Abstract
A series of well-defined 8-shaped polymers have been synthesized by the combination of reversible addition–fragmentation chain transfer (RAFT) polymerization and click reaction. Firstly, well-defined, azide-terminated, four-armed star polymers including poly(N,N-dimethylacrylamide) and polystyrene were prepared by RAFT polymerization using pentaerythritoltetrakis(3-(S-(2-azidoethylcarbonoyl)trithiocarbonyl)propanoate) as a chain transfer agent. Then, intramolecular cyclization of the azide-functionalized four-armed precursors was conducted under highly dilution conditions using a bifunctional N,N-bis(2-propargyloxycarbonyl ethyl)-2-hydroxylethyl amine as the linker, affording 8-shaped polymers. FTIR, 1H NMR, GPC, and MLADI-TOF MS analysis confirmed that the coupling reaction proceeded via the cyclization manner.
Introduction
Precise control of polymer topologies has gained a lot of attention over the last few decades because the properties of polymers are intimately related to their chain architectures.Citation[1] Cyclic topological polymers, including single and multiple cyclic polymers, due to the absence of end groups, exhibit unique properties as compared to their linear and branched counterparts. Among these cyclic structures, bicyclic polymers, such as theta-shaped,[2–7]Citation2Citation3Citation4Citation5Citation6Citation7 8-shaped,[7,8–17]Citation7Citation8Citation9Citation10Citation11Citation12Citation13Citation14Citation15Citation16Citation17 and manacle-shaped constructions,[6,7,18,19]Citation6Citation7Citation18Citation19 are an important class of cyclic topological polymers. Although the 8-shaped polymer has been investigated the most, efficient and practical synthesis of this polymer architecture still remains challenge. 8-shaped polymer was almost exclusively prepared via inter- or intramolecular coupling reaction under highly diluted conditions.
General strategies toward the synthesis of 8-shaped polymers have been summarized in several reviews (Scheme ).[2,20,21]Citation2Citation20Citation21 The first strategy for the construction of this topology involves the reaction between two units of bifunctionally living polymers and an equimolar amount of a tetrafunctional coupling reagent (Scheme ).[9–13]Citation9Citation10Citation11Citation12Citation13 Electrostatic self-assembly of the two reactants can significantly improve the cyclization efficiency.[11–13]Citation11Citation12Citation13 A different synthetic strategy toward 8-shaped polymers utilizes intramolecular cyclization of the linear tetrafunctional polymer precursor which bears two types of complementary functionality (Scheme ).Citation[14] The third approach for the synthesis of polymers with 8-shaped architecture involves the reaction between monocyclic polymer precursor and bifunctional linker (Scheme ).[7,8]Citation7Citation8 The final synthetic method involves intermolecular coupling reaction of cyclic polymer precursor having bifunctional groups with bifunctional linker under equimolar conditions (Scheme ). However, the strategies described previously suffer from poor yields and competing side reactions, usually requiring tedious purification procedures to isolate pure bicyclic polymers.Citation[1] Additionally, most of the existing approaches do not offer the same control over molecular weight, low polydispersity, and functional group tolerance demonstrated by controlled radical polymerization (CRP), such as reversible addition–fragmentation chain transfer (RAFT) polymerization, and atom transfer radical polymerization (ATRP). RAFT is a particularly attractive approach for making cyclic precursors because of its applicability to a wide range of monomers, suitability for a broad range of functional groups and reaction conditions, and tolerance to various organic and aqueous solvents.Citation[22] Therefore, a synthetic route was sought to efficiently cyclize polymers prepared by RAFT polymerization.
