Abstract
Siloxane-containing polyazomethines with linear (PAZ) and cross-linked (CPAZ) architectures and their iron complexes (FePAZ and CFePAZ) have been prepared. The structures of the resulted compounds were investigated by spectral methods (FT-IR, 1H NMR, UV–vis) and electrospray ionization mass spectrometry (ESI-MS). X-ray fluorescence spectrometry was used to determine the Si/Fe ratio and for quantitative analysis of silicon. Paramagnetic susceptibility (χ) and magnetic moment (μ eff) of the soluble iron complex were estimated based on 1H NMR measurements. The iron spin state was determined by Mössbauer spectroscopy, while the electrochemical behavior was studied by cyclic voltammetry. The catalytic activity in hydrogen peroxide decomposition reaction, in alkaline solution was tested. The compound FePAZ proved to increase the dielectric constant of a silicone matrix in which it was incorporated even in very low percent, acting also as an effective plasticizer.
1. Introduction
Polyazomethines or poly(Schiff base)s, polymers containing C=N bonds which are isoelectronic with the CH=CH group, are considered an alternative to conjugated polymers Citation[1], that require mild reaction conditions. Their synthetic methodology consists in condensation polymerization of diamines with dialdehydes (or diketones), without noble or sophisticated catalysts, leading to high yields and purity products. Polyazomethines are thermally stable and environmentally resistant polymers Citation[2], some of them having thermotropic behavior and potential applications for high-performance fibers or in opto-electronics Citation[3–6]. Low solubility and high melting or softening temperatures are the main drawbacks of aromatic poly(azomethine)s. Seeking for improved processability and high molecular weight polymers, different modifications of the synthetic methods have been reported Citation[7]. In order to reduce the transition temperatures and to improve the solubility, variations in the organic structure (like incorporating flexible aliphatic units into the main chain, introducing pendent alkyl groups along the backbone, and/or copolymerizing of various dialdehydes and diamines) were applied Citation[8–10]. Another way to improve the properties is to introduce siloxane moieties within the rigid conjugated aromatic azomethine structures. In our group, a rather large variety of poly(siloxane–azomethine)s having ether or ester linkages have been synthesized Citation[11–18], from low molecular mass compounds and dimesogenic oligomers, to segmented copolymers, and their thermotropic behavior and complexing ability have been investigated. In fact, on the ability to coordinate metal ions is based one of the main applications of the polyazomethines leading to systems with enhanced thermal stability and often improved chemical resistance Citation[19].
The most studied small molecular Schiff base metal complexes are N,N′-nis(salicylidene)-ethylenediamine, (M(salen)) Citation[20]. Many interesting properties have been reported, like: electroluminescence, magnetic, nonlinear optical properties, or oxygen transport. Especially the catalytic activity of Schiff base transition metal complexes of salen type has been investigated and proved to be very good Citation[21]. Antibacterial, antifungal, or cytotoxic activities are also widely reported Citation[22–24]. Polymeric Schiff base metal complexes may be obtained by complexation after the synthesis of the macromolecular ligand, by reaction of functional metal complexes with appropriate monomers, or by electrochemical procedures Citation[20]. The polymers obtained by the first method are generally insoluble. In other cases, the complexation was successfully used for solubilization of aromatic polyimines in organic solvents via their soluble coordination complexes which facilitated their solution characterization by NMR spectroscopy and processing to films and coatings Citation[1].
Here, we report on synthesis and characterization of an oligo(siloxane–azomethine) iron(III) complex and a homologous cross-linked coordination polymer. The linear ligand was prepared from siloxane-containing monomers – 1,3-bis(aminopropyl)tetramethyldisiloxane and 1,3-bis(formyl-p-phenoxymethyl)tetramethyldisiloxane – thus having a simple and highly flexible structure. This macromolecular ligand was then reacted with FeCl3·6H2O. The resulted iron complex, a soluble viscous material, has been investigated by spectral methods (FT-IR, 1H NMR, UV–Vis), electrospray ionization mass spectrometry (ESI-MS), cyclic voltammetry, differential scanning calorimetry (DSC), and Mossbauer spectroscopy. A small percent (2.3%) of this complex has been introduced into a PDMS matrix, in order to obtain a material with increased dielectric constant and good mechanical properties. The cross-linked poly(siloxane–azomethine) has been obtained from a siloxane copolymer with aminopropyl pendant groups and the siloxane dialdehyde. The corresponding iron complex was prepared similarly. Preliminary catalytic tests have been performed on the soluble and cross-linked complexes, with promising results.
2 Experimental
2.1 Materials
The starting materials were purchased from Sigma-Aldrich and used without purification: 1,3-bis(3-aminopropyl)1,1,3,3-tetramethyldisiloxane (AP), 3-aminopropyl(diethoxy)methylsilane (APMDES), dimethyldiethoxysilane (DMDES), and Iron(III)chloride hexahydrate (FeCl3 6H2O). Tetraethyl-orthosilicate (TEOS) was purchased from Fluka and dibutyltin dilaurate from Merck.
1,3-bis(formyl-p-phenoxymethyl)tetramethyldisiloxane siloxane dialdehide (SA) was prepared according to a previously reported procedure Citation[17].
