Abstract
The present study deals with the encapsulation of vegetable organogels in alginate microparticles (MPs). Mango butter (MB) and cocoa butter (CB) are natural organogels, which are being used in the preparation of pharmaceutical formulations. In addition to these, mustard oil (MO) was used as the control. The thermal stability of MB and CB can be enhanced by encapsulating them and can be used in oral drug-delivery formulations. Double emulsification method was followed for the preparation of MPs. By this method, spherical MPs were synthesized and the sphericity was confirmed by doing light microscopy and scanning electron microscopy. The size range of MPs was 107 ± 53 μm, 79 ± 43 μm, and 112 ± 78 μm for MPs encapsulating MO, MB, and CB, respectively. The melting endotherms of MPs with MB and CB were in exact match with the melting points of MB and CB. This confirmed the existence of MB and CB within the MPs. The encapsulation of the vegetable organogels altered the release pattern of the drug, metronidazole. The drug release kinetics suggested that the release pattern followed the non-Fickian kinetics. The MPs were found to be hemocompatible in nature and have shown good mucoadhesive and swelling properties. Based on the preliminary results, it was suggested that the vegetable organogels can be encapsulated in alginate MPs and used as drug-delivery vehicles.
1. Introduction
Since long, there has been an increasing trend of encapsulating bioactive agents within polymeric materials Citation[1–3]. This was done either to protect the bioactive agents from the environmental factors or to prevent the damaging effect of the bioactive agent to the tissues Citation[4,5]. For example, proteins (e.g. insulin) Citation[6–8], vaccines (e.g. tetanus toxoid) Citation[9], and antibiotics (e.g. gentamicin) Citation[10,11] get degraded in the stomach while non-steroidal anti-inflammatory drugs (e.g. aspirin) Citation[12,13] cause irritation of the gastric mucosa which might result in the ulceration Citation[14]. Microencapsulation of the bioactive agents in microparticles (MPs) has also been extensively used for masking the nauseous taste and odor of the lipophilic bioactive agents Citation[15,16]. Unfortunately, as the time progresses, the liquid lipophilic bioactive agents have a tendency to leach out the MPs. This hampers the masking effect of MPs and hence limits the shelf-life of the encapsulated particles Citation[17].
Cocoa butter (CB) and mango butter (MB) are vegetable fats of plant origin and are often categorized as plant-derived organogels Citation[18]. The main constituents of CB include high quantities of saturated fats derived from stearic and palmitic acids and are usually referred as theobroma oil Citation[19,20]. The triglycerides of the CB usually composed of stearic, palmitic and oleic acids Citation[21]. Composition of the MB is similar to CB Citation[22]. 80% of the MB composition consists of stearic and oleic acids. It also consists of palmitic and linoleic acids Citation[22]. They are solid at room-temperature (RT) but converts into an apolar sol at ∼38–39 °C, depending on the source of extraction Citation[23]. These fats are being extensively used in various food, cosmetics, and pharmaceutical industries due to their beneficial effects Citation[24,25].
Taking inspiration from the above, it seems quite feasible to develop vegetable fat-based MPs which may prevent the leaching of the internal phase. Hence, an attempt was made to develop alginate MPs encapsulating mustard oil (MO), CB, and MB as internal apolar phase. MO was used as a representative apolar liquid. The particles were characterized for their properties with a special emphasis on the leaching of the apolar internal phase.
2.1 Materials
Sodium alginate, calcium chloride (fused), and dialysis tubing (MW cut-off 12 kDa) were purchased from Himedia, Mumbai, India. Calcium carbonate and glacial acetic acid were purchased from MERCK, Mumbai, India and Nice Chemicals (P) Ltd, Cochin, India, respectively. Span 80 (SN) and Tween 80 (TW) were purchased from Lobachemi, Mumbai, India. Sunflower oil (SO) and MO were purchased from the local market. CB and MB were procured from NV Organics (P) Ltd, New Delhi, India. Fresh goat blood and small intestine were procured from local butcher shop. Double distilled water (DDW) was used throughout the study.