Click chemistry has been used extensively since its introduction, due to their modular nature, selectivity, and high yields.Citation[23] The most popular click reaction has been the Cu(I)-catalyzed Huisgen [3 + 2] cycloaddition of a terminal alkyne and an azide to form a 1,4-disubstituted triazole.Citation[24] This coupling process reaches near-quantitative conversion in both aqueous and organic media with little or no side reactions being observed. Additionally, this highly efficient reaction tolerates a wide range of functional groups. As a result, click chemistry has emerged as an important and general methodology for constructing polymeric materials. Combining the synthetic techniques of CRP and click chemistry provides an efficient route to the preparation of cyclic polymeric materials. Several groups reported the synthesis of macrocyclic polymers via CRP and subsequent azide–alkyne coupling reactions,[1,25–27]Citation1Citation25Citation26Citation27 although the significant majority of these reports concern the linear functional polymer precursors prepared by ATRP. For example, Grayson and coworkers Citation[1] demonstrated the preparation of macrocyclic polystyrene (PS) from alkyne functionalized polystyrene (PS) prepared by ATRP via end group substitution with NaN3 and subsequent intramolecular coupling of the α-propargyl ω-azido telechelic linear precursor. Pan group reported the preparation 8-shaped PS via ATRP and azide–alkyne coupling.Citation[25] This approach proved an efficient and specific means to prepare 8-shaped polymer with end-group functionalized precursors from any monomer polymerizable by ATRP; however, we seek to expand the method to other radically polymerizable monomer classes.
Herein, we describe the synthesis of well-defined 8-shaped polymers by the combination of RAFT polymerization and click coupling. Firstly, well-defined, tetraazide-terminated, four-armed star polymers including poly(N,N-dimethylacrylamide) (PDMA) and PS have been prepared by RAFT polymerization using pentaerythritoltetrakis(3-(S-(2-azidoethylcarbonoyl)trithiocarbonyl)propanoate) (PATP) as a chain transfer agent (CTA). Then, cyclization of the azide-functionalized, four-armed precursors was conducted using N,N-bis(2-propargyloxycarbonyl ethyl)-2-hydroxylethyl amine (BPHA) as the bifunctional linker under highly dilution conditions, affording well-defined 8-shaped polymers. A series of 8-shaped polymers based on PDMA and PS, respectively, were synthesized in this paper. The structure of the 8-shaped polymer was characterized by FTIR, 1H NMR, GPC, and MLADI-TOF MS.
Experimental section
Materials
N,N-Dimethylacrylamide (DMA, Alfa Aesar, 99%) was purified by passing through a basic alumina column followed by vacuum-distillation from CaH2. Styrene (99%) was dried with CaH2 and distilled under reduced pressure before use. 2,2′-Azobis(isobutyronitrile) (AIBN, Sigma, 98%) was recrystallized twice from ethanol. N,N,N′,N′,N′′-Pentamethyldiethylenetriamine (PMDETA, Alfa Aesar, 99%) was used as received, and CuBr (Shanghai Reagents Company, China, 99%) was stirred in glacial acetic acid overnight, filtered, washed three times with alcohol, and dried under vacuum at 60 °C. Propargyl alcohol was dried with molecular sieves and distilled under reduced pressure prior to use. All other reagents and solvents were obtained from Shanghai Reagents Company and used as received.
Synthesis of PATP
Triethylamine (2.02 g, 20.0 mmol) in 5 mL of CHCl3 was added dropwise to a stirred solution of pentaerythritol tetra(3-mercaptopropionate) (1.22 g, 2.50 mmol) and carbon disulfide (1.52 g, 20.0 mmol) in CHCl3 (8 mL) at room temperature. The solution gradually turned deep yellow from light yellow and became cloudy during the addition. The solution was allowed to stir for an additional 1 h. 2-Azidoethyl 2-bromoacetate (2.29 g, 22.0 mmol) dissolved in 5 mL of CHCl3 was added dropwise, and the solution was stirred for a further 2 h. The mixture was poured into a cold solution of 10% aqueous HCl and extracted three times with ethyl acetate. The organic phase was then washed with deionized water three times and finally dried with MgSO4. After evaporation of the solvent under reduced pressure, yellow oil was obtained. The oil was purified using column chromatography on silica gel (petroleum spirits/ethyl acetate, 1/1, v/v) to obtain the PATP as a thick viscous yellow oil. (1.05 g, 36%). 1H NMR (400 MHz, CDCl3), δ (TMS, ppm): 4.30 (t, 8H, OCH2CH2N3), 4.20 (s, 8H, SCH2CO), 4.13 (s, 8H, CCH2O), 3.62 (t, 8H, COCH2CH2S), 3.50 (t, 8H, OCH2CH2N3), 2.79 (t, 8H, COCH2CH2S). 13C NMR (100 MHz, CDCl3), δ (TMS, ppm): 221.43 (C=S), 170.67 (C=O), 167.08 (C=O), 64.38 (OCH2CH2N3), 62.39 (CCH2O), 49.63 (OCH2CH2N3), 42.51 (SCH2CO), 38.24 (CCH2O), 32.85 (COCH2CH2S), 31.71 (COCH2CH2S).