Poly(dimethylsiloxane)-α,ω-diol PDMS (M n = 48 000) was synthesized according to a previously described procedure Citation[25], i.e. cationic ring-opening polymerization of octamethylcyclotetrasiloxane in the presence of a cation exchanger as catalyst.
2.2 Physico-chemical characterization
The presence and ratio of Fe and Si were evidenced using an Energy-Dispersive X-ray Fluorescence (EDXRF) system EX-2600 X-Calibur SDD. Apart from the qualitative assessment, we made a quantitative evaluation of the Si content. The method for sample preparation and data recording was as follows: In all cases, pieces of filter paper, of 1.5 × 1.5 cm were cut from the same bulk, then 10 mg of silicon-containing materials were dissolved in minimum volume of suitable solvents (preferably chloroform) and the filter papers were carefully impregnated with the solutions. After drying, each piece of paper was subjected to XRF analysis. The measurements were done in the same conditions for all the samples, i.e. 50 μA, 15 kV, 180 s, in vacuum. The Kα 1 peak for Si was identified and its intensity (in Counts) was represented as a function of Si content in order to obtain a calibration curve, using commercially available materials: PDMS, 1,3-bis(carboxypropyl)tetramethyldisiloxane, 1,3-bis(glycidoxypropyl)tetramethyldisiloxane, and diphenylsilanediol. The samples were measured at least twice on each side and an average value was calculated.
The infrared spectra were registered on a Bruker Vertex 70 FT-IR instrument, in transmission mode, in the 300–4000 cm−1 range (resolution 2 cm−1, 32 scans), at ambient temperature. Far Fourier transform infrared spectra were recorded using with a CsI beamsplitter that covers a spectral range from 600 to 180 cm−1, in transmission mode at room temperature with a resolution of 2 cm−1 and accumulation of 64 scans.
The electronic absorption spectra were measured with an Analytic Jena SPECORD 200 spectrophotometer in 10 mm optical path quartz cells fitted with polytetrafluoroethylene stoppers.
The mass spectrometry data were obtained using an Agilent 6520 Series Accurate-Mass Quadrupole Time-of-Flight (Q-TOF) LC/MS. The solutions were introduced into the electrospray ion source (ESI) via a syringe pump at a flow-rate of 0.01 mL/min. After optimization of the Q/TOF MS parameters, they were set as follows: electrospray ionization (positive ion mode), drying gas (N2) flow rate 10.0 L/min; drying gas temperature 325 °C; nebulizer pressure 35 psig, capillary voltage 4200 V; fragmentation voltage 400 V; the full-scan mass spectra of the investigated compounds were acquired in the m/z range of 100–3000. The mass scale was calibrated using the standard calibration procedure and compounds provided by the manufacturer. The data was collected and processed using MassHunter Workstation software.
Gel permeation chromatography (GPC) measurements were made in DMF on a PL-EMD 950 Chromatograph – Evaporative Mass Detector. The calibration was performed with polystyrene standards.
The 1H NMR spectra for calculation of the paramagnetic susceptibility (χ) and magnetic moment (μ eff) were acquired on a 400 MHz Brüker spectrometer, in DMSO-D6 with tert-buthanol as external reference Citation[26]. The calculations were done using Equations (1)–(4).
where Δ(v) is the difference between the resonance frequencies in the presence and in the absence of the paramagnetic material (Hz); m is the sample mass (mg); = 0.63 × 10−6; M is the molecular weight; T is the temperature (298 K); and n is the the number of the paramagnetic ions (in our case, 2).
DSC investigations were done on a Pyris Diamond DSC (Perkin Elmer USA) instrument. The samples were cooled from room temperature to −150 °C, then heated with 10 °C/min, in order to detect the glass transition temperature in the second heating run.
The Mössbauer spectrum was acquired using a conventional spectrometer in the constant-acceleration mode (MS4, Edina, USA) equipped with a 57Co source (3.7 GBq) in a rhodium matrix. Isomer shifts are given relative to α-Fe at room temperature. The samples were measured at 300 K and the spectra were fitted using the NORMOS Mössbauer Fitting Program.
The electrochemical measurements were performed with an Autolab System PGSTAT-302 N potentiostat/galvanostat from Echo Chemie (Utrecht, Netherlands) connected to a PC and controlled by GPES software. The electrochemical cell consists of working electrode: carbon (4 mm diameter), counter electrode: carbon and reference electrode: Ag/AgCl with a planar configuration of screen printed electrode (SPE, Ref. DRP-110), fabricated by DropSens, Asturias, Spain. All potentials are referred to Ag/AgCl. Tetrabutylammonium fluoride trihydrate (TBAF – Aldrich) 0.1 M was used as the supporting electrolyte for electrochemical measurements in ethanol solution.
For mechanical testing of the PDMS-based materials, stress–strain measurements were performed on dumbbell-shaped cut samples on a TIRA test 2161 apparatus, Maschinenbau GmbH Ravenstein, Germany. Measurements were run at an extension rate of 50 mm/min, at room temperature. Novocontrol setup (Broadband dielectric spectrometer Concept 40, GmbH Germany), integrating an ALPHA frequency response analyzer and a Quatro temperature control system, was used to investigate the dielectric properties of the polymer films over a broad frequencies window, 100–106 Hz.
