Abstract
A monomer, vanillin oxime (VO), has been synthesized from vanillin and hydroxylamine hydrochloride, and its terpolymer resin VO-formaldehyde-p-hydroxyacetophenone (VOFHA) has also been synthesized by condensation of VO, formaldehyde, and p-hydroxyacetophenone (HA) in 1:2:1 M proportion in the presence of hydrochloric acid. The structures of monomer and terpolymer have been investigated by FT-IR and 1H NMR techniques. The number-average molecular weight and polydispersity index of the terpolymer were found to be 5337.6 g/mol and 1.33, respectively, by gel permeation chromatography. Apparent activation energies of the thermal decomposition of the terpolymer were determined using the Flynn–Wall–Ozawa method. Terpolymer (VOFHA) has shown highest zone of inhibition as compare to other terpolymer and standard drugs. This novel bio-responsive antimicrobial terpolymer shows promise for use in the control of medical devices associated infections.
1. Introduction
Nowadays, the synthesis of functional monomers and their polymers and use in the synthesis of new functional polymers have attracted considerable interest. Recently, a series of high-molecular weight poly(aryl ether ketone)s with phosphorus and nitrogen on the side and main chains were synthesized by a nucleophilic substitution polycondensation of 1,2-dihydro-4-(4-hydroxyphenyl)-phthalazin-1(2H)-one with 1,1′-bis(4-hydroxyphenyl)-metheylene-bispheny-1-oxophosphine oxide (DOPO-PhOH) and 4,4′-difluorobenzophenone at various molar ratios.Citation[1] Two functional bisbenzoxazine monomers containing carboxylic groups, bisphenol-A-based bisbenzoxazine, and phenolphthalein-based bisbenzoxazine have been prepared and further polymerized via ring-opening polymerization.Citation[2] Hydrophilic–hydrophobic terpolymers containing poly(ethylene glycol) (PEG) and two different polyester units: butylene terephthalate and butylene linoleate have been synthesized as new scaffolding materials in tissue engineering applications.Citation[3] The 2-methacryloxyacetophenone (MAP) was prepared and subjected to suspension polymerization with divinylbenzene (DVB). The differently sulfonated MAP–DVB cross-linked copolymer cationic exchange resins were prepared by sulfonation with a fixed volume of concentrated sulfuric acid in an oil bath, which could sulfonated resins could be utilized as novel carriers for drug delivery.Citation[4] Organo clay-modified alkyd resins were synthesized, and these modified alkyd resins were cured with different ratios of melamine–formaldehyde resin for the first time. Alkyd resins were blended with 30 and 40% of a commercial melamine–formaldehyde resin. These resins are suitable for manufacturing of high-performance industrial baking enamels.Citation[5]
Moreover, p-chloroacetophenone oxime, p-hydroxy benzaldehyde oxime, 4-acetylpyridine oxime, and 8-hydroxyquinioline-based terpolymers were synthesized in the presence of an acid with excellent biocidal properties.[6–11]Citation6Citation7Citation8Citation9Citation10Citation11 The oxime click reaction is shown to be a straightforward methodology for the synthesis of PEG-polysaccharide di-block copolymers. The method is applicable to unmodified polysaccharides with a reductive end as demonstrated for dextran, hyaluronic acid, and chitosan. Notably, the oxime click reaction is applied for the first time to the end modification of polysaccharides.Citation[12] A series of anthracenone-based oxime ethers and -esters were synthesized in order to evaluate their antiproliferative activity for the development of novel antiproliferative drugs, based on tubulin interaction.Citation[13] Vanillin can be produced on a commercial scale by depolymerizing renewable lignin. Plant/crop-based (sometimes termed bio-based) resources are material derived from a range of plant systems, primarily agricultural crops, forestry products, and processing streams in the food, feed, and fiber industries. Electrochemical reductive polymerization of di-vanillin in aqueous sodium hydroxide using a lead cathode gives polyvanillin in 91% yield.Citation[14] In order to generate a highly bio-based thermoset for use in polymer composites, a lignin-derived chemical, vanillin, was methacrylated in a two-step, one-pot synthesis to produce a vinyl ester resin with a 1:1 mol ratio of a mono-functional monomer with excellent