2.2 Preparation of microparticles
The MPs were prepared by modified double-emulsion method as described elsewhere Citation[26]. The details of the method are described in short. 0.5 g of sodium alginate was dissolved in 20 ml of water, kept on stirring at 250 rpm using an over-head stirrer at 50 °C, to form alginate sol. 0.15 g of calcium carbonate was dispersed in the alginate sol. The dispersion, so formed, was stirred for 15 min followed by the addition of 0.5 ml of span 80. 5 g of MO or CB or MB, maintained at 50 °C, was added to the alginate sol with constant stirring to form primary emulsion. The primary emulsion was cooled in an ice-bath with constant stirring for 10 min, which increased the thickness of the primary emulsion. The thickened primary emulsion was then slowly transferred to the 100 ml of SO (external phase), kept on stirring at 250 rpm and maintained at 0 °C, so as to form a double emulsion. This was followed by the addition of 6 ml of acidified oil (5.5 ml of SO + 0.5 ml glacial acetic acid) which resulted in the release of calcium ions from the calcium carbonate in the alginate sol layer. The release of the calcium ions resulted in the crosslinking of the alginate molecules by ionotropic method. Subsequently, 0.5 M calcium chloride solution was added to the above mixture to consolidate the alginate particles. The MPs, so obtained, were then filtered out and washed with 1% (v/v) TW solution followed by washing with distilled water. Drug-containing MPs were prepared in a similar manner except that the internal phase contained 2% (w/v) metronidazole (MZ) in the internal apolar phase. The MPs were then dispersed in 50 ml of water and stored under refrigerated conditions for further use. The MO-, CB-, and MB-containing MPs were regarded as MOP, CBP, and MBP, respectively, while the drug-containing MPs were regarded as MOD, CBD, and MBD, respectively.
2.3 Optical microscopy
The MPs, equilibrated in water, were observed under an upright bright-field compound microscope (LEICA-DM 750 equipped with ICC 50-HD camera, Germany) to analyze the size distribution and to understand the microstructure of the particles formed. The size distribution of the MPs was found out by automated particle size analysis by image processing. NI Vision Assistant-2010 software was used for the analysis. The measurements were done at RT, 25 °C.
2.4 Confocal laser scanning microscopy
Fresh vegetable fat-based MPs were observed under confocal laser scanning microscope (FV1000 confocal microscope, Olympus, USA). The MPs samples were prepared in a similar way as described above except that the CB and MB contained 0.1% (w/w) fluorol yellow 088 (FY). The chromophore was excited at 405 nm and the fluorescence at 515 nm was observed Citation[27]. The measurements were done at RT.
2.5 Scanning electron microscopy
The MPs were dried for 6 h at 40 °C in hot air oven. The dried MPs were coated with a platinum layer by sputter coating technique and were subsequently analyzed under scanning electron microscope (JEOL, JSM-6390, Japan).
2.6 XRD analysis
The MPs were subjected to X-ray diffraction (PW3040, Philips Analytical Ltd, Holland) using monochromatic Cu K α radiation (λ = 0.154 nm) in the range of 5° to 50°2θ. The scanning rate was 2° 2θ/min. The measurements were done at RT.
2.7 Thermal studies
Thermal properties of the MO, CB, MB, MOP, MBP, and CBP were analyzed using a differential scanning calorimeter (DSC-200 F3 MAIA, Netzsch, Germany). The samples were analyzed in the temperature range of 30 to 120 °C at a scan rate of 2 °C/min. The analysis was done under nitrogen atmosphere in a pierced aluminum pan.
2.8 Leaching studies
About 0.25 g of dried MPs was kept on a filter paper. Any leakage of the internal phase was monitored after 2 h Citation[28]. The measurements were done at RT.
