Abstract
Fluorescent and glucose-sensitive hyperbranched poly(amidoamine)s (HPAMAMs-B) were synthesized via Michael addition of 1-(2-aminoethyl) piperazine, methyl acrylate, and 3-aminobenzeneboronic acid (APBA). These hyperbranched polymers emitted chartreuse photoluminescence and showed emission band at 378 nm under excitation wavelength. The intensity of emission band was enhanced with increasing the amount of APBA in HPAMAMs-B. The glucose sensitivity of HPAMAMs-B has been investigated at different glucose concentrations and pH conditions. These hyperbranched polymers showed potential for biomedical applications such as fluorescence imaging technology and glucose detecting sensors.
1. Introduction
In order to develop intelligent drug delivery vehicles, fluorescent polymers are emerging as agents for real-time imaging technologies allowing tracking of carrier locations.[Citation1] Self-emission fluorescent polymers can be used to directly analyze intercellular behavior (through fluorescence microscopy) without requiring additional fluorescence labeling (e.g. with organic dyes, nanoclusters, or quantum dots), which may allow a decrease of analysis time as well as an increase in selectivity and sensitivity of the methods.[Citation2] Among them, hyperbranched fluorescent polymers have received much attention due to their three-dimensional structures and potential building blocks for the construction of organized functional materials. In addition, hyperbranched fluorescent polymers are usually prepared by facile one-pot synthesis from specific monomers with branching potential.[Citation3–Citation8] For example, we have reported the hyperbranched poly(amidoamine)s (HPAMAMs) that synthesized by Michael addition of 1-(2-aminoethyl) piperazine (AEPZ) and methyl acrylate (MA) can emit bright blue fluorescence intensity under excitation wavelength.[Citation9,Citation10] The fluorescence intensity of the polymers increased correspondingly with the increasing tertiary nitrogen in the molecular structure by alkylation of the residual amino protons of HPAMAMs with propylene oxide.[Citation11] In particular, there has been increased research interest in the development of glucose-sensitive polymeric systems that exhibit different fluorescent level in response to changing glucose concentration, so as to mimic the natural response of the body. This could lead to better control of blood glucose levels in diabetic patients.[Citation12] This approach involves an enzyme-substrate reaction that results in a change of microenvironment.
The aim of the present work was to develop a facile and one-pot method to prepare hyperbranched polymers with striking fluorescence properties. Using hyperbranched fluorescent polymers based on fluorescent response, we are able to detect glucose both visually and spectrally. Scheme shows the synthesis process for hyperbranched fluorescent poly(amidoamine)s from AEPZ, MA, and 3-aminobenzeneboronic acid (APBA).
2. Experimental
2.1. Materials
AEPZ, APBA, and MA were purchased from Sigma-Aldrich and used as received. All reagents and solvents of analytical grade are purchased from commercial suppliers and used without further purification unless stated otherwise.
2.2. Polymerization
To prepare the hyperbranched fluorescent and glucose-sensitive polymers HPAMAMs-B, the monomers, MA, AEPZ, and APBA were firstly added into a 10 mL glass tubes. The molar ratio of these three monomers was controlled as listed in Table . Then, the polymerization was carried out at 80 °C for 48 h in a sealed glass tube after three freeze-pump-thaw cycles. The white solid sample was dissolved in DI water. The purification step via precipitation in diethyl ether can effectively erase the unreacted monomer or low-molecular weight products to narrow the molecular weight distribution. The feed molar ratio of AEPZ, MA, and APBA is controlled at 30:70:0, 30:65:5, 30:57:13, 30:52:18 for HPAMAMs, HPAMAMs-B1, HPAMAMs-B2, and HPAMAMs-B3, respectively.
Table 1. Molecular characteristics of fluorescent and glucose-sensitive HPAMAMs-B.
2.3. Characterization
1H NMR spectra were recorded on a 300 MHz Varian Mercury 300 at ambient temperature. Gel permeation chromatographic analysis was carried out using a Waters 1525 pumping system (USA) at the flow rate of 0.5 mL/min with an Ultrahydrogel 500 column (Waters). The eluent was DI water. UV–visible spectra were obtained using a Shimadzu UV-2550 spectrometer. Steady-state fluorescence spectra at room temperature were measured with a HITACHI F-4600 fluorescence spectrophotometer.
