Abstract
This study is concerned to the immobilization of silver-loaded titanium dioxide (AT) nanoparticles (NPs) into or onto polyvinyl alcohol (PVA) as matrix. The nanocomposites films were fabricated via solution casting method. The optical properties and morphology of the NPs and nanocomposites were characterized by dynamic light scattering, transmission electron microscopy, fourier transform infrared spectroscopy, ultraviolet–visible spectroscopy, contact angle measurements, field emission scanning electron microscopy (FE-SEM), and atomic force microscopy (AFM). The obtained results of FE-SEM analysis for nanocomposites indicate that the 40–80 nm AT NPs are homogenously distributed in PVA matrix. The unsymmetrical parts of peaks and valleys for nanocomposites in three-dimensional AFM images were related to the residual of AT NPs in outsides, although in the majority of locations it showed similar patterns of peaks and valleys. The studies of nanocomposites by using UV revealed that existence of the AT NPs were increased the shield properties against UV light. Reinforcement of PVA with AT nanomaterials enhances the properties of the prepared films and develops their applications in different industrial fields such as packaging.
1. Introduction
The latest progresses in nanotechnology offer an exclusive chance to create innovatory materials with considerable properties Citation[1]. Nowadays, the studies on organic–inorganic hybrid materials have attracted much attention in view of their potential to coalesce the features of organic materials with those of inorganic materials, finally with synergic effects Citation[2]. Nanocomposites are a class of composites with at least one phase having a dimension in the neighborhood of 1–100 nm Citation[3]. In recent years, the synthesis and structural characterizations of polymer nanocomposites have concerned scientific and industrial attentiveness because nanoparticles (NP)s have significant surface effects, size effects, and quantum effects that improve material properties, including toughness, stiffness, transparency, scratch, abrasion, heat resistance, and gas permeability Citation[4].
Polyvinyl alcohol (PVA) is a polyhydroxy, water-soluble polymer that has been studied intensively because of its many pleasing characteristics, such as biocompatibility, biodegradability, and water solubility Citation[5]. PVA has been used for membranes, drug delivery system, and artificial biomedical devices Citation[6–8]. However, insufficient mechanical properties of PVA have restricted its wider applications. To improve its properties, the conventional method is to blend PVA with other materials like fillers. Among the different fillers structure, NPs, nanorods, and nanotubes showed considerable interests Citation[9,10]. Two methods can be used to prepare such nanocomposites materials: casting of films from a mixture of preformed nanomaterials and polymer or in situ formation of particles in the polymer matrices. In particular, PVA/metal and metal oxide nanocomposites are particularly promising materials Citation[11–13].
Titanium dioxide (TiO2) particles are promising candidates for photocatalytic material applications Citation[14–16]. Several attempts have been made to improve their photocatalytic activity. TiO2 photoactivity is strongly influenced by the presence of noble metals Citation[17]. Silver (Ag) has received attention in the context of disinfection since 1985. Further work on the field provides evidence of several physico-chemical phenomena of importance to interpret photokilling activity. On first place, silver has a well-known bactericidal capability by its own. Silver nanoclusters release Ag(0) and both chemical species Ag(I) ions and Ag(0) atoms, which have been shown to be effective in killing bacteria and fungi Citation[18–24]. Silver can also play roles associated to its presence at the TiO2 surface. This metal powerfully influences charge separation upon light absorption affecting in this way the photo-chemical activity. Besides that, silver can change the surface characteristics of TiO2 at local level. Some reports have been published in the field of photoactivity and photokilling ability of silver-loaded titanium dioxide (AT) nanocomposites Citation[25–28]. But till now, there are few reports on the morphological investigation of PVA/metal oxide nanocomposites Citation[29,30]. For instance, Peng and Kong Citation[30] prepared polyvinyl alcohol/silica (PVA/SiO2) nanocomposites by incorporating solution compounding and self-assembly technique and investigated the morphology of prepared nanocomposites with atomic force microscopy (AFM). The aim of this work was to prepare nanocomposites using PVA and AT NPs by using solution casting process. Also in the present study, we employ Fourier transform-infrared spectroscopy (FT-IR), ultraviolet–visible spectroscopy (UV–vis), AFM, field emission scanning electron microscopy (FE-SEM), and contact angle measurements to investigate the effect of NPs on the properties of the nanocomposites.