Preparation of four-armed star polymers via RAFT polymerization
Polymerization of DMA or styrene was conducted at 60 °C under a nitrogen atmosphere employing PATP as a CTA and AIBN as the primary radical source. The molar ratio of [PATP]:[AIBN] was maintained at 2:1, and the initiator concentration was maintained at 4.2 mM, while the [DMA]:[PATP] molar ratio was changed for preparation of PDMA with different molecular weight. A typical RAFT polymerization procedure was as follows. DMA, PATP, AIBN, and 1,4-dioxane was sealed in a 25 mL flask. The solution was purged with nitrogen for 40 min and then placed in a preheated oil bath (60 °C) for 4.5 h. The polymerization was quenched by cooling reaction flask in an ice bath and exposing the solution to air. The polymer was precipitated in diethyl ether and then reprecipitated twice from THF/diethyl ether and dried in a vacuum oven at room temperature for 12 h.
Synthesis of BPHA
BPHA was synthesized according to the description elsewhere.Citation[28] Propargyl acrylate (27.0 g, 0.250 mol) was dissolved in a mixture of THF (34 mL) and tert-butanol (38 mL). 2-Aminoethanol (5.00 g, 80.0 mmol) was then added to the mixture. The mixture was stirred for 24 h at room temperature. Solvent was removed by a rotary evaporator. The product was purified by silica gel chromatography (ethyl acetate/petroleum ether, v/v, 1/6) to produce a pale yellow oil in 91% yield. 1H NMR (400 MHz, CDCl3), δ (TMS, ppm): 4.70 (d, 4H, HC≡CCH2), 3.64 (t, 2H, CH2CH2OH), 2.88 (t, 4H, NCH2CH2CO), 2.65 (t, 2H, NCH2CH2OH), 2.58 (t, 4H, NCH2CH2CO), 2.49 (1H, HC≡CCH2).
Synthesis of 8-shaped polymers via click reaction
PMDETA (39 mg, 0.225 mmol), four-armed star precursor PDMA (29.3 mg, Mn = 7800 g/mol, 15.0 μmol azido moiety), and 400 mL DMF were added into a 500-mL flask. After the mixture was thoroughly deoxygenated by purged with nitrogen for 30 min, and CuBr (32 mg, 0.225 mmol) was introduced into the flask, and then, the solution was purged with nitrogen for 1 h. Then, the flask was placed in an oil bath preheated at 90 °C, a previously degassed mixture of BPHA (5.0 mg, 20.0 μmol), and 20 mL DMF was added into the flask via a syringe pump at a rate of 0.5 mL/h. Once the addition was completed, the reaction was allowed to proceed for an additional 2 h before cooling to room temperature. After most of the solvents were rotated under reduced pressure, the residues were passed through a neutral alumina column to remove copper catalyst. The eluents were further concentrated and precipitated into an excess of cold diethyl ether. The above dissolution/precipitation cycle was repeated twice. After drying in a vacuum at room temperature for 12 h, the product was obtained.