In a separate study, accurately weighed 1 g of the MPs (whose surface moisture was removed by blotting paper) were kept on a filter paper Citation[29]. The MPs were incubated at 37 ± 1 °C. The weight of the MPs was determined. The loss in the weight of the MPs was accounted to the leaching of the internal phase. The moisture loss from the MPs was compensated by treating the blank alginate MPs in a similar manner. The results have been reported as percent leaching (%L) given by the formula:
where, W f = weight of test MP at time ‘t’, W i = initial weight of test sample.
2.9 Swelling studies
The swelling behavior of the MPs was studied at an interval of 2 min. Accurately weighed 0.1 g (W i) of dried MPs of MOP, MBP, and CBP were immersed in 50 ml of distilled water. At regular intervals of 2 min, the MPs were filtered out and weighed after removing the surface moisture using Whatmann paper (Wt ). The study was conducted for 10 min. The % swelling of MPs was calculated from the equation 1 Citation[30,31]. The measurements were done at RT.
where, Wt = weight of MPs after time t, W i = initial weight of MPs.
2.10 In vitro drug release studies
MZ-loaded samples were used to understand the release kinetics of the drug from the MPs. About 5 g of dried MPs dispersed in 10 ml of water were weighed accurately and put in dialysis membrane bag. The dialysis bag was subsequently submerged in 50 ml of distilled water (pH = 7.0 ± 0.2), kept on stirring at 100 rpm at 37 °C. The dissolution medium was replaced with fresh distilled water at regular intervals of time, 15 min for the first hour and 30 min for the remaining time to ensure sink conditions. The samples were then analyzed at 321 nm using UV–Visible double beam spectrophotometer (UV–3200, LabIndia, India).
2.11 Mucoadhesive studies
The mucoadhesive property of the MPs was analyzed by using modified USP disintegration method as described elsewhere Citation[32]. In short, a piece of the intestinal lumen of goat was cut open and attached to glass slides using acrylate adhesive. 0.5 g of each sample was weighed and was put on the exposed surface of the mucosa. A 5 g weight was applied on the samples for 5 min. Then, the slides were put into USP disintegration baskets vertically. The disintegration medium used was phosphate buffer (pH = 7.2). The experiment was carried out for 24 h. The measurements were done at 37 ± 1 °C.
2.12 Hemocompatibility studies
The MPs were analyzed for hemolysis by incubating them in the presence of goat blood at 37 ± 1 °C, as described elsewhere Citation[30]. The method calculates the extent of hemolysis in the presence of the samples. The % hemolysis for each sample was calculated using the following equation:
where,
ODtest = optical density for the test sample,
ODpositive = optical density for the positive control,
ODnegative = optical density for the negative control.
3 Results and discussion
3.1 Light microscopic evaluation of MPs
The micrographs of MPs have been shown in Figure . The particles were found to be circular in nature having different sizes. The particle size distribution was found by automated particle size distribution analysis using NI Vision 2010 software. The particle size distribution was figured out by the following algorithm (Figure ). The image was first calibrated for the real-world measurement. After the calibration of the image was done, the intensity plane of the image was extracted out followed by thresholding of the image, which resulted in segmenting out the particles from the background. Thresholding incorporated some noise in the image and were subsequently removed by using the remove small objects function. The resultant image contained the segmented particles. A Heywood circularity particle filter was used to select near circular particles. Convex Hull function allowed closing of unclosed or irregularly segmented particles. The border particles were removed before the particle analysis. The results were exported in an excel file. The particle size distribution and cumulative particle size distribution were calculated and are shown in , respectively. The particle size distribution indicated that the MPs encapsulating MO and MB showed a Gaussian distribution of the particles, which is often associated with the particles prepared by physical methods (Figure ). The MOP showed a narrow distribution of the particles having maximum particles of 150 μm (approx) as compared to the MBP which showed maximum particles of 50 μm (approx). The size distribution of the CBP indicated bimodal size distribution having highest number of particles at 60 μm (approx) followed by particles of size 210 μm (approx) (Figure ). The cumulative particle size distribution showed a sigmoid distribution (Figure ). The results suggested that the 50% of the particles of MOP, MBP, and CBP were having sizes of 109 μm, 68 μm, and 92 μm, respectively. The results were in close proximity of the particle size calculated by averaging the particle sizes (Table ).