2.3 Syntheses
2.3.1 Synthesis of polyazomethine PAZ
0.31 g SA (0.77 mmol) and 0.21 mL AP (0.19 g, 0.77 mmol) were dissolved in 3 mL of methanol/methylene chloride (1/1 vol) and heated at 60 oC for 4 h, then the solvents were removed in vacuo.
1H NMR (CDCl3 δ, ppm): 8.22 (m, 2H, CH=N), 7.72–7.51 (m, 4H aromatic, ortho to –CH=), 7.02–6.73 (m, 4H aromatic, ortho to –O–), 3.85–3.60 (m, 4H CH2–O–; 4H CH2–N=), 1.80–1.72 (m, 4H C–CH2–C), 0.64–0.50 (m, 4H C–CH2–Si), 0.35–0.05 (m, 24 H CH3–Si).
FT-IR (KBr pellet, cm−1): 3414m, 2957s, 2925s, 2898s, 1645s, 1605vs, 1578s, 1510s, 1417 m, 1286s, 1254vs, 1165s, 1051vs, 835vs, 798vs, 704 m, 600 m, 530 m.
2.3.2 Synthesis of FePAZ
0.20 g (0.32 mmol) PAZ and 0.35 g (1.3 mmol) FeCl3· 6H2O were separately dissolved in methanol/dichloromethane (1/1 vol). To the warm PAZ solution (50 °C), the metal salt solution was added dropwise, then the reaction mixture was stirred for 2 h at 60 °C. The solvents were removed and the product was washed with water several times and dried.
FT-IR (KBr pellet, cm−1): 3391s, 3221s, 2959s, 2901s,1668s, 1597vs, 1508s, 1425 m, 2300s, 1258vs, 1159s, 1059vs, 925 m, 839vs, 802s, 704 m, 646 m, 610 m, 515 m.
2.3.3 Synthesis of poly[dimethyl-methyl(3-aminopropyl)siloxane] (SAP)
16 mL (13.80 g, 93.3 mmol) DMDES was mixed with 6.7 mL (6.10 g, 32.0 mmol) 3-APMDES, then 2.2 mL 1 N HCl aqueous solution was added dropwise during 15 min under vigorous magnetic stirring. The mixture was further stirred up to 24 h at room temperature after which it was devolatilized at room temperature/10 mm Hg. The remaining oil was repeatedly washed with water until neutral pH of the waste waters was achieved.
1H NMR(CDCl3, δ, ppm): 2.651–2.665 (–CH2–NH2), 1.475–1.487 (–CH2–CH2–CH2–); 0.499–0.568 (–Si–CH2–), 0.065–0.111 (CH3–Si–); intensity ratio: 1:1:1:10, indicating about 25.5% mol methyl(3-aminopropyl)siloxane groups content.
FT-IR (KBr pellet, cm−1): 3363s, 3289s, 2962s, 2927s, 1596s, 1444s, 1412s, 1259s, 1074–1016vs, 922s, 847s, 811s, 776s, 702s, 692s, 554 m, 531 m, 466w, 417w.
2.3.4 Synthesis of cross-linked polyazomethine (CPAZ)
0.30 g SAP (3.53 mmol) and 0.32 g SA (0.8 mmol), each dissolved in chloroform (total volume 3 ml), were mixed together at 40 °C for 5 h. The solvent was left to evaporate overnight from the viscous solution. The crude product was extracted with ethanol to remove the excess dialdehyde, then dried.
FT-IR (KBr pellet, cm−1): 3420w, 2964m, 2930w, 2833w, 1647m, 1607m, 1580w, 1510m, 1417w, 1385s, 1288m, 1261s, 1165m, 1097–1012vs, 800vs, 698w, 604w, 530w, 399w.
2.3.5 Synthesis of complex CFePAZ
The cross-linked polyazomethine (CPAZ) (0.1 g, 0.096 mmol) was swolen in chloroform, then soaked in a methanol/dichloromethane solution of FeCl3·6H2O (0.1 g, 0.37 mmol) for 4 h. The color changed from yellow to dark brown. The mixture was warmed at 40 °C and left to evaporate. The crude product was washed several times with water, then dried.
FT-IR (KBr pellet, cm−1): 3435 m, 2963 m, 2925w, 2855vw, 1672 m, 1601s, 1510 m, 1416 m, 1385 m, 1261vs, 1159s, 1095–1022vs, 802vs, 690 m, 609w, 513w, 399w.
2.3.6 Preparation of silicone-based blend
To a solution consisting of 1 g PDMS in 5 mL chloroform, 0.5 mL TEOS were added. The mixture was stirred for 10 min then 2 drops of dibutyltin dilaurate catalyst were added, as well as a solution containing 0.035 g of metal complex FePAZ in 2 ml chloroform, and stirred for 10 min. The resulted mixture was poured into a 10 cm diameter Teflon Petri dish. The dish was left at ambient conditions to slowly evaporate the solvent and cross-link.