Characterization
NMR spectra were recorded with a 400 MHz Bruker AV-400 NMR spectrometer. FTIR spectra in KBr pellets were recorded on a PE Spectrum One FTIR spectrometer. The molecular weights and polydispersity indexes of polymers were determined by a PL-GPC120 setup equipped with a PL HTRI RI detector, and a column set consisting of two PL gel 5 μm mixed-D columns (7.5 × 300 mm, effective molecular weight range of 0.2–400.0 kg/mol) using DMF that contained 1.0 g/L LiBr as an eluent at 80 °C at a flow rate of 1.0 mL/min. Narrowly distributed polystyrene standards over the molecular weight range of 0.5–7500 kg/mol (PS, Mainz, Germany) were utilized for calibration. MALDI-TOF mass spectrum was recorded in the reflector mode on a Bruker BIFLEXeIII mass spectrometer using a nitrogen laser (337 nm) and an accelerating potential of 20 kV. 2,5-Dihydroxybenzoic acid (DHB) (99%, Sigma) was used as the matrix.
Results and discussion
In the present work, the strategy developed for the preparation of 8-shaped bicyclic polymers is derived from the one previously reported as shown in Scheme . However, the tetrafunctional coupling reagent was replaced by a tetraazide-functionalized, four-armed star polymer, which was then cyclized through a 1,4-disubstituted 1,2,3-triazole moiety formed by an intermolecular click reaction with bialkyne as a linker under equimolar conditions (Schemes 1e and 2). Due to the high movement of the small linker in the reaction mixture, it is reasonable to consider that the linker is easy to encounter the terminal azide moiety in the star precursor and combine with it. Once one linker has reacted with an azide group, the remaining alkyne group will preferentially react with a terminal azide group in the neighboring arms within the same macromolecule via an intramolecular manner. This click reaction was very promising quantitative under very mild conditions and tolerated several functional groups. Therefore, the optimization of the reaction conditions allows the preparation of cyclic polymer with a relatively high efficiency.
Synthesis of four-armed star polymer precursors
RAFT is a well-established radical polymerization technique that allows the synthesis of polymeric architecture of well-controlled molecular weight distribution and functionality; RAFT has already been successfully employed with Cu(I)-catalyzed azide/alkyne cycloaddition, by introducing azide and alkyne groups either as end groups or as monomers. Recently, the synthesis of pentaerythritoltetrakis(3-(S-benzyltrithiocarbonyl)propionate) CTA has been reported by Mayadunne and coworkers Citation[29], of which can be applied for the preparation of four-armed star polymers with well-defined architectures. We adapted the synthetic procedure to prepare PATP, which involved the addition of carbon disulfide to a solution of the commercially available tetrathiol and triethylamine, followed by alkylation of the resulting ammonium salt with 2-azidoethyl 2-bromoacetate, affording the desired tetrafunctional CTA (PATP) in good yield. The presence of the azide groups was confirmed by 1H NMR and FTIR data as shown in experimental section.
RAFT polymerization with multifunctional CTAs has been demonstrated to be ‘living’.Citation[29] These CTAs have a linear molecular weight conversion profile and have the ability to form star block copolymers with sequential addition of monomer. Thus, a series of well-defined, four-armed star PDMA and PS were separately prepared by RAFT polymerization using PATP as the CTA and AIBN as the initiator. Due to star polymers synthesized from a tetrafunctional core, the number of arm can be accurately controlled, and the terminal functionalities can be preintroduced. All polymerizations proceeded with good control, as evidenced by low product polydispersity. Similarly, the presence of the azide end-groups in the polymer was also confirmed by 1H NMR with the CH2N3 signal at 3.42 ppm and by FTIR spectroscopy showing the characteristic azide band at 2113 cm−1 (vide infra). Although recent literatures had demonstrated that azide can undergo 1,3-dipolar cycloaddition toward electron-deficient olefins;Citation[30] however, the disappearance of azide groups during polymerization was not observed. The way to circumvent this side reaction and preserve high end-group fidelity is to keep the polymerization time short and/or work at low temperatures.