2 Experimental
2.1 Materials
All chemicals were purchased from Fluka Chemical Co. (Buchs, Switzerland), Aldrich Chemical Co. (Milwaukee, WI), and Merck Chemical Co. The PVA powder (Mw = 75,000 Da and degree of hydrolysis DH = 98%) was obtained from Fluka Chemical Co. and was used as received. The AT NPs was gained as a gift from Nano-Pac Persia Co. with average particle sizes of 40–60 nm according to the dynamic light scattering result.
2.2 Equipments
A FT-IR, Brucker, VECTOR 22 spectrometer was employed to examine the chemical bonds on the polymer and nanocomposites. Spectra of solids were obtained with KBr pellets. Vibration bands were reported as wavenumber (cm−1). The band intensities are assigned as weak (w), medium (m), strong (s), and broad (br). The dispersion morphology of the NPs on PVA matrix was observed using FE-SEM, HITACHI (S-4160). Transmission electron microscopy images were obtained using a Philips CM 120 microscope with an accelerating voltage of 100 kV. An UV–vis spectrophotometer (Cecil 5000 UV) was employed to gain the absorbance and transmittance behavior of the PVA and nanocomposite films in the wavelength range of 200–500 nm and the scan rate of 5 nm/min. The films for UV–vis absorbance measurements were organized by dividing films into pieces with a knife and were vacuum dried at 50 °C for 10 h. Contact angle measurements of prepared samples with water were measured by using contact angle meter (Phoenix 300) under an ambient condition at 25 °C in air according to Good and Girifaleo method.
2.3 AT nanoparticles synthesis
According to the supplier report, the following procedures were used to prepare the AT NPs. Titanium tetrachloride was used as the starting material to prepare pure TiO2 by sol–gel method and the preparation procedure and characterization methods were reported in pervious study Citation[31]. Silver nitrate was used as a silver source. The Ag immobilization onto the TiO2 NPs was reported based on the earlier work Citation[32].
2.4 Fabrication of AT/PVA nanocomposites films
In the first step, PVA powder was dissolved in distilled water (80 °C) under slight stirring for 2 h to acquire a PVA solution at a fixed concentration of 5 wt.%. Different amounts of the AT suspension (between 1 and 6 g) were well mixed with 20 g of the as-prepared PVA solution. The solid content of the AT suspension was measured gravimetrically to be about 2 wt.%. Distilled water was added into the mixture so that the total weight of the mixture was fixed at about 30 g. Table reviewed the composition of each of the as-prepared mixtures. The final concentrations of both PVA and AT in the mixtures were calculated and are also summarized in Table . The weight content of NPs with respect to the weight of PVA ranged between 0 and 2%. The AT/PVA mixtures were continuously stirred overnight prior to further fabrication. The resulting blends were spread in glass petri dishes. The globes were detached by trembling. The mixture was kept at room temperature until dried. The prepared nanocomposites were peeled out and dried at 80 °C under vacuum for 3 h.
Table 1. Composition of PVA solution and AT/PVA mixtures.
3 Results and discussion
3.1 AT NPs characterizations
The novel silver–TiO2 nanocomposite material was produced using an industrial UV reduction process. This process involves circulation of a solvent containing homogeneously dissolved compounds as the source of components for the synthesis of the AT material. A representative transmission electron micrograph of the AT nanostructure is shown in Figure . The nanomaterial consists of silver NPs embedded in a matrix of anatase titanium dioxide. The TiO2 fine structure consists of particles with an average diameter of approximately 6–8 nm. Silver NPs are located on the surface of the TiO2 and are also embedded within the matrix. This nanostructure can be used as antibacterial agent according to many reports Citation[33,34], which previously were demonstrated the mechanistic action of silver released of nanostructure AT.
Figure 1 Transmission electron micrograph showing a titanium dioxide NPs (gray structure) together with numerous supported silver NPs (dark spots).
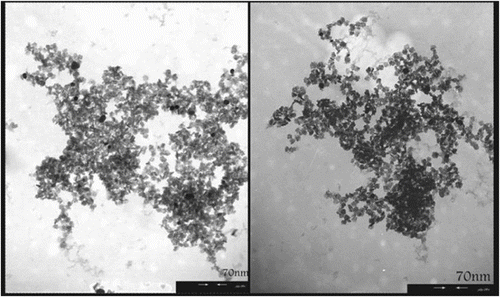
It can be concluded that, in contact with humidity, the silver particles perform as a source of silver ions, which stand for the active antimicrobial agent. Two key factors of the novel AT nanomaterials are the dispersion of the separate silver particles throughout the titania and the small diameter of the silver particles, which allows the material to be uniformly dispersed and eagerly incorporated into an assortment of substrates, including synthetic plastics, fibers, and other thin materials. The TiO2 in AT nanostructure material was acted as a suitable carrier for incorporation of the inorganic antimicrobial agents into plastics, textiles, and coatings. Therefore, these nanomaterials with respect to antimicrobial activity evidences were incorporated into or onto the PVA as polymeric matrix, and in the second step study the different optical and morphological properties of as-prepared nanocomposites.