2.4 Tests of catalytic activity
Catalytic tests were done with FePAZ and CFePAZ for hydrogen peroxide decomposition, in alkaline solution, using the gasometric technique Citation[27]. In this reaction, the H2O2 is present as perhydroxyl ion and oxygen evolves from the vessel, according to the equation:
In principle, the O2 formed displaces water from a Bunte gas burette and its volume is measured vs. time, at atmospheric pressure and 300 K. After performing a blank experiment, the soluble complex FePAZ was used as a solution in 1 ml methanol (homogeneous catalyst), while CFePAZ was used as such (heterogeneous catalyst). For all the experiments, a 4 N NaOH solution and the catalyst were mixed together for 15 min, then 15% H2O2 was added (10/1 vol. ratio) and the oxygen evolution was monitored until no modification was noticed for at least 5 min.
An additional test was done using cyclohexanol as a substrate in the oxidation reaction. The experimental procedure was as follows: 0.5 mL of cyclohexanol and 1.5 mL of methanol were mixed with 10 mg of FePAZ, then 1 mL of 30% H2O2 was added and the reaction carried on at 60 °C. The methanol and water were removed in vacuum and the resulted product was analyzed by FT-IR and 1H NMR.
3 Results and discussion
The SA has been previously reported and polyazomethines have been prepared thereof, using different organic diamines, in order to obtain liquid crystalline polymers Citation[17,28]. Here, it was combined with a siloxane diamine, thus obtaining a highly flexible and soluble polyazomethine (PAZ) (Scheme ).
In the FT-IR spectrum of PAZ, the aldehyde band from 1690 cm−1 in SA disappeared, as well as the primary amine group bands from 1581, 3293, and 3360 cm−1 in AP0. The new, azomethine band was observed at 1645 cm−1. The siloxane bands were visible at: 1254 cm−1 (δ sy Si–CH3), 835, and 798 cm−1 (γ Si–(CH3)2), 1051 cm−1 (Si–O–Si asymmetric stretch).
The 1H NMR spectrum also confirmed the polycondensation reaction. The aldehyde protons at 9.91 ppm in SA disapeared, while the N=CH protons were assigned at 8.22 ppm and all the expected protons were present, in the correct ratio.
For the synthesis of cross-linked polyazomethine, the polysiloxane functionalized with aminopropyl groups SAP was obtained in a preliminary step, by co-hydrolysis of the DMDES with APMDES in water – hydrochloric acid, according to procedures described in the literature Citation[29,30] and adapted by us Citation[18] (Scheme ). The structure of the resulted oily product was verified by 1H NMR spectroscopy. A content of about 25.5% mol methyl(3-aminopropyl)siloxane groups was found.
The condensation reaction between this copolymer and dialdehyde SA was followed by FT-IR, having the linear compound PAZ as a model. The presence of the CH=N band at 1647 cm−1 and the complete disappearance of the CH=O band from 1690 cm−1 confirmed the reaction (Figure ). The siloxane band exhibited the typical shape for a polymer, i.e. a strong double-band at 1012 and 1097 cm−1.
Figure 1 FT-IR spectra of the cross-linked polyazomethine and the corresponding iron(III) complex. Insert shows the Far-IR region for the complex FePAZ.
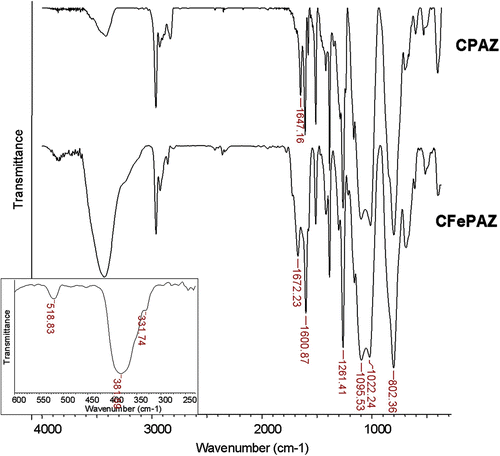
The synthesis of iron(III) complexes (Scheme ) was made by reacting FeCl3·6H2O with the pre-formed polyazomethines.
The complexation reactions were followed by FT-IR. In the spectrum of the iron complex FePAZ, the azomethine band shifted to 1668 cm−1. In the Far-IR region (Figure ), the absorption band at 519 cm−1 was assigned to the Fe–N bond Citation[31,32], while the bands at 382 and 331 cm−1 may be assigned to Fe–Cl stretching frequencies Citation[33,34]. The cross-linked complex CFePAZ showed very similar FT-IR spectrum, with the coordinated azomethine bond at 1672 cm−1 (Figure ).
The DSC scan for the complex FePAZ showed a glass transition temperature (T g) at −16 °C, slightly higher than the corresponding ligand, PAZ, which has the Tg value of −23 °C. A single T g value indicates a unitary polymer, with monophasic morphology.
Electrospray (ESI) ionization mass spectrometry was used to obtain information about the mass of the structural unit and to verify the polymeric nature of the soluble complex FePAZ. In the ESI-MS spectrum obtained in the negative mode, we observed many multiple charge peaks, which correspond to mass values in the oligomeric range. The assignment of the structure was made based on the fact that Fe has a coordination number of 6 and it is trivalent in our compound. The main species detected, having 1–4 repeating units (RU), are described in Table .
Table 1. ESI-MS data and assignment of the peaks (negative mode of ionization, methanol) for the complex FePAZ.