Cyclization reaction
As shown in Scheme , a four-armed star polymer precursor bearing complementary azide functionalities was subjected to the click reaction with two units of bifunctional linker (BPHA) under high dilution at 25 °C, resulting in the formation of the 8-shaped polymer. The concentration of the functional groups is the primary factor in determining whether the click reaction will prefer cyclization or condensation. In our study, concentrations of polymer above 37.5 μM favored condensation, while dilution below that concentration favored cyclization using Cu(I) catalyst (15 equiv., relative to azide group) in DMF. Due to the dilution required in the synthesis of 8-shaped polymer, a continuous addition technique was utilized to ensure an infinitesimal concentration of unreacted linker during the course of the coupling reaction. Using a syringe pump, the solution of BPHA in DMF was added at a rate of 0.5 mL/h into a DMF solution containing polymer precursor, Cu(I)Br and PMDETA. During the click reaction process, once one BPHA molecule has reacted with an azide group in the four-armed star polymer, the remaining alkyne group will preferentially react with a terminal azide group in the neighboring arms within the same macromolecule. Before the next drop of BPHA solution was introduced, the terminal alkyne groups in the previously added one have completely reacted with azide functionalities. After the addition of BPHA solution and stirring for another 2 h, the product was isolated by precipitation.
The key methods for verifying the successful cyclization were MALDI-TOF MS, NMR, FTIR, and GPC. The MALDI-TOF mass spectra of 8-shaped PDMA and its four-armed star precursor (run 3, Table ) are shown in Figure . Although the resolution of the mass spectra is relatively low due to low ionization efficiency; however, they verified that the molecular weight of the polymer samples remained almost unchanged after click cyclization because of the low molecular weight of the linker. The presence of high–molecular-weight impurities, such as condensation products, was not observed in the mass spectral data. High magnification spectra in the range of 4000–5000 g/mol (insert) show a series of peaks corresponding to PDMA (peak interval of 99 mass units). For 8-shaped polymer, each peak corresponds to the molar mass summing up the linking structure produced by the click reaction. As an example, the peak (assumed to be the adduct with H+) at 4639.24 corresponds to the product with the degree of polymerization (DP) of 28, (C5H9NO) × 28 + C37H48N12O16S12 (CTA) + C14H19NO5 (BPHA) × 2 plus H+ as 4640.87. The star polymer precursor also showed a series of the peaks corresponding to the H+ adduct. Thus, the peak (assumed to be the adduct with H+) at 4078.36 corresponds to the product with the DP of 28, (C5H9NO) × 28 + C37H48N12O16S12 (CTA), plus H+ as 4078.27. Since the 8-shaped polymer was produced from the star precursor by the addition of two BPHA molecules, their molecular weights differ by 562 mass units (found 561). This was confirmed by the two mass spectra shown in Figure .
Table 1. Molecular weight and polydispersity data for the star polymers and 8-shaped polymers.
Figure 1 MALDI-TOF mass spectra of 8-shaped PDMA (blue) and its star precursor (black) (run 3, Table ).
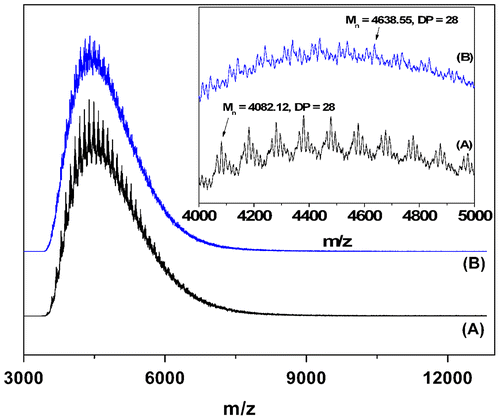
1H NMR was used to verify the chemical transformations of the end groups. As shown in Figure , small, but clearly discernible, signals at 8.48, 5.22, and 3.81 ppm are present in spectrum B, corresponding to the methylidyne proton (Hg) of 1,2,3-triazole ring, methylene proton (Hh) adjacent to the 1,2,3-triazole ring, and the methylene proton (l) next to the hydroxyl group, respectively. Additionally, signal at 3.42 ppm corresponding to methylene proton (f) next to azide group distinctly decreased, and a new signal at 4.62 ppm that belongs to the methylene proton (f′) adjacent to the 1,2,3-triazole ring is observed. Further evidence can be seen in the FTIR spectra (Figure ), by monitoring the remarkable reduction of the azide (2113 cm−1), which indicates the consumption of the azides. All these suggested that 8-shaped polymer was successfully synthesized with a relatively high efficiency by intramolucular cyclization reaction. Important and necessary to note, the as-synthesized 8-shaped polymer contained trace four-armed star polymer precursor based on 1H NMR and FTIR analysis, and the further purification was not fulfilled in this study due to the difficulties in separation.