Figure 2 Micrographs at different stages of image processing. (a) Original Image, (b) thresholded image after image calibration and color intensity plane extraction, (c) remove small objects, (d) separate objects, (e) Heywood circularity particle filter, (f) Convex Hull, (g) fill holes, and (h) remove border objects.
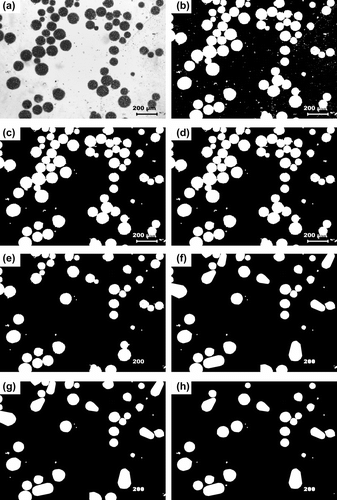
Table 1. Size distribution of the MPs.
3.2 Confocal laser scanning microscopy
The CLSM images indicate that the MB and CB were encapsulated within the MPs (Figure ). Bright yellow color of MPs was due to the fluorescent activity of FY chromophore, dissolved within MB and CB. The micrographs suggested that MB and CB were successfully encapsulated within the alginate particles.
3.3 SEM analysis
The micrographs obtained from SEM suggested that the particles were spherical in shape as was also evident from the micrographs from the light microscope (Figure ). The sizes of MOP, MBP, and CBP MPs were found to be around ∼120, ∼80, and ∼130 μm, respectively. The observed sizes of MPs under SEM were almost similar to the MPs when observed under light microscope. It was identified that the surface morphology of the MPs was not smooth.
3.4 XRD analysis
The X-ray diffractograms of the MPs have been shown in Figure . The MPs were found to be amorphous in nature as there were no sharp peaks. The amorphousness was calculated from the full width half maximum (FWHM) value, of the diffractograms were in the order of CBP < MBP < MOP (Table ) Citation[33]. As MO was encapsulated within the MOP, presence of isotropic liquid might has enhanced the amorphousness of the MOP Citation[34,35]. Also, the shift of the peak toward the right-hand side supported the increase in the amorphous nature of the MOP as compared to CBP and MBP Citation[36]. This variation in the physical nature has influenced the drug release pattern from the MPs.
Table 2. Peak position and FWHM values of the MPs, deduced from the X-ray diffractograms.
3.5 Thermal studies
The DSC thermograms of CB, MB, and MPs have been shown in Figure . The CB and MB showed endothermic peaks at ∼38.4 °C. The DSC thermograms of both CBP and MBP also showed endothermic peaks at ∼38 °C. The melting temperature (T m) associated with the CBP and MBP may be associated to the CB and MB present within the alginate MPs. This suggested that the CB and MB were successfully encapsulated within the CBP and MBP, respectively. The thermogram of MOP showed a broad endotherm peak starting at 28 °C and extending up to 90 °C with an endothermic peak at 60.9 °C. Broad endothermic peak in MOP may be due to the diffusion of aqueous phase into MO during the preparation of the MOP. The endothermic peak corresponds to the loss of moisture from the MO. Table shows the T m, change in enthalpy during melting (ΔH m) and change in entropy during melting (ΔS m) values of the samples. High ΔH m and ΔS m values for MOPs were due to the loss of moisture over a wide range of temperature, whereas MBP and CBP showed much lower ΔH m and ΔS m.
Table 3. DSC data of the samples.