The GPC curve of FePAZ, registered in DMF gave the following values: M n = 2700, M w = 3040, polydispersity index 1.13. The measured molecular weight values are slightly lower than that of the corresponding PAZ ligand (M n = 3240, M w = 3600), probably due to the different hydrodynamic volume and conformation in solution. Although the values are not absolute, there is a good correlation with the MS data (species with mass in the range 1250–4200 detected), confirming that FePAZ is an oligomer.
Due to the oligomeric nature and to the structural complexity of FePAZ, in the calculations that follow we used the molecular weight of the structural unit (RU), as it was deducted from the mass spectrum, i.e. 1011.55.
The absorption spectrum of the iron complex, in ethanol (Figure ) was compared with the ligand. PAZ showed the bands characteristic for the π−π ∗ benzenoid transitions (λ max about 270 nm) and to π−π ∗ transitions of the azomethine chromophore (λ max about 288 nm). In the metal complex FePAZ, these absorption bands were slightly shifted to 274 nm and 282 nm, while two new bands at 330 nm and 374 nm (shoulder) appeared, due to the binding of the metal to the ligand. (For comparison, the starting FeCl3· 6H2O in ethanol shows two broad bands, centered at 250 and 360 nm, respectively.) The extinction coefficients (ϵ, L/mol cm) are presented in Table for the polyazomethine and its complex.
Figure 2 The UV–Vis spectrum of the iron complex, compared with the corresponding polyazomethine ligand PAZ.
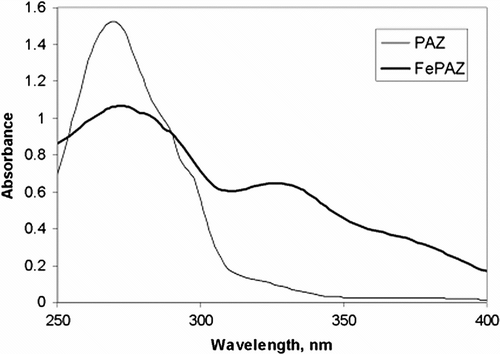
Table 2. UV data for the iron complex and the ligand (in ethanol).
Based on EDXRF spectrum, the ratio between Si and Fe was determined for FePAZ, having a value of 1.95. The quantitative analysis by this method is subjected to limitations and implies suitable calibration. On the other hand, it is a fast and non-destructive method, which can use very small amounts of the sample. In the case of Si and other ‘light’ elements, in order to get reliable results, the measurements are to be done in vacuum. Apart from finding suitable etalons, for liquid siloxane-containing compounds, or very small amounts of powder (not enough to make pellets), the quantitative analysis may be quite challenging. The method used frequently for the determination of Si content is based on desegregation with concentrated sulfuric acid of at least 0.1 g of sample Citation[35] and is a time consuming, polluting, and not always a very accurate method, which is not reliable for metal-containing compounds. Given these arguments, we tried to find a way for having very fast and satisfactory accurate results for the silicon content of our samples, by XRF method.
In order to perform quantitative analysis of Si%, we have constructed a calibration curve, using commercially available siloxane compounds and used it to estimate the Si content of PAZ and FePAZ. The values obtained were in good agreement with the theoretical ones: PAZ 18.53% (calcd: 18.26%); FePAZ 11.29% (calcd: 11.07%). Given the oligomeric nature of the compounds and the complex structure of FePAZ, we may conclude that the method is satisfactory. Since it can only be applied to soluble compounds, in the case of CFePAZ, we could only consider the Si/Fe ratio calculated from the XRF spectrum (Figure ). The found value of 4.6 is in rather good correlation with the theoretical structure. If we consider an average of 10 Si atoms per structural unit, this gives a Si/Fe theoretical ratio of 5.
The 1H NMR spectroscopy was used to calculate the paramagnetic susceptibility (χ) and magnetic moment (μ
eff) of FePAZ, as described in Citation[26] (see Section 2.2. for details). In the case of FePAZ, the number of the paramagnetic ions per structural unit is n = 2. The calculated values are: χm
= 29,203 × 10−6 (c.g.s.); μ
eff = 5.9 B.M., which is practically identical to the expected value for Fe3+ ions (i.e. ). Thus, we have an indirect confirmation of the purity and correct assignment of the molecular mass of our compound.
The Mössbauer spectrum of FePAZ was registered at room temperature (Figure ). The obtained parameters are as follows: the isomer shift δ = 0.367 mm/s, the quadrupole splitting ΔE Q = 0.649 mm/s, and the line width Γ (defined as the full-width at half-maximum peak height) = 0.493 mm/s. These data indicate the presence of iron(III) in a high spin state (S = 5/2).
Cyclic voltammetry technique was used to study the electrochemical behavior of the iron complex FePAZ in ethanol, with 0.1 M TBAF as supporting electrolyte.
The electrochemical properties of the iron complex were investigated in comparison to the polyazomethine precursor. Figure shows that the polyazomethine displayed one small oxidation peak around +60 mV corresponding to the electronic transfer process at the CH=N group level. For the complex, in the anodic scan, the appearance of two oxidation peaks nearly overlapped demonstrates that FePAZ undergoes two consecutive oxidation processes under potential control. The first oxidation peak (I a) which appeared around +20 mV can be attributed to the electronic transfer process involving the carbon–nitrogen double bond in the polymeric chain. So, in the complex, the peak I a corresponding to CH=N oxidation is shifted to smaller potential value, compared to PAZ. This difference may be explained by the delocalization of electrons in the coordination complex, which lowers the potential required for oxidation.