Generally, cyclization will lead to a more compact polymer structure, and therefore, the 8-shaped polymer exhibit a longer size exclusion retention time than the four-armed star polymer precursor. Figure shows the GPC traces of the star PDMA and their cyclization products. All traces demonstrate unimodal and symmetrical peaks with narrow molecular weight distributions. However, it is noteworthy that the average molecular weights for the four-armed star polymer precursor (run 5, Table ) obtained by GPC (Mn = 13,400, PDI = 1.39) and MALDI-TOF MS (Mn = 4500, Figure ) were incomparable. These results suggested that our GPC analysis may not provide reliable results for the polymers. The difference in physical characteristics of star-shaped polymers and the linear polystyrene standards used to evaluate the molecular weights is the most likely explanation for this discrepancy.
The <Gexp> factor, the ratio of the apparent peak molar mass of the cyclic polymers with respect to their linear or star precursors derived from the GPC curves, is usually used to characterize of the formation of cyclic polymers.[31,32]Citation31Citation32 In this study, the <Gexp> values corresponding to the molecular weight of 8-shaped polymer and its star precursor are calculated and the results are listed in Figure and Table . The <Gexp> values for PDMA are in the range of 0.65–0.68, which is much lower than that of the single cyclic PS (0.82–0.85) Citation[32] as well as the 8-shaped PS (0.86–0.91).Citation[25] This difference cannot be ascribed to the cleavage of the trithiocarbonate group in the polymer, although the CTA can undergo decomposition in the presence of nucleophiles, ionic reductants, oxidant, and radical attacker or by thermolysis and by UV irradiation.[33–36]Citation33Citation34Citation35Citation36 Since mass spectral data for the four-armed star precursor and 8-shaped PDMA (run 5, Table ) have excluded the possibility of decomposition of trithiocarbonate group as mentioned before (Figure ). Additionally, low polydispersities for 8-shaped polymers can also exclude this decomposition. We speculate that the lower <Gexp> values for PDMA may be ascribed to the different backbone structure of the polymer precursors. In order to verify this assumption, the cyclization reaction was carried out on four-armed star PS precursors under identical conditions. GPC measurement was also conducted on the star precursors and their cyclization products. As expected, the elution profiles of the starting polymers and the 8-shaped polymers are unimodal and symmetrical peaks with narrow molecular weight distributions. The undesired condensation products cannot be observed, as shown by the absence of any detectable high–molecular-weight contamination in the GPC profiles (Figure ). Interestingly, the experimental <Gexp> values for PS are in the range of 0.87–0.89 after cyclization. These values are in good agreement with <Gexp> values reported in the literature for 8-shaped PS.Citation[18] However, no dependence can be found between <Gexp> and the variation of polymer molecular weights. These results indicate that the observed fluctuations of <Gexp> for PS and PDMA may be ascribed to the difference of the polymer architectures. However, the underlying reasons will be further investigated in our following studies. Owing to the ionization efficiency problems, we are unable to determine the molecular weight of star and 8-shaped PS by MALDI-TOF mass.
Conclusion
In conclusion, we have successfully demonstrated an efficient protocol for the synthesis of 8-shaped polymers by the combination of RAFT polymerization and click reaction. The synthesis procedure involves two steps. Firstly, well-defined, azide-terminated, four-armed star polymers were prepared by RAFT polymerization using PATP as the CTA. Then, intramolecular cyclization of the four-armed precursors was conducted under highly dilution condition using BPHA as the linker and CuBr/PMDETA complex as the catalyst, affording 8-shaped polymers with well-defined architecture. Successful intramolecular cyclization with a relatively high efficiency was confirmed by FTIR, 1H NMR, GPC, and MLADI-TOF MS analysis. The <Gexp> values for PDMA are in the range of 0.65–0.68, which is much lower than that of the PS (0.87–0.89). The observed fluctuations of <Gexp> values may be ascribed to the difference of the polymer architectures.