3.2 Nanocomposite synthesis and characterizations
Nanocomposites were synthesized by casting solution process of PVA as matrix with AT nanomaterials in water (Scheme ). Different amounts of AT nanostructures were dispersed into PVA solution and the mixture was introduced into distilled water. After that, the resulting complex was collected and dried at room temperature (Table ).
The structure of PVA and the formation of PVA nanocomposites were confirmed by FT-IR analysis technique. Figure shows the plots of infrared spectra of the pure PVA, AT/PVA nanocomposites with different nano contents. The distinctive absorption bands of 3444, 2941, 1741, 1559, 1428, 1375, 1249, and 1094 cm−1 are attributed to the –OH stretching, C–H stretching, C–H bending, and C–O stretching of PVA. The pure PVA film has a broad and massive band centered at 3344 cm−1, which is consigned to the stretching vibration of hydroxyl units with strong intra-hydrogen bonding, while that of the AT/PVA nanocomposite is shifted to 3345 cm−1, and its shape becomes broadened. This may be due to the interaction between the AT NPs and the PVA matrix, which increased the bond formation. In the FT-IR spectra of AT/PVA nanocomposites, the characteristic absorbing peaks of TiO2 are appeared around 450–800 cm−1, which can be ascribed to the vibration of Ti–O–Ti groups. This evidence indicates that the PVA matrix and AT NPs are not simply blended, and complex interactions may exist. Due to the hydrogen bond, short-ranged steric, electrical interaction and also some covalent bonds between AT nanostructures and functional groups of PVA, the dispersing of nonmaterial in the structure of nanocomposites can be expected to be homogeneous.
3.3 Morphological study
3.3.1 AFM studies
AFM is an amazing system that allows us to observe and compute surface construction with exceptional resolution and accuracy. AFM has a gigantic benefit, in that almost any sample can be imaged to be very hard, such as the surface of a ceramic material, or a dispersion of metallic NPs, or extremely soft, such as greatly plastic materials, or discrete molecules of proteins. An AFM image provides information about surface morphologies of AT/PVA nanocomposite by using the two- and three-dimensions. AFM images were taken with 20 μm × 20 μm scan area from the illustrations. Figures and show the two- and three-dimension AFM images of AT/PVA (N3) nanocomposite. No major concision area in size is observed in the pictures. From three-dimensional 20 μm × 20 μm outline, it comes out that the gained nanocomposite shows an interrupted structure with a superior exterior planarity. In the surface organization of the coat, AT NPs with a size less than 50 nm were observed clearly.
3.3.2 Height distribution, mean, and roughness investigations
In the width of 20 μm, six singular entries for N3 nanocomposite were chosen with different spans. The unsymmetrical allocations of peaks and valleys from the central point in graph (b) of Figure for nanocomposite N3 were related to the residual of AT NPs in outsides, but in the most sites it demonstrated analogous patterns of peaks and valleys. As stated by ISO 4287-1997, the average deviation of total point’s roughness profile from a mean line above the evaluation length (roughness average) was estimated to be 2 nm. The height of the highest peak in the roughness profile over the appraisal length (Rp) was calculated as 11 nm. The difference between the highest peak and the lowest valley within the multiple models in the evaluation length (Rtm) was calculated and shown in Table .
Figure 5 Selected zones from two-dimensional AFM image (a) and the height profiles related to measuring length (b).
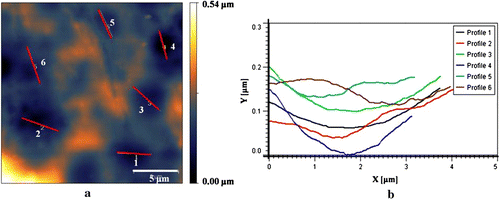
Table 2. Roughness parameters for AT/PVA nanocomposites.