Figure 5 Comparison of the cyclic voltammetry data for the iron complex FePAZ (solid line) and the ligand PAZ (thin line) dissolved in ethanol and TBAF as electrolyte (dotted line). Scan rate: 50 mV/s.
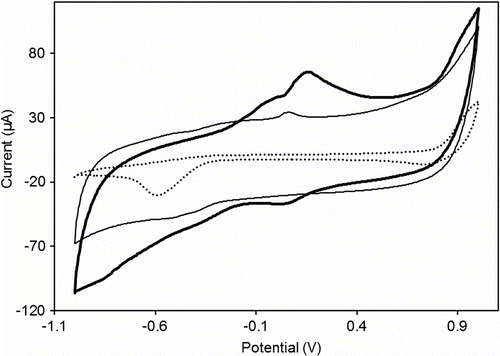
The second oxidation peak (II a) appeared around + 100 mV, accompanied by a corresponding reduction peak at +30 mV and can be assigned to the oxido-reduction of the coordinated iron sites along the polymer chain. The peak separation of this couple (ΔEII = EII a–EII c) is ≈ + 70 mV, which is characteristic for a slow (quasi) reversible one electron transfer process.
Figure shows a couple of voltammograms obtained for increasing concentrations of FePAZ dissolved in ethanol and 0.1 TBAF. The potential was swept in a large window potential (−1 V to +1.4 V) with a scan rate of 50 mV/s.
Figure 6 Cyclic voltammetry in TBAF as supporting electrolyte without (dotted line) and with increasing concentrations of FePAZ in ethanol (solid lines): (1) 0.33 mM; (2) 0.56 mM; (3) 0.74 mM; and (4) 0.88 mM. Scan rate: 50 mV/s. Potential scanned: −1 to + 1.4 V.
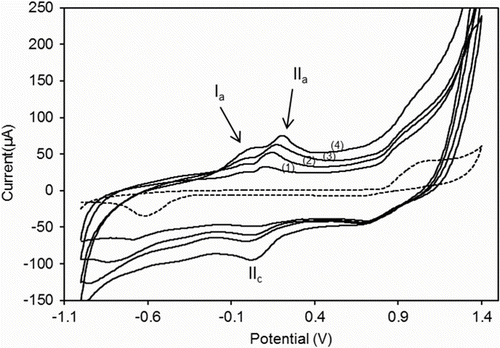
The detection of single reversible oxidation wave for iron moieties indicates that in these polymers the iron centers are basically noninteracting. It was shown that iron-based polymers in which the metallic units are spaced out at a significant distance show only very weak or nonexistent interactions between the iron centers Citation[36].
These results indicate that the oxidation of the iron complex occurs via an irreversible electron transfer corresponding to C=N bond followed by a quasi-reversible oxido-reduction of iron sites. As suggested by the cyclic voltametry data, the electrocatalytic response of the siloxane-containing iron complex is rather weak.
For certain applications of silicones, like actuation, increased dielectric constant (ϵ) is a requirement. One way to achieve this goal is to use high ϵ materials as fillers. In order to get some information about the dielectric properties of FePAZ and its effect on a polydimethylsiloxane (PDMS) elastomer matrix, we prepared a blend, by mixing PDMS, TEOS (in 2/1 wt. ratio), and 2.3% FePAZ, followed by cross-linking of the film. The dielectric constant of the obtained material increased by 30% (from 2.3 in neat PDMS to a value of 3), while a two order increase in conductivity was registered, due to the presence of the metal complex.
The mechanical properties of this film were also evaluated. A plasticizing effect was noticed, since the Young modulus decreased by 36% (from 99 kPa for the PDMS material used as the matrix to 73 kPa). Additionally, 47% increase in tensile strength and practically unchanged elongation at break (around 375%) were registered.
Preliminary catalytic tests were done for FePAZ and CFePAZ in hydrogen peroxide decomposition reaction, in alkaline solution. The turnover number (TON) was calculated as moles of H2O2 per mole of catalyst and turnover frequency TOF, as TON/time. The obtained values for the two complexes tested are listed in Table . The homogeneous catalyst (FePAZ in methanol) gave a fivefold higher TON value than the equivalent heterogeneous one. Instead, the cross-linked complex was about three times faster than FePAZ in the same conditions. For comparison, the PDMS–FePAZ blend showed no catalytic effect probably due to the pronounced hydrophobic character of the matrix, which precluded the reaction in water.
Table 3. The catalytic parameters of the polymeric iron complexes for the hydrogen peroxide decomposition in basic conditions.
We also tested FePAZ in the oxidation of cyclohexanol to cyclohexanone, at 60 °C. The conversion was 36% after 4 h (by 1H NMR). This is a promising result, compared with the reported data for this oxidation agent Citation[37,38]. Further investigations are in progress.