thermal stability.Citation[15] Recently, vanillin-incorporated poly(lactic-co-glycolic acid) (PLGA) films and scaffolds were fabricated to evaluate the effects of vanillin on the inflammatory responses and extracellular matrix formation in vitro and in vivo. The incorporation of vanillin to PLGA films induced hydrophilic nature, resulting in the higher cell attachment and proliferation than the pure PLGA film. Vanillin also reduced the generation of reactive oxygen species in cells cultured on the pure PLGA film and significantly inhibited the PLGA-induced inflammatory responses in vivo, evidenced by the reduced accumulation of inflammatory cells and thinner fibrous capsules, which make them promising candidates in the future biomedical applications.Citation[16] Moreover, renewable resource-based furan resins were prepared with good thermal and biocidal properties for biomedical applications.[17–19]Citation17Citation18Citation19
In this work, the synthesis and characterization of terpolymer derived from vanillin, p-hydroxyacetophenone (HA), and formaldehyde in the presence on an acid as catalyst is reported. The obtained terpolymer was fully characterized by Fourier transform infrared spectroscopy (FTIR), proton nuclear magnetic resonance (1H NMR) spectroscopy, and gel permeation chromatography (GPC). Its thermal properties were determined by thermogravimetric (TG) analysis and differential scanning calorimetry, and antimicrobial properties were studied by cup-well method (antibacterial) and food poisoned method (antifungal). This polymer possibly could be used as an antimicrobial polymer.
2 Experimental
2.1 Chemicals and reagents
Vanillin (Ranbaxy, Mumbai), and HA (SRL, India) were purified by rectified spirit. Formaldehyde (37%) (AR grade, Merck) was used as received. All other chemicals, solvent, and indicators were analytical grade procured from Thomas Baker, Mumbai, India. Double-distilled water was used for all the experiments.
2.2 Preparation of monomer: vanillin oxime (VO)
Hydroxylamine hydrochloride (1.55 g, 0.02 mol) and sodium acetate trihydrate (5.44 g, 0.04 mol) in water (20 mL) were heated on a steam bath. To this hot solution, solid vanillin (3.04 g, 0.02 mol) was added. Swirl the flask until all of the vanillin has first melted and then completely dissolved with continuous heating the solution on the steam bath for another 5 min. Remove the flask from the steam bath, and allow the mixture to stand first at room temperature and then in an ice bath for crystallization, collect the product by suction filtration, using a little water for rinsing and washing. Yield: 3.1 g (0.19 mol, 90%), melting point: 116 °C, density: 1.25 g/cc.
IR (KBr, cm−1): 3328.5, 1886.9, 1664.2, 1642.8, 1608.3, 1589, 1518.8, 1364.17, 1314.9, 1271.98, 1177.89, 1108.3, 1089.5, 1012.35, 942.05, 827.07, 781.58, 724.34, 664.6, and 640.1.
1H NMR [DMSO/TMS, (ppm), 400 MHz]: 10.83 (s, 1H, –C=N–), 9.6 (s, 1H, –NO
), 7.45–7.47 (m, 3H, Ar–
), 6.75–6.77 (s, 1H, Ar–O
), 3.344 (m, 3H, –OC
3), 2.5 (s, DMSO).
2.3 Preparation of terpolymer: vanillin oxime-formaldehyde-p-hydroxyacetophenone
A mixture of vanillin oxime (VO) (0.01 mol); condensing reagent formaldehyde (0.02 mol); and comonomer, p-hydroxy acetophenone (0.01 mol) was taken in a round bottom flask. About 2.0 mL of 6.0 M HCl was added slowly to the reaction mixture, and the contents were refluxed at 120 °C for 6 h on an oil bath with periodical shaking. The solid resinous product was removed immediately from flask. It was washed with cold water and dried. The shinning blue colored resins separated out. It was washed with warm water and methanol to remove unreacted monomers. Then, it was extracted with diethyl ether to remove excess of copolymers (vanillin oxime-formaldehyde (VOF) and 4-hydroxyacetophenone-formaldehyde), which might be present along with VOFHA terpolymer resin. Finally, the terpolymers were air-dried. The dried sample was then dissolved in 10% NaOH and regenerated using 1:1 (v/v) HCl/water. It was filtered off and cured in an air oven at 60 °C for 48 h. After completion of the reaction, the mixture was poured in ice-cold water and washed with alcohol, dried in vacuum and used for spectral and GPC characterization. Herein, unimodal gel permeation chromatogram clearly indicates that terpolymer resin with larger extent of VOFHA. Scheme is shown in Figure .