Acknowledgements
The authors thank the financial supports from the Key project of Education Department of Hunan Province (12A134), the Department of Science and Technology of Hunan Province Project (2012FJ4094, 2013FJ3035), Open Project Program of Key Laboratory of Advanced Functional Polymeric Materials of Hunan Province (12K050) and the High-tech Project of Development and Reform Commission of Hunan Province.
References
- Laurent BA, Grayson SM. An efficient route to well-defined macrocyclic polymers via “click” cyclization. J. Am. Chem. Soc. 2006;128:4238–4239.
- Tezuka Y, Tsuchitani A, Oike H. Synthesis of θ-shaped poly(THF) by electrostatic self-assembly and covalent fixation with three-armed star telechelics having cyclic ammonium salt groups. Macromolecules. 2003;36:65–70.
- Tezuka Y, Tsuchitani A, Oike H. Synthesis of polymeric topological isomers through electrostatic self-assembly and covalent fixation with star telechelic precursors. Macromol. Rapid Commun. 2004;25:1531–1535.
- Tezuka Y, Ohashi F. Synthesis of polymeric topological isomers through double metathesis condensation with H-shaped telechelic precursors. Macromol. Rapid Commun. 2005;26:608–612.
- Tezuka Y, Takahashi N, Satoh T, Tezuka KA. Synthesis of polymeric topological isomers having θ- and manacle-constructions with olefinic groups at designated positions. Macromolecules. 2007;40:7910–7918.
- Oike H, Imaizumi H, Tezuka Y. Designing unusual polymer topologies by electrostatic self-assembly and covalent fixation. J. Am. Chem. Soc. 2000;122:9592–9599.
- Sugai N, Heguri H, Ohta K, Meng QY, Yamamoto T, Tezuka Y. Effective click construction of bridged- and spiro-multicyclic polymer topologies with tailored cyclic prepolymers (kyklo-telechelics). J. Am. Chem. Soc. 2010;132:14790–14802.
- Kubo M, Hayashi T, Kobayashi H, Itoh T. Syntheses of tadpole- and eight-shaped polystyrenes using cyclic polystyrene as a building block. Macromolecules. 1998;31:1053–1057.
- Antonietti M, Fölsch KJ. Synthesis and characterization of “eight-shaped” polystyrene. Macromol. Rapid Commun. 1988;9:423–430.
- Madani El, Favier JC, Hémery P, Sigwalt P. Synthesis of ring-shaped polyisoprene. Polym. Int. 1992;27:353–357.
- Oike H, Hamada M, Eguchi S, Danda Y, Tezuka Y. Novel synthesis of single- and double-cyclic polystyrenes by electrostatic self-assembly and covalent fixation with telechelics having cyclic ammonium salt groups. Macromolecules. 2001;34:2776–2782.
- Tezuka Y, Oike H. Self-assembly and covalent fixation for topological polymer chemistry. Macromol. Rapid Commun. 2001;22:1017–1029.
- Tezuka Y, Oike H. Topological polymer chemistry. Prog. Polym. Sci. 2002;27:1069–1122.
- Schappacher M, Deffieux A. Controlled synthesis of bicyclic “eight-shaped” poly(chloroethyl vinyl ether)s. Macromolecules. 1995;28:2629–2636.
- Tezuka Y, Komiya R, Washizuka M. Designing 8-shaped polymer topology by metathesis condensation with cyclic poly(THF) precursors having allyl groups. Macromolecules. 2003;36:12–17.
- Ishikawa K, Yamamoto T, Harada H, Tezuka Y. Synthesis and topological conversion of an 8-shaped poly(THF) having a metathesis-cleavable unit at the focal position. Macromolecules. 2010;43:7062–7067.
- Schmidt BV, Fechler N, Falkenhagen J, Lutz JF. Controlled folding of synthetic polymer chains through the formation of positionable covalent bridges. Nat. Chem. 2011;3:234–238.
- Sugai N, Heguri H, Yamamoto T, Tezuka Y. A programmed polymer folding: click and clip construction of doubly fused tricyclic and triply fused tetracyclic polymer topologies. J. Am. Chem. Soc. 2011;133:19694–19697.