Conclusion
A polyazomethine from siloxane-containing diamine and dialdehyde, the corresponding iron(III) complex, as well as cross-linked homologous compounds were synthesized and characterized. We used mass spectrometry to identify oligomeric species in the soluble metal complex and a specially designed method for quantitative measurement of Si content by XRF spectroscopy. Based on 1H NMR measurements, the paramagnetic susceptibility and magnetic moment of the soluble iron complex were estimated as χ m = 29,203 × 10−6 c.g.s. and μ eff = 5.9 B.M. (this being practically identical to the expected value for Fe3+ ions). The presence of iron(III) in a high spin state (S = 5/2) was also confirmed by Mössbauer spectroscopy. The electrochemical behavior was studied by cyclic voltammetry. The effect of about 2% of the iron complex on the dielectric and mechanical properties of a silicone matrix were rather significant. The catalytic activity in hydrogen peroxide decomposition reaction, in alkaline solution was tested for both soluble and cross-linked polymeric metal complexes. The oxidation of cyclohexanol was tested for a possible application.
Acknowledgments
This research was financially supported by the European Regional Development Fund (SOP IEC-A2-O2.1.2-2009-2, ID 570, CODE SMIS-CSNR: 12473, Contract 129/2010-POLISILMET).
The authors thank Professor Constantin Turta for helpful discussion.
One of the authors (Adina Arvinte) acknowledges the financial support of European Social Fund – “Cristofor I. Simionescu” Postdoctoral Fellowship Programme (ID POSDRU/89/1.5/S/55216), Sectoral Operational Programme Human Resources Development 2007–2013.
References
- Yang , CJ and Jenekhe , SA . 1995 . Conjugated aromatic polyimines. 2. Synthesis, structure, and properties of new aromatic polyazomethines . Macromolecules. , 28 : 1180 – 1196 .
- Kimura , K , Zhuang , JH , Shirabe , K and Yamashita , Y . 2003 . Preparation of needle-like poly(azomethine) crystals by means of reaction-induced crystallization of oligomers . Polymer. , 44 : 4761 – 4764 .
- Matsumoto , T , Matsuoka , T , Suzuki , Y , Miyazawa , K , Kurosaki , T and Mizukami , T . 2003 . Synthesis of fully conjugated aromatic polyazomethine from AB-type monomer using soluble precursor method . Macromol. Symp. , 199 : 83 – 96 .
- Morgan , PW , Kwolek , SL and Pletcher , TC . 1987 . Aromatic azomethine polymers and fibers . Macromolecules. , 20 : 729 – 739 .
- Wojtkowski , PW . 1987 . Aromatic-aliphatic azomethine ether polymers and fibers . Macromolecules. , 20 : 740 – 748 .
- Sek , D , Iwan , A , Kaczmarczyk , B , Jarzabek , B , Kasperczyk , J and Bednarski , H . 2007 . New conjugated azomethines containing triphenylamine core – characterization and properties . High Perform. Polym. , 19 : 401 – 426 .
- Iwan , A and Sek , D . 2008 . Processible polyazomethines and polyketanils: from aerospace to light-emitting diodes and other advanced applications . Prog. Polym. Sci. , 33 : 289 – 345 .
- Kim , H , Park , SB , Jung , JC and Zin , WC . 1996 . Structure and phase behavior of polyazomethines having flexible (n-alkoxy)methyl side chains . Polymer. , 37 : 2845 – 2852 .
- Adell , JM , Alonso , MP , Barbera , J , Oriol , L , Pinol , M and Serrano , JL . 2003 . Structural modifications and fibre processing of hydroxy-functionalized mesogenic polyazomethines . Polymer. , 44 : 7829 – 7841 .
- Choi , MK , Kim , HL and Suh , DH . 2006 . Changes of fluorescence color in novel poly(azomethine) by the acidity variation . J. Appl. Polym. Sci. , 101 : 1228 – 1233 .
- Racles , C and Cozan , V . 2002 . Siloxane-containing thermotropic liquid crystals based on new azomethine dimesogens . High Perform. Polym. , 14 : 169 – 181 .
- Racles , C , Cozan , V , Cazacu , M , Foldes , E and Sajo , I . 2002 . Poly(azomethine–ester–siloxane)s. Synthesis and thermal behaviour . High Perform. Polym. , 14 : 397 – 413 .
- Racles , C , Cazacu , M , Ioanid , A and Vlad , A . 2008 . Micellization of a siloxane-based segmented copolymer in organic solvents and its use as a tool for metal complex nanoparticles . Macromol. Rapid Commun. , 29 : 1527 – 1531 .
- Racles , C , Cazacu , M , Vasiliu , M and Cozan , V . 2005 . Structure-LC properties relationship in siloxane–azomethine compounds . Polym. Plast. Technol. Eng. , 44 : 1049 – 1058 .
- Cazacu , M , Marcu , M , Vlad , A , Toth , A and Racles , C . 2003 . Chelate polymers. III. New polyazomethines of 5,5′-methylene-bis-salicylaldehyde with siloxane diamines and their divalent metal complexes . J. Polym. Sci, A: Polym. Chem. , 41 : 3169 – 3179 .
- Bronnikov , S , Racles , C , Nasonov , A and Cazacu , M . 2006 . Kinetics of the nematic ordered phase growth during a temperature quench of an isotropic siloxane–azomethine polymer . Liq. Cryst. , 33 : 1015 – 1019 .
- Racles , C , Cozan , V and Sajo , I . 2007 . Influence of chemical structure on processing and thermotropic properties of poly(siloxane–azomethine)s . High Perform. Polym. , 19 : 541 – 552 .