IR (KBr, cm−1):3160, 1664.19, 1609.3, 1513.58, 1439.1, 1362.59, 1279.56, 1224.6, 1168.9, 1111.3, 984.2, 840.0, 610.
1H NMR [DMSO/TMS, (ppm), 400 MHz]: 10.61 (s, 1H, ––), 9.60 (w, 1H,
), 7.77–7.92 (m, 3H,
, (1 (VO + 2 (p-hydroxy acetophenone)), 6.82–6.91 (m, 1H,
), 3.5–3.78 (m, 2H,
), 3.35(m, 3H, –
), 3.77 (m, 2H), 2.87 (s, 3H, –
).
= 5337.6 g/mol,
= 7099 g/mol,
= 1.33).
2.4 Spectral characterizations
The infrared (FT-IR) spectrum for identify the linkages and functional groups was recorded at the frequency range of 4000–400 cm−1 on PerkinElmer 2000 FT-IR spectrometer. 1H NMR (400 MHz) spectrum was recorded on Bruker DPX-300 spectrometer using DMSO-d6 as the solvent and TMS as internal standard.
2.5 GPC analysis
The average molecular weight and its polydispersity index were determined with GPC analysis. It was performed with a set up consisting of a Waters 600 pumps and 2 ultra styragel columns (104, 500 Å) with tetrahydrofuran (THF) as the eluent at a flow rate of 1 mL/min equipped with Waters differential refractometer and calibrated with the standard linear PSt.
2.6 Thermal analyses
Differential scanning calorimetry (DSC) curves were recorded with a Mettler Toledo DSC-2007 apparatus. A sample was heated from −50 to 250 °C with a heating rate of 10 °C/min under nitrogen atmosphere. Thermal gravimetric analysis (TGA) was carried out using a Mettler Toledo Star System AG 2007 Module. The results were acquired with heating rates of 10, 20, 30, and 40 °C/min under nitrogen gas from 30 to 600 °C at constant flow rate of 80 mL/min. The purpose of this study is to provide trustworthy kinetic data for prolonging life time estimation.
2.7 Antimicrobial assessment
Pathogenic bacteria viz Bacillus subtilis, Escherichia coli, Staphylococus aureus, and Pseudomonas aeuroginosa were used for antibacterial activity (cup or well method). Pathogenic fungi viz. Alternaria solani and Fusarium oseysporum were used for antifungal activity studies by poisoned food techniques).Citation[17] Concentrations of samples for antibacterial and antifungal activity were taken as 250 μg/mL.
3 Results and discussion
3.1 Solubility
The terpolymer resin VOFHA was soluble in solvents like N,N dimethylformamide, THF, and dimethylsulfoxide (DMSO). The resin was insoluble in non-polar solvents such as benzene, toluene, hexane, acetone, chloroform, ethanol, and isopropanol.
3.2 Spectral analysis
3.2.1 FTIR spectra
FTIR spectroscopy is a useful tool for the identification of presence/ absence of functional groups in a terpolymer. The spectra of monomer VO and terpolymer VOFHA are depicted in Figure . The peak of –OH/N–O stretching of VO shifts from 3328.5 to 3160.4 cm−1 and from 1012.3 to 984.2 cm−1, respectively, and their intensity has been reduced significantly after physically cross-linking, which may be due to the intramolecular hydrogen bond interaction between 4-hydroxyacetophenone and VO. The absorption band at 1609.2 and 1513 cm−1 is characteristic peaks of aromatic stretching vibration from VOFHA and absorption peak at 1664.2 correspond to C=N stretching of oxime groups of monomer moieties. Appearance of broad peak at 1362.6 to 1439.1 cm−1 in terpolymer clearly confirmed the presence of methylene (CH2) stretching between VO and 4-hydroxyacetophenone. The characteristic stretching vibration absorption of benzene ring corresponds to three peaks at 1609.3, 1513, and 840 cm−1, respectively. The peak at 1279.6 cm−1 corresponds to bending vibrations of phenolic hydroxyl group. The peak at 1111.3–1168.9 cm−1 is attributed to C–O stretching.