3.3.3 Application of AFM in determining the fractal dimension
Fractal geometry can be used to quantitatively explain irregular shapes and surfaces Citation[35]. The fundamental quantitative component of fractal geometry, the fractal dimension D, is a noninteger number with a Euclidean and nonEuclidean component. Several methods have been used to measure the D of a fracture surface. The measurement of the outline of the fracture surface is one of the most frequently used methods. To calculate D from the AFM data, in this study a software Gwyddion package was used. Gwyddion uses four methods to calculate D from AFM data: (1) cube counting, (2) triangulation, (3) variance, and 4) power spectrum distribution. The obtained results for the N3 distribution profiles were 2.21, 2.24, 2.28, and 2.22, respectively. Figure showed the cube counting and power spectrum graphs for nanocomposites N3.
3.3.4 FE-SEM analysis of nanocomposites
The homogeneous dispersion of the NPs in the matrix is very crucial to improve the property of the nanocomposites. To well-disperse the AT nanostructures into the PVA matrix, homogeneous AT NPs was first prepared in water and then was blended with water-soluble PVA. The AT/PVA nanocomposites were obtained and the morphology and structure of the as-prepared products were probed by FE-SEM.
The surface morphology at the micrometer and nanometer length scale of the neat PVA, AT containing PVA nanocomposites is observed using FE-SEM micrographs of the samples (). Microstructure surface morphology of the neat PVA matrix was investigated by using SEM and reported by Mallakpour and Barati Citation[36]. For AT NPs, the FE-SEM results show that the sample composes of spherical crystallites with smooth exteriors in the size range of 40–80 nm. These NPs are in uniform state due to the stabilization effect.
With the addition of AT NPs, the surface microstructure morphologies of the samples are changed. Clearly, smoother surface morphology at the micrometer length scale is observed in the neat PVA. The micrograph of AT/PVA samples with different AT contents has been shown in Figure . It was became visible uniform, dense, without any momentous domains of aggregated particles. The smallest specific particles are predictable to be ca. 40 nm. The FE-SEM images demonstrated that the NPs were well thought-out and homogeneously dispersed. It is implicit that the created networks between inorganic and organic moieties with physical and/or chemical bonds caused to fabricated materials that can be considered as homogeneous composites. Hydroxyl groups of PVA can form strong hydrogen bonds with functional groups present on AT NPs surfaces. As a consequence, hydrogen bonds should enhance the interfacial interaction between PVA and AT nanostructures, which favored property improvements with AT NPs as the reinforcement nanofiller.
3.4 Optical characterization
Figure shows the UV–visible absorption spectra of PVA and AT/PVA nanocomposite thin films. The nanocomposite films containing AT NPs absorb UV light starting at around 372 nm down to 250 nm and maximum absorption is found to be at 250 nm. There is considerable increase in the absorption intensity of the nanocomposite films compared to neat PVA film. The UV–visible spectra of PVA and AT/PVA nanocomposite thin films for five different concentrations of AT NPs are shown in Figure . The PVA film does not absorb UV light, while the PVA nanocomposite films containing AT NPs absorb UV light starting at around 360 nm, down to 250 nm. All signals come to maximum absorption in the UV region regardless of the AT content, which shows that absorption is autonomous of the AT content added.
3.5 Contact angle measurements
The wetting conduct between a liquid and a discrete film is dependent, at least in part, upon the closeness of the surface energy of the liquid and the surface energy of the substrate. The multifarious interaction between a liquid and a surface may be considered as a mixture of three different processes of wetting, absorption, and adsorption. In this study, wetting is best clarified with a drop of water on a film surface. Figure (a–d) shows representative images of water droplets on the films (PVA, N2, N3, and N5), which indicating hydrophilicity against water. The contact angle of PVA and prepared AT/PVA nanocomposite films (N2, N3, and N5) for water were 68°, 64°, 58°, and 54°, respectively, which demonstrating sufficient hydrophilicity. The water droplets instantly penetrated through the polymeric films.
4 Conclusion
A series of AT/PVA nanocomposites were prepared by effective dispersion of the inorganic AT NPs into PVA matrix, via solvent casting technique. The developed nanocomposites were studied by an assortment of microscopic and macroscopic methods. Morphological investigations using FE-SEM proved the outstanding dispersion of the AT NPs into the PVA matrix. Alternatively, AFM revealed the specific locations in the dispersions of the AT NPs to the surface of the nanocomposite. The data obtained from FT-IR spectroscopy were shown the existence of characteristic absorption bands related to polymer and AT NPs in the nanocomposite structures. The studies of nanocomposites by using UV revealed that the existence of the AT NPs was increased the shield properties against UV light. Bolstering of PVA with AT nano fillers increase the physical and chemical properties of the prepared films and expand the applications of them. This hybrid material can be used in different industrial fields such as packaging industry.
