-hexylthiophene) and [6,6]-phenyl C61-butyric acid methyl ester blends . Appl. Phys. Lett. , 87 : 112105
- Wang , EG , Wang , L , Lan , LF , Luo , C , Zhuang , WL , Peng , GB and Cao , Y . 2008 . High-performance polymer heterojunction solar cells of a polysilafluorene derivative . Appl. Phys. Lett. , 92 : 033307
- Li , G , Shrotriya , V , Huang , JS , Yao , Y , Moriarty , T , Emery , K and Yang , Y . 2005 . High-efficiency solution processable polymer photovoltaic cells by self-organization of polymer blends . Nat. Mater. , 4 : 864 – 868 .
- Kim , JY , Lee , K , Coates , NE , Moses , D , Ngnyen , TO , Dante , M and Heeger , AJ . 2007 . Efficient tandem polymer solar cells fabricated by all-solution processing . Science. , 317 : 222 – 225 .
- Hidayat , R , Fujii , A , Ozaki , M and Yoshino , K . 2001 . Influences of interchain interaction on exciton dynamics in poly(3-alkylthiophene) . Jpn J. Appl. Phys., Part 1. , 40 : 7103 – 7109 .
- Lee , SJ , Lee , JM , Cho , H , Koh , WG , Cheong , IW and Kim , JH . 2010 . Jacketed polymers with dendritic carbazole side groups and their applications in blue light-emitting diodes . Macromolecules. , 43 : 2484 – 2486 .
- Choi , J , Ruiz , CR and Nesterov , EE . 2010 . Temperature-induced control of conformation and conjugation length in water-soluble fluorescent polythiophenes . Macromolecules. , 43 : 1964 – 1974 .
- Guillerez , S and Bidan , G . 1998 . New convenient synthesis of highly regioregular poly(3-octylthiophene) based on the Suzuki coupling reaction . Synth. Met. , 93 : 123 – 126 .
- Koeckelberghs , G , Vangheluwe , M , Samyn , C , Persoons , A and Verbiest , T . 2005 . Regioregular poly(3-alkoxythiophene)s: toward soluble, chiral conjugated polymers with a stable oxidized state . Macromolecules. , 38 : 5554 – 5559 .
- Greenwald , Y , Cohen , G , Poplawski , J , Ehrenfreund , E , Speiser , S and Davidov , D . 1996 . Transient photoconductivity of acceptor-substituted poly(3-butylthiophene) . J. Am. Chem. Soc. , 118 : 2980 – 2984 .
- Andreani , F , Bizzari , PC , Casa , CD and Salatelli , E . 1991 . First approach to ester-functionalized poly(3-alkylthienylene)s . Polym. Bull. , 27 : 117 – 121 .
- Yamamoto , T , Komarudin , D , Arai , M , Lee , BL , Suganuma , H , Asakawa , N , Inoue , Y , Kubota , K , Sasaki , S , Fukuda , T and Matsuda , H . 1998 . Extensive studies on π-stacking of poly(3-alkylthiophene-2,5-diyl)s and poly(4-alkylthiazole-2,5-diyl)s by optical spectroscopy, NMR analysis, light scattering analysis, and X-ray crystallography . J. Am. Chem. Soc. , 120 : 2047 – 2058 .
- Zhu , ZG and Swager , TM . 2001 . Conjugated polymers containing 2,3-dialkoxybenzene and iptycene building blocks . Org. Lett. , 3 : 3471 – 3474 .
- Sun , Q , Wang , H , Yang , C and Li , Y . 2003 . Efficient two layer leds on a polymer blend basis . J. Mater. Chem. , 13 : 800 – 806 .
- Huo , L , Zhou , Y and Li , Y . 2009 . Synthesis and electroluminescence of novel copolymers containing crown ether spacers . Macromol. Rapid Commun. , 30 : 925 – 931 .
- Prommerehne , J , Vestweber , H , Guss , W , Mahrt , RF , Bassler , H and Porsch , M . 1995 . Alkylthio-substituted polythiophene: absorption and photovoltaic properties . Adv. Mater. , 7 : 551 – 554 .