3.2.2 1H NMR spectra
The 1H NMR spectrum of the monomer has very sharp proton signals. However, after polymerization, the peaks are broad and more numerous. The broadening of the peaks in the spectrum is attributed to the polymerization. The 1H NMR spectra of the monomer VO and its terpolymer VOFHA are shown in Figure , and the spectral data are presented in Table . The 1H NMR spectrum of VO is shown in Figure . The methoxy protons gave a resonance signal at 3.34 ppm. The methyne and hydroxyl protons of the aldoxime groups are observed at 10.83 and 9.6 ppm, respectively. The signals in the region at 7.45–7.60 ppm are assigned to all the protons of the aromatic ring, and the signal obtained at 6.71–6.77 ppm is attributed to the –OH groups of VO and 4-hydroxyacetophenone moieties. The appearance of new signals in the region 3.40–3.78 ppm clearly confirms the presence of methylene linkage between VO and 4-hydroxyacetophenone moieties through polycondensation mechanism. A signal appeared at 2.5 ppm is due to residual protons of solvent DMSO-d6 in both spectra. The aromatic protons of the aromatic rings in (1 + 2) pattern form gave signals ranging from 7.77 to 7.92 ppm (Figure ).
Table 1. 1H NMR spectral data of monomer VO and its terpolymer VOFHA resin.
The ratio of monomer, comonomer, and condensing unit in VOFHA was determined by 1H NMR method (17). It was determined by comparing the integrals (or intensity of signals) of phenolic group (for VO unit), methylene backbone (for condensing unit, formaldehyde), and methyl (for 4-hydroxyacetophenone unit) region in the spectrum. Molar fractions of the comonomer units (m1, m2 and m3) in terpolymer using 1H NMR analysis data were calculated according to the following equations:(1)
(2)
(3)
where Am1, Am2, and Am3 are the normalized areas (or intensity) per H from the corresponding functional groups of the monomer unit regions; Atotal is the total area of proton for carbon atoms in the terpolymer; n1, n2, and n3 are integers of proton(s) in the functional group of monomer units; a, b, and c are the integers of proton in the monomer units; in the case of (m1 + m2 + m3) = 1, monomer unit ratios can be calculated from Equations (1)–(3) using the following simplified form:(4)
(5)
(6)
The obtained results by 1H NMR integral areas are summarized as m1 = 1.23, m2 = 0.85, and m3 = 0.59 mol%.
3.3 GPC measurements
The molecular weight of the terpolymer was measured by GPC using THF as the solvent (Figure ). The number-average molecular weight (Mn), weight-average molecular weight (Mw), and the dispersity of the terpolymer were 5.3, 7.9 kDa, and 1.33, respectively.
3.4 Thermal properties
3.4.1 DSC analysis
In differential scanning calorimetry (DSC), the difference in heat flow between a sample and a reference is measured under precisely controlled thermal conditions. Coating generally possesses one or more characteristic transitions, including (1) the glass transition (Tg) or a transition related to change in specific heat; (2) narrow endothermic peak related to fusion or melting; (3) broader endothermic peaks caused by the volatization of low-molecular weight materials, dissociation, or decomposition; (4) exothermic peak brought about a physical process or a chemical process such as cross-linking reaction. In non-isothermal or rising temperature DSC experiment, the glass transition coincides with a relatively sharp increase in heat flow to the polymer and a corresponding increase in specific heat. Several techniques can be used in the assignment of a DSC-Tg, including the onset, midpoint, and end point of the transition; in practice, the Tg is most commonly assigned to midpoint of stepwise specific heat increment. The glass transition temperature (Tg) is observed at 88 °C as an endothermic stepwise increase in the heat flow or heat capacity. All endothermic peak values were obtained at higher temperature for faster heating rates. The endothermic transition is characterized by noting the following parameters: Tonset (endothermic onset temperature obtained by extrapolation of the steepest portion of the initial side of the endotherm), Tp (endothermic temperature of the peak position), and (the heat of melting calculated by the measurement of area under endothermic transition) are tabulated in Table . The dynamic DSC curve for resin at four different heating rates is shown Figure . All curves shows one exothermic peak regardless of the heating rate and shift toward higher temperature as heating rates increases. Because of thermal hystersis, peak temperature was different according to different heating rates. Since resin was preheated so free water molecules were evaporated. Only one broad endotherms appeared in the range 106–141 °C may probably be due to melting transition and also probably some bound water present in terpolymer. It is known that the higher melting points of polymers are associated with many factors including inter- and intramolecular interactions through hydrogen-bonded functional linkages and structural regularity and rigidity of macromolecules.Citation[20]
Table 2. DSC data for terpolymer at different heating rates.