Acknowledgments
We wish to express our thankfulness to the Research Affairs Division Mazandaran University, Babolsar, for partial financial support. Further financial support from National Elite Foundation (NEF), Iran Nanotechnology Initiative Council (INIC) is appreciatively acknowledged.
References
- Sellers K, Mackay Ch, Bergeson LL, Clough SR, Hoyt M, Chen J, Henry K, Hamblen J. Nanotechnology and the environment. Boca Raton (FL): CRC Press, Taylor & Francis Group; 2009.
- Merhari , L . 2009 . Hybrid nanocomposites for nanotechnology , New York , NY : Springer .
- Mai , YW and Yu , ZZ . 2006 . Polymer nanocomposites , Cambridge , , UK : Woodhead .
- Mallakpour , S and Hatami , M . 2011 . Des. Monomers Polym. , 14 : 461
- Goyal , R , Tripathi , SK , Vazquez , E , Kumar , P and Gupta , KC . 2012 . Biodegradable poly(vinyl alcohol)–polyethylenimine nanocomposites for enhanced gene expression in vitro and in vivo . Biomacromolecules. , 13 : 73 – 83 .
- Tang , E , Tian , B , Zheng , E , Fu , C and Cheng , G . 2008 . Preparation of zinc oxide nanoparticle via uniform precipitation method and its surface modification by methacryloxypropyltrimethoxysilane . Chem. Eng. Commun. , 195 : 479 – 491 .
- Abbasi , Z . 2012 . Water resistance, weight loss and enzymatic degradation of blends starch/polyvinyl alcohol containing SiO2 nanoparticle . J. Taiwan Inst. Chem. , 43 : 264 – 268 .
- Killeen , D , Frydrych , M and Chen , B . 2012 . Porous poly(vinyl alcohol)/sepiolite bone scaffolds: preparation, structure and mechanical properties . Mater. Sci. Eng. C. , 32 : 749 – 757 .
- Gaume , J , Rivaton , A , Thérias , S and Gardette , J-L . 2012 . Influence of nanoclays on the photochemical behaviour of poly(vinyl alcohol) . Polym. Degrad. Stab. , 97 : 488 – 495 .
- Gaume , J , Taviot-Gueho , C , Cros , S , Rivaton , A , Thérias , S and Gardette , J-L . 2012 . Optimization of PVA clay nanocomposite for ultra-barrier multilayer encapsulation of organic solar cells . Sol. Energy Mater. Sol. , 99 : 240 – 249 .
- Maiti , J , Kakati , N , Lee , SH , Jee , SH and Yoon , YS . 2011 . PVA nano composite membrane for DMFC application . Solid State Ionics. , 201 : 21 – 26 .
- Kokabi , M , Sirousazar , M and Muhammad Hassan , Z . 2007 . PVA–clay nanocomposite hydrogels for wound dressing . Eur. Polym. J. , 43 : 773 – 781 .
- Junkasem , J , Rujiravanit , R and Supaphol , P . 2006 . Fabrication of α-chitin whisker-reinforced poly(vinyl alcohol) nanocomposite nanofibres by electrospinning . Nanotechnology. , 17 : 4519 – 4528 .
- Lingaiah , S , Sadler , R , Ibeh , C and Shivakumar , K . 2008 . A method of visualization of inorganic nanoparticles dispersion in nanocomposites . Composites Part B. , 39 : 196 – 201 .
- Hashimoto , M , Takadama , H , Mizuno , M and Kokubo , T . 2006 . Enhancement of mechanical strength of TiO2/high-density polyethylene composites for bone repair with silane-coupling treatment . Mater. Res. Bull. , 41 : 515 – 524 .
- Zhang , F , Shi , ZL , Chua , PH , Kang , ET and Neoh , KG . 2007 . Functionalization of titanium surfaces via controlled living radical polymerization: from antibacterial surface to surface for osteoblast adhesion . Ind. Eng. Chem. Res. , 46 : 9077 – 9086 .
- Choi , W , Termin , A and Hoffmann , MR . 1994 . The role of metal ion dopants in quantum-sized TiO2: correlation between photoreactivity and charge carrier recombination dynamics . J. Phys. Chem. , 98 : 13669 – 13679 .
- Rai , M , Yadav , A and Gade , A . 2009 . Silver nanoparticles as a new generation of antimicrobials . Biotechnol. Adv. , 27 : 76 – 83 .