3.6 Leaching studies
The MPs were subjected to leaching studies to determine the leakage of the internal phase. The results of the study have been shown in . The study showed that the %L in MOP was 40.18% at the end of 3 h. Thereafter, there was no significant change in the weight loss and hence %L. MBP and CBP did not show any reduction in weights associated with the loss of the internal phase under the experimental conditions. Surface moisture loss was observed in all the MPs and was compensated by considering blank MPs as the reference. The results suggested that the MO was leaching from the MPs while there was no leaching of the internal phase from the CBP and MBP. This may be attributed to the fact that MO is liquid at RT. As the MPs are being dried, the gelled structure start shrinking thereby increases the pressure on the inner liquid core. The liquid core is not able to oppose the inward pressure exerted by the alginate layer. This, in turn, results in the leaching of the liquid core. When the inner core is solid in nature as in CBP and MBP, the inner core maintains its physical structure and opposes the pressure exerted by the alginate layer Citation[37]. This phenomenon does not allow leaching of CB and MB from the gelled structure of the alginate. The results indicated that encapsulation of organogels may offer a better way to encapsulate bioactive agents.
3.7 Swelling studies
The swelling behavior of the MPs at RT has been shown in Figure . MBP and CBP have shown 80% increase in weight compared to initial weight whereas; MOP had shown only 60%. The maximum% swelling was achieved after 5 min of incubation. No change in the absolute weight of the samples was observed on further incubation. The results suggested that quick swelling of the MPs which may be attributed to ready diffusion of water into the alginate layer, which in turn, might allow the release of the drug instantaneously Citation[38,39].
3.8 In vitro drug release studies
The cumulative percentage drug release (CPDR) of MPs over 7 h of study have been shown in Figure . The cumulative release of the drug from MOD, CBD, and MBD MPs was found to be ∼85, ∼43, and ∼24%, respectively. The higher drug release from the MOD MPs might be accounted to the quick partitioning of the drug from MO to the surrounding aqueous gelled layer. Also, the XRD study suggested increased amorphous nature of the alginate layer when MO was the internal phase Citation[34]. Hence, the diffusion of the drug from the alginate layer to the dissolution media was quicker. The lower CPDR values of MBD and CBD can be related to the combined effect of the lower partitioning of the drug from the inner core to the alginate layer and slower diffusion of the drug from the alginate layer due to the presence of slightly higher ordered structure as compared to the MOD. The drug release from the three samples followed the Higuchian kinetics (Figures and 14, Table ). The n value of the Krossmeyer–Peppas (KP) model gives information on the mechanism of drug release from the formulations. The results suggested that the release of the drug from the MPs followed non-Fickian kinetics (n ≈ 0.6) (Figure and Table ) Citation[40].
Table 4. R 2 values from model fitting of drug-loaded samples.
Table 5. Higuchian and Fickian constants for MOD, MBD, and CBD.
3.9 Mucoadhesive studies
Mucoadhesive properties of the MPs were analyzed by in vitro wash-off method by modified USP disintegration test method. The time required for MPs to detach from the mucosal surface was determined. The results showed that detachment of the MOP, MBP, and CBPMPs occurred at 148 ± 10, 292 ± 25, and 348 ± 21 min, respectively. The mucoadhesion was higher in MPs containing vegetable fats as compared to the MO. The results may be explained by the leakage of the internal phase from the MPs. The lower mucoadhesive property of the MOP MPs might be due to the continuous leaching of the MO from the particles. The presence of oil on the surface of the MOP MPs might have limited the mucoadhesion properties of the MPs. As there was no leakage from the MBP and CBP MPs, these MPs have shown higher residence time upon the mucous membrane. The results suggested that MBP and CBP formulations have better mucoadhesivity than MOP which may be attributed to the cessation of the leaching of the inner core phase.