- Tezuka Y, Oike H. Topological polymer chemistry: systematic classification of nonlinear polymer topologies. J. Am. Chem. Soc. 2001;123:11570–11576.
- Takuya Y, Yasuyuki T. Topological polymer chemistry: a cyclic approach toward novel polymer properties and functions. Polym. Chem. 2011;2:1930–1941.
- Kricheldorf HR. Cyclic polymers: synthetic strategies and physical properties. J. Polym. Sci., Part A: Polym. Chem. 2010;48:251–284.
- Chiefari J, Chong YK, Ercole F, Krstina J, Jeffery J, Le TPT, Mayadunne RTA, Meijs GF, Moad CL, Moad G, Rizzardo E, Thang SH. Living free-radical polymerization by reversible addition-fragmentation chain transfer: the RAFT process. Macromolecules. 1998;31:5559–5562.
- Kolb HC, Finn MG, Sharpless KB. Click chemistry: diverse chemical function from a few good reactions. Angew. Chem. Int. Ed. 2001;40:2004–2021.
- Huisgen R. 1,3-Dipolar cycloaddition chemistry. In: Padwa A, editor. Synthetic applications of 1, 3-dipolar cycloaddition chemistry toward heterocycles and natural products. New York, NY: Wiley; 1984 . p. 1–1521.
- Shi GY, Pan CY. Synthesis of well-defined figure-of-eight-shaped polymers by a combination of ATRP and click chemistry. Macromol. Rapid Commun. 2008;29:1672–1678.
- Shi GY, Yang LP, Pan CY. Synthesis and characterization of well-defined polystyrene and poly(ϵ-caprolactone) hetero eight-shaped copolymers. J. Polym. Sci., Part A: Polym. Chem. 2008;46:6496–6508.
- Kempe K, Krieg A, Becer CR, Schubert US. “Clicking” on/with polymers: a rapidly expanding field for the straightforward preparation of novel macromolecular architectures. Chem. Soc. Rev. 2012;41:176–191.
- Dong YQ, Tong YY, Dong BT, Du FS, Li ZC. Preparation of tadpole-shaped amphiphilic cyclic PS-b-linear PEO via ATRP and click chemistry. Macromolecules. 2009;42:2940–2948.
- Mayadunne RTA, Jeffery J, Moad G, Rizzardo E. Living free radical polymerization with reversible addition−fragmentation chain transfer (RAFT Polymerization): approaches to star polymers. Macromolecules. 2003;36:1505–1513.
- Mahoney JM, Smith CR, Johnston JN. Brønsted acid-promoted olefin aziridination and formal anti-aminohydroxylation. J. Am. Chem. Soc. 2005;127:1354–1355.
- Rique-Lurbet L, Schappacher M, Deffieux A. A new strategy for the synthesis of cyclic polystyrenes: principle and application. Macromolecules. 1994;27:6318–6324.
- Gan Y, Dong D, Hogen-Esch TE. Effects of lithium bromide on the glass transition temperatures of linear and macrocyclic poly(2-vinylpyridine) and polystyrene. Macromolecules. 1995;28:383–385.
- Quinn JF, Barner L, Barner-Kowollik C, Rizzardo E, Davis TP. Reversible addition−fragmentation chain transfer polymerization initiated with ultraviolet radiation. Macromolecules. 2002;35:7620–7627.
- Chong YK, Moad G, Rizzardo E, Thang SH. Thiocarbonylthio end group removal from RAFT-synthesized polymers by radical-induced reduction. Macromolecules. 2007;40:4446–4455.
- Xu JT, He JP, Fan DQ, Wang XJ, Yang YL. Aminolysis of polymers with thiocarbonylthio termini prepared by RAFT polymerization: the difference between polystyrene and polymethacrylates. Macromolecules. 2006;39:8616–8624.
- Xu JT, He JP, Fan DQ, Wang XJ, Yang YL. Thermal decomposition of dithioesters and its effect on RAFT polymerization. Macromolecules. 2006;39:3753–3759.