- Petica , A , Gavriliu , S , Lungua , M , Buruntea , N and Panzaru , C . 2008 . Colloidal silver solutions with antimicrobial properties . Mater. Sci. Eng. B: Solid. , 152 : 22 – 27 .
- Raffi , M , Hussain , F , Bhatti , TM , Akhter , JI , Hameed , A and Hasan , MM . 2008 . Antibacterial characterization of silver nanoparticles against E. coli ATCC-15224 . J. Mater. Sci. Technol. , 24 : 192 – 196 .
- Singh , G , Patankar , RB and Gupta , VK . 2010 . The preparation of polymer/silver nanocomposites and application as an antibacterial material . Polym. Plast. Technol. Eng. , 49 : 1329 – 1333 .
- Rathnayake , I , Ismail , H , Azahari , B , Darsanasiri , ND and Rajapakse , S . 2012 . Synthesis and characterization of nano-silver incorporated natural rubber latex foam . Polym. Plast. Technol. Eng. , 51 : 605 – 611 .
- Jokar , M , Abdul Rahman , R and Ibrahim , NA . 2012 . Layer by layer deposition of polyethylene glycol capped silver nanoparticles/chitosan on polyethylene substrate . Int. J. Polym. Mater. , 61 : 371 – 383 .
- Bajpai , SK , Bajpai , M and Sharma , L . 2011 . In situ formation of silver nanoparticles in poly(n-isopropyl acrylamide) hydrogel for antibacterial applications . Des. Monomers Polym. , 14 : 383 – 394 .
- Chang , C-C , Lin , C-K , Chan , C-C , Hsu , C-S and Chen , C-Y . 2006 . Photocatalytic properties of nanocrystalline TiO2 thin film with Ag additions . Thin Solid Films. , 494 : 274 – 278 .
- Tatsuma , T and Suzuki , K . 2007 . Photoelectrochromic cell with a Ag–TiO2 nanocomposite: concepts of drawing and display modes . Electrochem. Commun. , 9 : 574 – 576 .
- Cheng , Q , Li , C , Pavlinek , V , Saha , P and Wang , H . 2006 . Surface-modified antibacterial TiO2/Ag+ nanoparticles: preparation and properties . Appl. Surf. Sci. , 252 : 4154 – 4160 .
- Clemenson , S , David , L and Espuche , E . 2007 . Structure and morphology of nanocomposite films prepared from polyvinyl alcohol and silver nitrate: influence of thermal treatment . J. Polym. Sci. , A1 : 2657 – 2672 .
- Paiva , MC , Zhou , B , Fernando , KAS , Lin , Y , Kennedy , JM and Sun , Y-P . 2004 . Mechanical and morphological characterization of polymer–carbon nanocomposites from functionalized carbon nanotubes . Carbon. , 42 : 2849 – 2854 .
- Peng , Z and Kong , LX . 2007 . Morphology of self-assembled polyvinyl alcohol/silica nanocomposites studied with atomic force microscopy . Polym. Bull. , 59 : 207 – 216 .
- Marandi , R , Khosravi , M , Olya , ME , Vahid , B and Hatami , M . 2011 . Photocatalytic degradation of an azo dye using immobilised TiO2 nanoparticles on polyester support: central composite design approach . Micro Nano Lett. , 6 : 958 – 962 .
- Rodrguez-Gonzlez , V , Obregn Alfaro , S , Torres-Martnez , LM , Chod , S-H and Leed , S-W . 2010 . Silver–TiO2 nanocomposites: synthesis and harmful algae bloom UV-photoelimination . Appl. Catal. B: Environ. , 98 : 229 – 234 .
- Hou , X-G , Huang , M-D , Wu , X-L and Liu , A-D . 2009 . Preparation and studies of photocatalytic silver-loaded TiO2 films by hybrid sol–gel method . Chem. Eng. J. , 146 : 42 – 48 .
- Dunnill , CW , Noimark , S and Parkin , IP . 2012 . Silver loaded WO3−x/TiO2 composite multifunctional thin films . Thin Solid Films. , 520 : 5516 – 5520 .
- Eaton , P and West , P . 2010 . Atomic force microscopy , New York , NY : Oxford University Press .
- Mallakpour , S and Barati , A . 2011 . Efficient preparation of hybrid nanocomposite coatings based on poly(vinyl alcohol) and silane coupling agent modified TiO2 nanoparticles . Prog. Org. Coat. , 71 : 391 – 398 .