3.10 Hemocompatibility studies
The results of hemocompatiblity study of the samples have been tabulated in Table . The results indicated that the MOP, MBP and CBP MPs were found to be highly hemocompatible in nature as the % hemolysis was <5%.Citation[41] Based on the preliminary studies, the MPs may be regarded as biocompatible in nature.
Table 6. Hemocompatibility test for MPs.
4 Conclusion
The present study confirms that encapsulation of vegetable organogels in alginate MPs was quite feasible by double emulsification method. The light microscopic and SEM studies have shown the formation of spherical MPs. The observed size ranges of MOD, CBD, and MBD MPs were 109 ± 53, 68 ± 43, and 92 ± 78 μm, respectively. The encapsulation of MB and CB were confirmed by performing DSC studies. The DSC thermograms have shown endothermic peaks corresponding to the MB and CB in MBP and CBP MPs, respectively. The differences in the amorphousness of MPs affected their CPDR. The drug release kinetics suggested that the drug release was following Higuchian kinetics with non-Fickian diffusion. The reduction in the leakage of the internal phase (MB and CB) suggested that the MBP and CBP MPs may be stored for longer periods of time. This eventually might improve the stability of the MB and CB during storage and transport. The MPs were found to be hemocompatible in nature and have shown good mucoadhesive properties. Based on the preliminary results, it can be concluded that the encapsulated MB and CB may be used as the drug-delivery vehicles.
Acknowledgments
The authors acknowledge the instrumental facilities provided by National Institute of Technology, Rourkela (NIT-R), India and Indian Institute of Technology, Kharagpur (IIT-KGP), India. The leading author acknowledges the scholarship provided by National Institute of Technology, Rourkela (NIT-R), India, for the completion of the PhD degree. Funds leveraged from the project (BT/PR14282/PID/06/598/2010) sanctioned by the Department of Biotechnology, Govt. of India is hereby acknowledged.
Reference
- Jabbal Gill , I , Fisher , AN , Hinchcliffe , M , Whetstone , J , Farraj , N , De Ponti , R and Illum , L . 1994 . Cyclodextrins as protection agents against enhancer damage in nasal delivery systems II: effect on in vivo absorption of insulin and histopathology of nasal membrane . Eur. J. Pharm. Sci. , 1 : 237 – 248 .
- Chang , Y-C and Chu , IM . 2008 . Methoxy poly(ethylene glycol)-b-poly(valerolactone) diblock polymeric micelles for enhanced encapsulation and protection of camptothecin . Eur. Polym. J. , 44 : 3922 – 3930 .
- Fonseca , LSD , Silveira , RD , Deboni , AM , Benvenutti , EV , Costa , TMH , Guterres , SS and Pohlmann , AR . 2008 . Nanocapsule@xerogel microparticles containing sodium diclofenac: a new strategy to control the release of drugs . Int. J. Pharm. , 358 : 292 – 295 .
- Soehngen , EC , Godin-Ostro , E , Fielder , FG , Ginsberg , RS , Slusher , MA and Weiner , AL . 1988 . Encapsulation of indomethacin in liposomes provides protection against both gastric and intestinal ulceration when orally administered to rats . Arthritis Rheum. , 31 : 414 – 422 .
- Felnerova , D , Viret , JF , Glück , R and Moser , C . 2004 . Liposomes and virosomes as delivery systems for antigens, nucleic acids and drugs . Curr. Opin. Biotechnol. , 15 : 518 – 529 .
- Xie , J and Wang , CH . 2007 . Encapsulation of proteins in biodegradable polymeric microparticles using electrospray in the Taylor cone-jet mode . Biotechnol. Bioeng. , 97 : 1278 – 1290 .
- Rosa , GD , Iommelli , R , La Rotonda , MI , Miro , A and Quaglia , F . 2000 . Influence of the co-encapsulation of different non-ionic surfactants on the properties of PLGA insulin-loaded microspheres . J. Controlled Release. , 69 : 283 – 295 .