TG-DTG thermograms at different scanning rates of 10, 20, 30, and 40 °C/min are shown in Figure . Terpolymer exhibited a two-step degradation pattern with initial degradation temperature range around 105–147 °C. The first degradation peak is due to the expulsion of bound water content and side group elimination. While the second step degradation ranging 430–612 is due to the presence of main chain scission through the methylene linkages between thermally stable aromatic moieties in the polymeric backbone. The TGA thermograms exhibited 50 wt.% losses at 340 °C and a char residue of 4% at 600 °C. In addition, the results obtained from these analyses are completely tabulated in Table .
Figure 6 TG-DTG plots obtained for terpolymer HQFCA in nitrogen atmosphere at different heating rates.
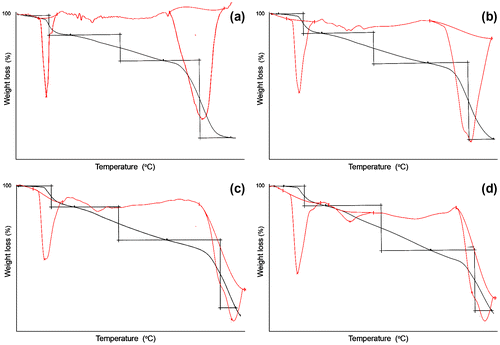
Table 3. TG-DTG data for VOFHA at different heating rates.
Moreover, Flynn–Wall–Ozawa method Citation[18] has been used to determine the activation energy from dynamic tests by plotting the logarithm of heating rate as a function of the inverse of the temperature at different conversions. It can be given as(7)
where β is the heating rate, α is the mass loss, A is the pre exponential factor, R is the universal gas constant, T is the absolute temperature, and Ea is the activation energy of the thermal decomposition reaction. The values of the activation energy (Ea) for a specific mass loss can be calculated from the slope of the plot of ln β versus 1/T at different heating rates.
Flynn–Wall–Ozawa method is an integral method also being independent of the degradation mechanism. Figure illustrated the plots of ln β versus 1000/T at varying conversion (0.05 ⩽ α ⩽ 0.80). The apparent activation energies of each thermal decomposition steps were calculated from the slopes, and the values are given in Table . The mean value of apparent activation energy of thermal decomposition was found to be 13.7 kJmol−1.
Figure 7 Typical plots of ln β vs. T−1 at several conversion values in the range from α = 0.1 to 0.8.
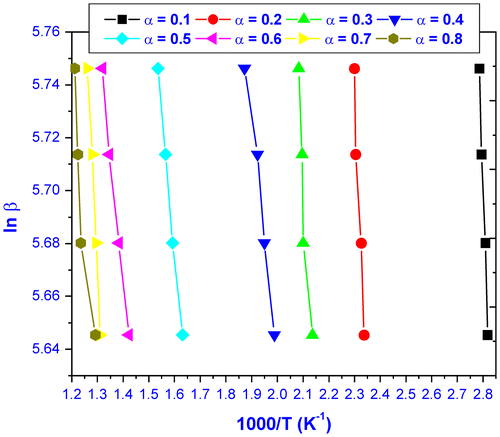
Table 4. Ea values of the thermal decomposition for a VFUMA from Flynn–Wall–Ozawa expression.