2.4. Detection of glucose sensitivity of polymers
To investigate the glucose fluorescence sensitive property of as-prepared polymers, 5 mg of polymer was added to 5 mL of glucose aqueous solution and the concentration of glucose was controlled at 0–0.8 mg/mL. After 3 min of incubation at 37 °C, the fluorescent intensity was detected by fluorescence spectrophotometer with λex = 310 nm.
3. Results and discussion
The molecular weight (Mn) and yields of these hyperbranched polymers were collected in Table . Figure (A) showed the 1H NMR spectra of hyperbranched fluorescent poly(amidoamine)s without and with APBA moiety (HPAMAMs-B). For the HPAMAMs sample, the protons neighboring primary and secondary amines appeared at 2.00–3.30 ppm. After adding APBA for synthesis of HPAMAMs-B, new peaks at 6.50–7.20 ppm appear, which was due to the protons from phenyl ring in APBA.[Citation13] The intensity of these peaks from phenyl ring gets stronger as the amount of APBA increases, which indicated that more APBA structures have been covalently linked to HPAMAMs. FT-IR spectroscopy was also employed to characterize the compositions of fluorescent and glucose-sensitive HPAMAMs. As shown in Figure (B), the characteristic absorption peak of the HPAMAMs at 1635 and 1546 cm−1 can be observed which is contributed to the amide bond, and the polymerization successfully occurred. With increasing ratio of APBA to AEPZ, the strength of the characteristic absorption peaks belonging to –OH at about 3299 cm−1 enhanced gradually. And the obvious peaks at 2949 and 2820 cm−1 are contributed to the N–H stretching vibration.[Citation14] In addition, the structure of benzene ring cannot be observed from FT-IR spectra due to relatively low amount of APBA in the polymers.
HPAMAMs displayed blue photoluminescence (PL) under 365 nm irradiation according to the previous reports.[Citation9] However, HPAMAMs modified with APBA showed strong chartreuse PL, as shown in Figure (A). With the increasing amount of APBA in polymers and concentration of polymers, PL of HPAMAMs-B is enhanced gradually.[Citation15] UV–visible spectrometer was used to investigate the UV absorption properties of HPAMAMs-B polymers (Figure (B)). For the sample of HPAMAMs, an absorption band at ca. 308 nm can be found. The highest absorption peak exhibits a red shift with increasing the amount of APBA in the polymers, and a shoulder peak at ca. 350 nm increases gradually as well, which presents the n→π* energy changes of nitrogen atoms.
Figure 2. Illumination photographs of aqueous solutions of HAMAMs, HAMAMs-B1, HAMAMs-B2, and HAMAMs-B3 with different concentrations under UV irradiation (A) and UV–vis spectra of HAMAMs, HAMAMs-B1, HAMAMs-B2, and HAMAMs-B3 (B).
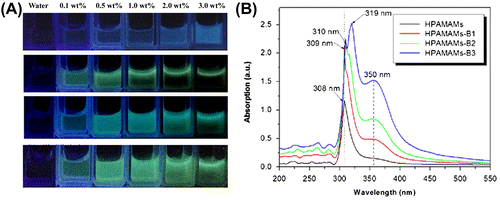
As shown in Figure (A), an emission band at 405 nm in the PL spectra of HPAMAMs is observed under light irradiation at 310 nm, due to tertiary nitrogen with lone pair electrons inside the high branching.[Citation16,Citation17] However, the emission band of polymers exhibits a blue shift to 378 nm after modification by APBA moiety. The intensity of emission band is increased compared with that of HPAMAMs at same concentration. Interestingly, the intensity of polymers modified by APBA moiety at emission band firstly increases at low concentration (0.1–0.5 wt%), reaches a maximum at 0.5 wt%, and then decreases with the increasing concentration (1.0–3.0%). It was mainly caused by intermolecular self-absorption, fluorescence quenching, and energy conversion of collision at high concentration.[Citation18–Citation22]