- Silva , CM , Ribeiro , AJ , Ferreira , D and Veiga , F . 2006 . Insulin encapsulation in reinforced alginate microspheres prepared by internal gelation . Eur. J. Pharm. Sci. , 29 : 148 – 159 .
- Singh , M , Li , XM , Wang , H , McGee , JP , Zamb , T , Koff , W , Wang , CY and O’Hagan , DT . 1997 . Immunogenicity and protection in small-animal models with controlled-release tetanus toxoid microparticles as a single-dose vaccine . Infect. Immun. , 65 : 1716 – 1721 .
- Tsifansky , MD , Yeo , Y , Evgenov , OV , Bellas , E , Benjamin , J and Kohane , DS . 2008 . Microparticles for inhalational delivery of antipseudomonal antibiotics . AAPS J. , 10 : 254 – 260 .
- Prior , S , Gamazo , C , Irache , JM , Merkle , HP and Gander , B . 2000 . Gentamicin encapsulation in PLA/PLGA microspheres in view of treating Brucella infections . Int. J. Pharm. , 196 : 115 – 125 .
- Yang , CY , Tsay , SY and Tsiang , RCC . 2001 . Encapsulating aspirin into a surfactant-free ethyl cellulose microsphere using non-toxic solvents by emulsion solvent-evaporation technique . J. Microencapsulation. , 18 : 223 – 236 .
- Qiu , X , Leporatti , S , Donath , E and Möhwald , H . 2001 . Studies on the drug release properties of polysaccharide multilayers encapsulated ibuprofen microparticles . Langmuir. , 17 : 5375 – 5380 .
- Coppi , G , Iannuccelli , V , Leo , E , Bernabei , MT and Cameroni , R . 2002 . Protein immobilization in crosslinked alginate microparticles . J. Microencapsulation. , 19 : 37 – 44 .
- Al-Omran , M , Al-Suwayeh , SA , El-Helw , AM and Saleh , SI . 2002 . Taste masking of diclofenac sodium using microencapsulation . J. Microencapsulation. , 19 : 45 – 52 .
- Augustin , MA and Sanguansri , L . 2008 . “ Encapsulation of bioactives ” . In Food materials science:principles and practice , Edited by: Aguilera , JM and Lillford , PJ . 577 – 601 . New York , NY : Springer .
- Tan , L , Chan , L and Heng , P . 2005 . Effect of oil loading on microspheres produced by spray drying . J. Microencapsulation. , 22 : 253 – 259 .
- Lupi F, Gabriele D, de Cindio B. Effect of shear rate on crystallisation phenomena in olive oil-based organogels. Food Bioprocess Technol. 2012;5:2880–2888.
- Pires , JL , Cascardo , JCM , Lambert , SV and Figueira , A . 1998 . Increasing cocoa butter yield through genetic improvement of Theobroma cacao L: seed fat content variability, inheritance, and association with seed yield . Euphytica. , 103 : 115 – 121 .
- Steinberg , FM , Bearden , MM and Keen , CL . 2003 . Cocoa and chocolate flavonoids: implications for cardiovascular health . J. Am. Diet. Assoc. , 103 : 215 – 223 .
- Liendo , R , Padilla , FC and Quintana , A . 1997 . Characterization of cocoa butter extracted from Criollo cultivars of Theobroma cacao L . Food Res. Int. , 30 : 727 – 731 .
- Baliga , B and Shitole , A . 1981 . Cocoa butter substitutes from mango fat . J. Am. Oil Chem. Soc. , 58 : 110 – 114 .
- Narasimha Char , B and Azeemoddin , G . 1989 . Edible fat from mango stones . Acta Hort. (ISHS). , 231 : 744 – 748 .
- Turcotte , AM and Scott , P . 2011 . Ochratoxin A in cocoa and chocolate sampled in Canada . Food Addit. Contam. , 28 : 762 – 766 .