Solid-state degradation mechanism of terpolymer was determined by Craido method as an earlier reported work.Citation[18] Figure shows the Z(α) – α master and experimental curve of the terpolymer. The result indicates that terpolymer undergoes through D2 (two dimensional diffusion) mechanism which means it releases monomer, dimmer, and trimer by chain scission and cationic biocides by side group elimination diffuses onto cell wall of microbes for the achievement of efficient biocidal material.
3.5 Antimicrobial activity of terpolymer
This was to be realized by synthesizing multifunctional polymers with biocidal groups and explore them regarding their antimicrobial potential as coatings application for biomedical devices.Citation[21] This type of resin may have a mechanism of action similar to antimicrobial peptides as they may induce membrane permeation via membrane disintegration or pore formation, accompanied by a loss of bacterial membrane potential.
The antibacterial mechanism of this polycationic disinfectant can be summarized in the following six steps: (1) adsorption onto the bacterial cell surface; (2) diffusion through the cell wall; (3) binding to the cytoplasmic membrane; (4) disruption of the cytoplasmic membrane; (5) it is possible that by the further action of the quaternary iminium groups, intracellular contents are leaked, such as K+ ions, DNA, and RNA; (6) finally, the death of bacteria cells is led to probably. The antimicrobial activity of the synthesized terpolymer VOFHA was determined by in vitro with the cup or well method and poisoned food technique method against a variety of bacteria and fungi. Comparative studies of the VOFHA and previously reported CAOFU and CAOFUBA with respect to standard drugs.Citation[22] Ciprofloxacin and amphotericin-B were used as standards for antibacterial and antifungal activity, respectively. The tested terpolymer VOFHA was dissolved in DMSO with concentration of 250 μg/mL. The inhibition zone of microbial growth produced by VOFHA was measured in millimeters. DMSO alone showed no inhibition zone. The results are illustrated in Table and Figure . Some of the properties may have affected their antimicrobial activity such as molecular weight, spacer length between active site and polymer, lipophilic character, functional group and concentration. According to this conclusion, this terpolymer can be suggested as antimicrobial polymers. Polymeric antimicrobial agents have the advantage that they are non-volatile and chemically stable and do not permeate through skin.Citation[23]
Table 5. Comparitive antimicrobial activities of VOFHA and CAOFUBA.
4 Conclusions
Solution polymerization of three monomers involving bio-based new monomer VO and p-HA with formaldehyde was carried out to obtain a terpolymer. The structure of monomer and terpolymer has been characterized by FT-IR, 1H NMR, GPC, DSC, and TG-DTG. From DSC analyses, the terpolymer showed Tg was at 88 °C. Thermo-stability of the terpolymer was determined by TG analysis, and the 50% weight loss temperatures of were found to be in the range of 348–370 °C. The activation energies for the thermal degradation of VOFHA in nitrogen obtained using the FWO method were 13.7 kJ/mol. The analysis of the results obtained using the Craido method and master plots method showed that the degradation mechanism of terpolymer in nitrogen is a D2 process. According to the results of biological activity, this terpolymer may be used in various applications as antimicrobial agents.
Acknowledgements
Author gratefully acknowledges Prof. Suresh C. Ameta, Pacific University, Udaipur, for encouragement.
References
- Xie X, Wang Z, Zhang K, Xu J. Novel phosphorous–nitrogen containing poly(aryl ether ketone)s: synthesis, characterization, and thermal properties. Des. Monomers Polym. 2013;16:38–46.
- Zou T, Li S, Huang W, Liu X. Comparison of two bisbenzoxazines containing carboxylic groups and their thermal polymerization. Des. Monomers Polym. 2013;16:25–30.
- Fray ME, Wagner HD. Influence of PEG molecular masses on electrospinning of new multiblock terpoly(ester-ether-ester)s. Des. Monomers Polym. 2013;15:547–559.
- Doraswamy K, Venkata-Ramana P. Synthesis, characterization, and evaluation of differently sulfonated resins as novel carriers for drug delivery. Des. Monomers Polym. 2013;16:56–66.