APBA was used in modification to endow HPAMAMs with glucose-sensitive property. Phenylboronic acid has been widely utilized for the design of chemosensors in the detection of saccharides over the past decades.[Citation22–Citation26] The covalent interactions between phenylbronic acid and the hydroxyl groups of saccharides (1,2-or 1,3-diols) lead to the formation of five- or six-membered rings and allow bronic acid to be a sensitive detector for saccharides.[Citation25–Citation28] Figure shows the fluorescent intensity curves vs. glucose concentration controlled at 0–0.8 mg/mL and the concentration of polymer kept at 0.5 wt%. The fluorescent intensity is decreased with the increasing glucose concentration in the solution. In the case of HPAMAMs, the fluorescent intensity is 35.5 a.u. when the concentration of glucose is 0 mg/mL. It is changed to 28 a.u. when the concentration of glucose is 0.8 mg/mL. The value has been dropped 7.5 a.u. with varying the concentration of glucose from 0 to 0.8 mg/mL. After modification by APBA, the strongest initial fluorescent intensity can be observed. The value of fluorescent intensity is decreased gradually with increasing the concentration of glucose. Especially in the case of HPAMAMs-B3, the fluorescent intensity is changed from 640 to 510 a.u. varying the concentration of glucose from 0 to 0.8 mg/mL. The value has been dropped to 130 a.u., which revealed that HPAMAMs modification with APBA moiety has superiority in detecting trace glucose, such as the glucose concentration in human blood. The decreasing fluorescent intensity with presence of glucose may be the photo-induced electron transfer that occurred from the unshared electron pair of the nitrogen atom to the phenyl ring through a complexation reaction of glucose with –OH groups of APBA. With the decreasing amount of –OH groups through the reaction, the intensity of emission band decreased gradually. The large and complex structure of hyperbranched polymer followed with complexation of glucose result in hindering the fluorophores emitting fluorescence.[Citation29] The fluorescent-sensitive property of HAMAMs-B dependence on the glucose concentration indicates that they are potential candidate sensitive detectors for glucose, especial at the low concentration level.
Figure 4. PL emission spectra of HPAMAMs (A), HPAMAMs-B1 (B), HPAMAMs-B2 (C), and HPAMAMs-B3 (D) in different concentration of glucose with mass fraction of 0.5 wt%.
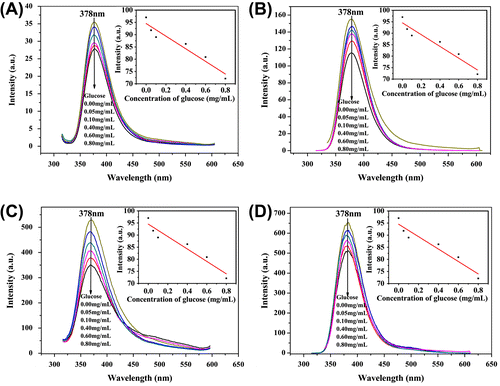
Figure showed the pH-dependent glucose sensitivity in terms of fluorescence intensity, where the concentration of polymers and glucose is controlled at 0.5 wt% and 0.05 mg/mL, respectively. It reveals that highest fluorescence intensity of solution is found at pH 7.4, which is close to the human’s physiological fluid environment. Interestingly, all the four polymers demonstrated a similar trend. The fluorescence intensity of solution decreases with either increasing or decreasing the pH of solution. Comparing with the blank samples, the fluorescence intensity is decreased 30–60% at pH 7.4 when the concentration of glucose controlled at 0.05 mg/mL. The maximum decrement in fluorescence intensity is observed in the acidic conditions. For example, the fluorescence intensity of HPAMAMs-B3 reaches a maximum decrement of 68.18% in the presence of glucose at pH 5.0, which indicates that the polymer has higher sensitivity for determining glucose in acidic environment. Although HPAMAMs and HPAMAMs-B2 have the relative higher decrement percent of fluorescence intensity, HPAMAMs-B3 has the highest optical sensitivity for the highest decrement of its absolute value in fluorescence intensity.
Figure 5. Relative fluorescence intensity of HPAMAMs, HPAMAMs-B1, HPAMAMs-B2, and HPAMAMs-B3 at different pH conditions with controlled glucose concentration at 0.05 mg/mL.