- Vlad , A , Cazacu , M , Turta , C , Tigoianu , RI , Airinei , A and Arvinte , A . 2012 . Telechelic or side-functionalized diorganosiloxanes with ferrocenylimine groups . Synth. Met. , 161 : 2659 – 2668 .
- Saraii , M and Entezami , AA . 2003 . Synthesis and characterization of poly Schiff bases derived from 5a,10b-dihydrobenzofuro [2,3-b] benzofuran-2,9-dicarbaldehyde with various diamines . Iran Polym. J. , 12 : 43 – 50 .
- Leung , ACW and MacLachlan , MJ . 2007 . Schiff base complexes in macromolecules . J. Inorg. Organomet. Polym. Mater. , 17 : 57 – 89 .
- Gupta , KC and Sutar , AK . 2008 . Catalytic activities of Schiff base transition metal complexes . Coord. Chem. Rev. , 252 : 1420 – 1450 .
- Nishat N, Parveen S, Dhyani S, Asma. Antimicrobial.polyesters containing Schiff base metal complexes. J. Coord. Chem. 2009;62:1091–1099. Available from: http://www.tandfonline.com/doi/pdf/10.1080/00958970802416020
- Abdulghani AJ, Abbas NM. Synthesis characterization and biological activity study of new Schiff and Mannich bases and some metal complexes derived from isatin and dithiooxamide. Bioinorg. Chem. Appl. 2011. doi:10.1155/2011/706262.
- Sabik AE, Karabork M, Ceyhan G, Tumer M, Digrak M. Polydentate Schiff base ligands and their La(III) complexes: synthesis, characterization, antibacterial, thermal, and electrochemical properties. Int. J. Inorg. Chem. 2012. doi:10.1155/2012/791219.
- Alexandru , M , Cristea , M , Cazacu , M , Ioanid , A and Simionescu , BC . 2009 . Composite materials based on polydimethylsiloxane and in situ generated silica by using the sol–gel technique . Polym. Compos. , 30 : 751 – 759 .
- Evans DF. The determination of the paramagnetic susceptibility of substances in solution by nuclear magnetic resonance. J. Chem. Soc. 1959; 2003–2005.
- Goldstein , JR and Tseung , ACC . 1974 . The kinetics of hydrogen peroxide decomposition catalyzed by cobalt–iron oxides . J. Catal. , 32 : 452 – 465 .
- Bronnikov , S , Nasonov , A , Racles , C and Cozan , V . 2008 . Kinetics of the isotropic-ordered phase transition in binary mixtures of mesogenic monomers and polymers . Soft Mater. , 6 : 119 – 128 .
- Bennevault-Celton , V , Maciejak , O , Desmazières , B and Cheradame , H . 2010 . Condensation of alkoxysilane in alcoholic media: I. Oligomerization of dimethyldiethoxysilane . Polym. Int. , 59 : 43 – 54 .
- Noll , W . 1968 . Chemistry and technology of silicones , New York , NY : Academic Press .
- Annigeri , SM , Naik , AD , Gangadharmath , UB , Revankar , VK and Mahale , VB . 2002 . Symmetric binuclear complexes with an ‘end-off’ compartmental Schiff base ligand . Trans. Met. Chem. , 27 : 316 – 320 .
- Tuncel , M , Ozbulbul , A and Serın , S . 2008 . Synthesis and characterization of thermally stable Schiff base polymers and their copper(II), cobalt(II) and nickel(II) complexes . React. Funct. Polym. , 68 : 292 – 306 .
- Nakamoto , K. 1986 . Infrared and Raman spectra of inorganic and coordination compounds. Applications in coordination, organometallic, and bioinorganic chemistry , 4th ed. , New York , NY : Wiley .
- Chervonova , UV , Gruzdeva , MS , Kolkera , AM , Manina , NG and Domracheva , NE . 2010 . Iron(III) complexes on the basis of azomethine derived from 4,4′-dodecyloxybenzoyloxybenzoyl-4-oxy-2-hydroxybenzaldehyde . Russ. J. Gen. Chem. , 80 : 1954 – 1962 .
- Smith AL, editor. The analytical chemistry of silicones. New York, NY: Wiley Interscience; 1991.
- Flanagan , JB , Margel , S , Bard , AJ and Anson , FC . 1978 . Electron transfer to and from molecules containing multiple, noninteracting redox centers. Electrochemical oxidation of poly(vinylferrocene) . J. Am. Chem. Soc. , 100 : 4248 – 4253 .
- Demetgül , C , Delikanli , A , Saribiyik , OY , Karakaplan , K and Serin , S . 2012 . Schiff base polymers obtained by oxidative polycondensation and their Co(II), Mn(II) and Ru(III) complexes: synthesis, characterization and catalytic activity in epoxidation of styrene . Des. Monomers Polym. , 15 : 75 – 91 .
- Kirillov , AM , Kopylovich , MN , Kirillova , MV , Haukka , M , Guedes da Silva , MFC and Pombeiro , AJL . 2005 . Multinuclear copper triethanolamine complexes as selective catalysts for the peroxidative oxidation of alkanes under mild conditions . Angew. Chem. Int. Ed. , 44 : 4345 – 4349 .