- Bal A, Acar I, Iyim TB, Guclu G. A novel type of organo clay containing alkyd-melamine formaldehyde resins. Int. J. Polym. Mater. Polym. Biomater. 2013;62:309–313.
- Chauhan NPS. Structural and thermal characterization of macro-branched functional terpolymer containing 8-hydroxyquinoline moieties with enhancing biocidal properties. J. Ind. Eng. Chem. 2013;19:1014–1023.
- Chauhan NPS, Ameta R, Ameta SC. Synthesis, characterization and thermaldegradation of substituted acetophenone based terpolymers having biological activities. J. Macromol. Sci. Part A Pure Appl. Chem. 2011;48:482–492.
- Chauhan NPS, Ameta SC. Preparation and thermal studies of self-crosslinked terpolymer derived from 4-acetylpyridine oxime, formaldehyde and acetophenone. Polym. Degrad. Stab. 2011;96:1420–1429.
- Chauhan NPS. Terpolymerization of p-acetylpyridine oxime, p-methylacetophenone and formaldehyde, and its thermal studies. J. Therm. Anal. Calorim. 2012;110:1377–1388.
- Chauhan NPS, Ameta R, Ameta SC. Synthesis, characterization, and thermal degradation of pchloroacetophenone oxime based polymers having biological activities. J. Appl. Polym. Sci. 2011;122:573–585.
- Chauhan NPS. Spectral and thermal investigation of designed terpolymers bearing p-acetylpyridine oxime moieties having excellent antimicrobial properties. Des. Monomers Polym. 2013;16:543–555.
- Caballal RN, Muller AHE. Synthesis of polysaccharide-b-PEG block copolymers by oxime click. Chem. Commun. 2012;48:3781–3783.
- Surkau G, Bohm KJ, Muller K, Prinz H. Synthesis, antiproliferative activity and inhibition of tubulin polymerization by anthracenone-based oxime derivatives. Eur. J. Med. Chem. 2010;45:3354–3364.
- Amarasekara AS, Wiredu B, Razzaq A. Vanillin based polymers: I. An electrochemical route to polyvanillin. Green Chem. 2012;14:2395–2397.
- Stanzione JF, Sadler JM, La-Scala JJ, Reno KH, Wool RP. Vanillin-based resin for use in composite applications. Green Chem. 2012;14:2346–2352.
- Lee Y, Knon J, Khang G, Lee D. Reduction of inflammatory responses and enhancement of extracellular matrix formation by vanillin-incorporated poly(lactic-co-glycolic acid) scaffolds. Tissue Eng. Part A. 2012;18:1967–1978.
- Chauhan NPS. Preparation and thermal investigation of renewable resource based terpolymer bearing furan rings as pendant groups. J. Macromol. Sci. Part A Pure Appl. Chem. 2012;49:655–665.
- Chauhan NPS. Facile synthesis of environmental friendly halogen-free microporous terpolymer from renewable source with enhanced physical properties. Des. Monomers Polym. 2012;15:587–600.
- Chauhan NPS. Isoconversional curing and degradation kinetics study of self-assembled thermo-responsive resin system bearing oxime and iminium groups. J. Macromol. Sci. Part A Pure Appl. Chem. 2012;49:706–719.
- Chauhan NPS, Kataria P, Chaudhary J, Ameta SC. Synthesis, characterization, and thermal studies of terpolymers derived from vanillin, furfural, and halo-substituted acetophenones. Int. J. Polym. Mater. 2012;61:57–71.
- Merchan M, Sedlarikova J, Vesel A, Machovsky M, Sedlarik V, Saha P. Antimicrobial silver nitrate-doped polyvinyl chloride cast films: influence of solvent on morphology and mechanical properties. Int. J. Polym. Mater. Polym. Biomater. 2013;62:101–108.
- Chauhan NPS, Ameta R, Punjabi PB, Ameta SC. Synthesis, characterization and antimicrobial properties of p-chloroacetophenone oxime based furan resins. Int. J. Polym. Mater. Polym. Biomater. 2012;61:1102–1114.
- Kenawy AER, Worley SD, Broughton R. The chemistry and applications of antimicrobial polymers: a state-of-the-art review. Biomacromolecules. 2007;18:1359–1384.