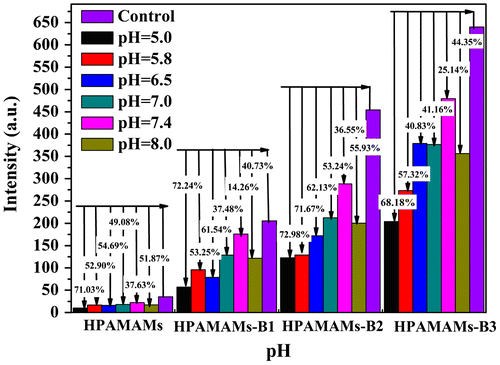
To determine the glucose sensitivity of polymers, four saccharides, namely sucrose, D-(+)-maltose, D-fructose, and glucose, have been chosen to investigate the fluorescent response properties.[Citation30,Citation31] As shown in Figure , addition of glucose to HPAMAMs-B3 solution results in an immediate decrease in intrinsic fluorescence (ex 310 nm, em 378 nm), reaching a maximum decrement of about 86.68% after 3 min. In the case of HPAMAMs-B1 and HPAMAMs-B2, the maximum decrement is around 85.66 and 76.06%, respectively. HPAMAMs exhibit the lowest decrement due to lack of the affinity functional groups to saccharide molecules. In sharp contrast, sucrose and D-(+)-maltose produces only a modest decrement of fluorescence. And D-fructose can only induce a smaller decrement. Therefore, HPAMAMs-B3 exhibits a highly selective, sensitive, and fluorescent response toward glucose.
4. Conclusion
Hyperbranched polymers with fluorescence and glucose-sensitive properties have been successfully synthesized via Michael addition polymerization of AEPZ, MA, and APBA. The resultant polymers emit chartreuse fluorescence intensity under excitation wavelength. The intensity of fluorescence is dependent on the concentration of glucose, the kinds of sugar, and the pH value of solution. The hyperbranched polymers modified by more APBA, the affinity functional groups to glucose molecules, exhibit higher glucose and fluorescent sensitivity. The as-synthesized fluorescence and glucose-sensitive hyperbranched polymers possess promising potential application in fluorescence imaging technology and glucose detector sensors.
Acknowledgments
This work was financially supported by the National Natural Science Foundation of China (51373155), ‘521 Talents Training Plan’ in Zhejiang Sci-Tech University (ZSTU). X. W. thanks the Innovative Program for Graduate Students of Zhejiang Sci-Tech University through Grant YCX12011.
References
- Shiau S-F, Juang T-Y, Chou H-W, Liang M. Synthesis and properties of new water-soluble aliphatic hyperbranched poly(amido acids) with high pH-dependent photoluminescence. Polymer. 2013;54:623–630.
- Odaci D, Gacal BN, Gacal B, Timure S, Yagci Y. Fluorescence sensing of glucose using glucose oxidase modified by PVA-pyrene prepared via “click” chemistry. Biomacromolecules. 2009;10:2928–2934.
- Wang X, Sun X, Jiang G, Wang R, Hu R, Xi X, Zhou Y, Wang S, Wang T. Synthesis of biomimetic hyperbranched zwitterionic polymers as targeting drug delivery carriers. J. Appl. Polym. Sci. 2013;128:3289–3294.
- Jiang G, Sun X, Ma Y, Cao J, Wang Y, Wang R, Wang X, Wang S. Hyperbranched polyether surface functionalized with biomimetic zwitterionic polymers as potential drug release carriers. Soft Mater. 2013;11:288–293.
- Sun X, Jiang G, Wang Y, Xu Y. Synthesis and drug-release properties of novel pH- and temperature-sensitive copolymers based on a hyperbranched polyether core. Colloid Polym. Sci. 2011;289:677–684.
- Jiang G, Sun X, Zhu Y, Wang Y. pH- and temperature sensitive copolymers based on a hyperbranched polymers as encapsulation and release carriers for guest molecules. Des. Monomers Poly. 2011;14:167–178.
- Xia W, Jiang G, Chen W. Synthesis and drug-release properties of hyperbranched polyesters grafted with biocompatible poly(ε-caprolactone). J. Appl. Polym. Sci. 2008;109:2089–2094.
- Jiang G, Xia W, Chen W. Environmental-sensitive hyperbranched polymers as drugs carriers. Des. Monomers Poly. 2008;11:105–122.
- Jiang G, Wang Y, Sun X, Shen J. Facile one-pot approach for preparing fluorescent and biodegradable hyperbranched poly(amidoamine)s. Polym. Chem. 2010;1:618–620.
- Jiang G, Wang Y, Sun X. Influence on fluorescence properties of hyperbranched poly(amidoamine)s by nano golds. J. Polym. Sci., Part B: Polym. Phys. 2010;48:2386–2391.
- Jiang G, Sun X, Wang Y, Ding M. Synthesis and fluorescence properties of hyperbranched poly(ether-imide) with highly density tertiary nitrogen. Polym. Chem. 2010;1:1644–1649.
- Farmer TG Jr, Edgar TF, Peppas NA. The future of open- and closed-loop insulin delivery systems. J. Pharm. Pharmacol. 2008;60:1–13.