- Ng , B . 2011 . Fiber content and storage stability of antioxidant activity of nutraceutical mango products and gluten-free cookies . aTDF , 480 : 43 – 48 .
- Chan , LW , Lee , HY and Heng , PWS . 2002 . Production of alginate microspheres by internal gelation using an emulsification method . Int. J. Pharm. , 242 : 259 – 262 .
- Ranathunge , K and Schreiber , L . 2011 . Water and solute permeabilities of Arabidopsis roots in relation to the amount and composition of aliphatic suberin . J. Exp. Bot. , 62 : 1961 – 1974 .
- Zhu , H , Srivastava , R , Brown , JQ and McShane , MJ . 2005 . Combined physical and chemical immobilization of glucose oxidase in alginate microspheres improves stability of encapsulation and activity . Bioconjug. Chem. , 16 : 1451 – 1458 .
- Nsereko , S and Amiji , M . 2002 . Localized delivery of paclitaxel in solid tumors from biodegradable chitin microparticle formulations . Biomaterials. , 23 : 2723 – 2731 .
- Shelma , R and Sharma , CP . 2011 . Development of lauroyl sulfated chitosan for enhancing hemocompatibility of chitosan . Colloids Surf., B. , 84 : 561 – 570 .
- Riyajan , SA and Sakdapipanich , JT . 2009 . Development of a controlled release neem capsule with a sodium alginate matrix, crosslinked by glutaraldehyde and coated with natural rubber . Polym. Bull. , 63 : 609 – 622 .
- Roy , S , Pal , K , Anis , A , Pramanik , K and Prabhakar , B . 2009 . Polymers in mucoadhesive drug-delivery systems: a brief note . Des. Monomers Polym. , 12 : 483 – 495 .
- Zhao , Q and Samulski , ET . 2006 . A comparative study of poly(methyl methacrylate) and polystyrene/clay nanocomposites prepared in supercritical carbon dioxide . Polymer. , 47 : 663 – 671 .
- Volkhard Jenning , SHG . 2001 . Encapsulation of retinoids in solid lipid nanoparticles (SLN) . J. Microencapsulation. , 18 : 149 – 158 .
- Abdallah , DJ , Sirchio , SA and Weiss , RG . 2000 . Hexatriacontane organogels. The first determination of the conformation and molecular packing of a low-molecular-mass organogelator in its gelled state . Langmuir. , 16 : 7558 – 7561 .
- Wright , A and Marangoni , A . 2006 . Formation, structure, and rheological properties of ricinelaidic acid-vegetable oil organogels . J. Am. Oil Chem. Soc. , 83 : 497 – 503 .
- Badve , SS , Sher , P , Korde , A and Pawar , AP . 2007 . Development of hollow/porous calcium pectinate beads for floating-pulsatile drug delivery . Eur. J. Pharm. Biopharm. , 65 : 85 – 93 .
- Martins , S , Sarmento , B , Souto , EB and Ferreira , DC . 2007 . Insulin-loaded alginate microspheres for oral delivery-Effect of polysaccharide reinforcement on physicochemical properties and release profile . Carbohydr. Polym. , 69 : 725 – 731 .
- Lin , WC , Yu , DG and Yang , MC . 2005 . PH-sensitive polyelectrolyte complex gel microspheres composed of chitosan/sodium tripolyphosphate/dextran sulfate: swelling kinetics and drug delivery properties . Colloids Surf., B. , 44 : 143 – 151 .
- Sawant , PD , Luu , D , Ye , R and Buchta , R . 2010 . Drug release from hydroethanolic gels: effect of drug’s lipophilicity (log P), polymer–drug interactions and solvent lipophilicity . Int. J. Pharm. , 396 : 45 – 52 .
- Pal , K and Pal , S . 2006 . Development of Porous Hydroxyapatite Scaffolds . Mater. Manuf. Processes. , 21 : 325 – 328 .