- Wang Y, Jiang G, Sun X, Ding M, Hu H, Chen W. Preparation of shell cross-linked nanoparticles via miniemulsion RAFT polymerization. Polym. Chem. 2010;1:1638–1643.
- Yamaguchi S, Banno M, Ohta K, Tominaga K. Frequency dependence of vibrational energy relaxation and spectral diffusion of the N-H stretching band of pyrrole-base complexes in solution. J. Phys. Chem. A 2013;117:6323–6331.
- Xu L, Xu Y, Zhu W, Yang C, Han L, Qian X. A highly selective and sensitive fluorescence “turn-on” probe for Ag+ in aqueous solution and live cells. Dalton Trans. 2012;41:7212–7217.
- Yang W, Pan C. Synthesis and fluorescent properties of biodegradable hyperbranched poly(amido amine)s. Macromol. Rapid Commun. 2009;30:2096–2101.
- Lin Y, Gao J-W, Liu H-W, Li Y-S. Sythesis and characterization of hyperbranched poly(ether amide)s with thermoresponsive property and unexpected strong blue photoluminescence. Macromolecules. 2009;42:3237–3246.
- Doose S, Neuweiler H, Sauer M. Fluorescence quenching by photoinduced electron transfer: a reporter for conformational dynamics of macromolecules. Chem. Phys. Chem. 2009;10:1389–1398.
- Lin Y-H, Tseng W-L. Ultrasensitive sensing of Hg2+ and CH3Hg+ based on the fluorescence quenching of lysozyme type VI-stabilized gold nanoclusters. Anal. Chem. 2010;82:9194–9200.
- Dong H, Gao W, Yan F, Ji H, Ju H. Fluorescence resonance energy transfer between quantum dots and grapheme oxide for sensing biomolecules. Anal. Chem. 2010;82:5511–5517.
- Chen X, Nam S-W, Kim G-H, Song N, Jeong Y, Shin I, Kim SK, Kim J, Park S, Yoon J. A near-infrared fluorescent sensor for detection of cyanide in aqueous solution and its application for bioimaging. Chem. Commun. 2010;46:8953–8955.
- Kobayashi H, Ogawa M, Alford R, Choyke PL, Urano Y. New strategies for fluorescent probe design in medical diagnostic imaging. Chem. Rev. 2010;110:2620–2640.
- Shao M, Zhao Y. Phenylboronic acid-functionalized TTFAQ: modular synthesis and electrochemical recognition for saccharides. Tetrahedron Lett. 2010;51:2508–2511.
- Xu Z, Uddin KMA, Ye L. Boronic acid terminated thermo-responsive and fluorogenic polymer: controlling polymer architecture for chemical sensing and affinity separation. Macromolecules. 2012;45:6464–6470.
- Huh P, Kim S-C, Kim Y, Wang Y, Singh J, Kumar J, Samuelson LA, Kim BS, Jo NJ, Lee JO. Optical and electrochemical detection of saccharides with poly(aniline-co-3-aminobenzeneboronic acid) prepared from enzymatic polymerization. Biomacromolecules. 2007;8:3602–3607.
- Ancla C, Lapeyre V, Gosse I, Catargi B, Ravaine V. Designed glucose-responsive microgels with selective shrinking behavior. Langmuir. 2011;27:12693–12701.
- Yao Y, Zhao L, Yang J, Yang J. Glucose-responsive vehicles containing phenylborate ester for controlled insulin release at neutral pH. Biomacromolecules. 2012;13:1837–1844.
- Matsumoto A, Yamamoto K, Yoshida R, Kataoka K, Aoyagi T, Miyahara Y. A totally synthetic glucose responsive gel operating in physiological aqueous conditions. Chem. Commun. 2010;46:2203–2205.
- Dhanoya A, Chain BM, Moore EK. The impact of DNA topology on polyplex uptake and transfection efficiency in mammalian cells. J. Biotechnol. 2011;155:377–386.
- Mader HS, Wolfbeis OS. Boronic acid based probes for microdetermination of saccharides and glycosylated biomolecules. Microchim. Acta. 2008;162:1–34.
- Qu Z-B, Zhou X-G, Gu L, Lan R-M, Sun D-D, Yu D-J, Shi G-Y. Boronic acid functionalized graphene quantum dots as fluorescent probe for selective and sensitive glucose determination in microdialysate. Chem. Commun. 2013;49:9830–9832.