Abstract
A methacrylate monomer, cyclohexene-3-yl methyl methacrylate (CHMA) was synthesized by reaction of 3-cyclohexene-1-methanol with methacryloyl chloride in the presence of triethylamine at 0–5 °C. The homopolymer of CHMA and its copolymer with styrene (St) were prepared by free radical polymerization. The Fourier transform infrared (FT-IR), 1H NMR, and 13C NMR spectroscopic techniques were used to characterize the homopolymers and copolymers. 1H NMR spectra were used to determine the compositions of the copolymers. Linearization methods such as Kelen–Tüdös, Extended Kelen–Tüdös, and a non-linear least squares method were all used to determine the reactivity ratios of the monomers. The effect of copolymer compositions on their thermal behavior was studied by differential thermal analysis and thermogravimetric analysis methods.
1. Introduction
Plenty of studies have been published on the synthesis of functional monomers and their polymers. Functional groups give special characteristics to the polymers and can provide an approach to the subsequent modification of the polymers for specific applications.[Citation1–Citation6] Recently Degirmenci et al., synthesized mid- and end-chain cyclohexene functionalized polystyrene and poly(ε-caprolactone) and by epoxidation of the cyclohexene group. They obtained macromonomers with cyclohexene oxide functionality with potential for applications in photoinitiated cationic polymerization.[Citation7–Citation9]
The most important feature of methacrylate type monomers is their optical clarity. Due to their high light transmittance, and good mechanical and thermal resistance, they have a fairly wide area of usage. A substantial fraction of the methacrylate products are copolymers.[Citation10–Citation13] Polystyrene (PSt) is one of the world’s most widely used polymers. The copolymers of styrene with (met)acrylates are very important to industry applications such as paints, adhesives, and coatings. There are no reports on copolymerization of methacrylate monomers containing cyclohexenyl group with styrene. Thus, in this study we report the results of copolymerization of CHMA with St monomer. This study includes the determination of the monomer reactivity ratios with 1H NMR spectroscopy and effects of copolymer compositions upon thermal behaviors. Three methods including Kelen–Tüdös (KT),[Citation14] Extended Kelen–Tüdös (EKT),[Citation15] and a non-linear least squares (NLLS) methods [Citation16] were used to calculate the reactivity ratios of the comonomers.
Tg’s of the new copolymers and the effect of cyclohexene-3-yl methyl methacrylate (CHMA) content on the thermal properties of the resulting copolymers were also investigated.
2. Experimental
2.1. Materials
3-Cyclohexene-1-methanol (Aldrich) and methacryloyl chloride (Merck) were used as received. Styrene (Aldrich) was purified by washing with 5% sodium hydroxide solution for several times, followed by distilled water. Eventually, the washed Styrene was dried with anhydrous sodium sulfate (Na2SO4) and then it was filtered and distilled under reduced pressure before use. 2,2′-Azobisisobutyronitrile was recrystallized from chloroform–methanol mixture. The analytical grade commercial products, triethylamine, 1,4-dioxane, chloroform, tetrahydrofuran (THF), and ethanol (Merck) were used as received.
2.2. Synthesis of CHMA monomer
CHMA was prepared from reaction of 3-cyclohexene-1-methanol (10.00 g, 0.0856 mol) with methacryloyl chloride (8.95 g, 0.0856 mol) in the presence of triethylamine (8.66 g, 0.0856 mol) in THF at 0–5 °C range according to the usual method [Citation17] (Scheme , first row). The precipitated triethylammonium chloride was filtered out of the mixture and the solvent in the filtrate was removed through a rotary evaporator. The obtained residue was dissolved in diethylether, washed with a 5% NaOH solution twice and then washed by distilled water. Organic layers were collected and then dried over anhydrous MgSO4 for one night. Eventually, diethylether was evaporated and CHMA was achieved by distillation under reduced pressure. Yield: 50%, bp: 56 °C (2 mm Hg).
The monomer was characterized by Fourier transform infrared (FT-IR) and 1H NMR spectra:
IR (cm−1, the most characteristic bands): 3024 (C=C–H in cyclohexene ring), 2915 and 2840 (aliphatic C–H), 1716 (C=O of ester), 1637 (CH2=C),
1H NMR (δ, ppm from TMS in CDCl3): 6.14 and 5.59 (2H, CH2=), 5.72 (2H, CH=CH in cyclohexene ring) 4.09 (2H, OCH2), 2.19–1.40 (10H, CH3 protons and the methylene protons of cyclohexene ring).
13C NMR (δ, ppm from TMS in CDCl3) 167.58 (C=O of ester), 136.5 (=C), 127.08 (CH=CH in cyclohexene ring), 125.5 (CH2=), 68.96 (OCH2), 18.37 (CH3).
2.3. Homopolymerization and copolymerization
The homopolymers of CHMA and St monomer were prepared in 1,4-dioxane solution at 70 °C using AIBN (1% based on the total weight of the monomers) as the initiator. The copolymerization reactions were also performed under the same conditions that applied for the homopolymerization reactions. Required amounts of CHMA and St with 1,4-dioxane and AIBN were mixed in a polymerization tube, purged with N2 atmosphere for 15 min. Polymerization was performed at 70 °C in a thermostatic oil bath. After 2 h, the copolymer was precipitated in excess ethanol and then filtered. The precipitated polymer was purified by repeated reprecipitation from chloroform solution using ethanol and dried in a vacuum oven at 40 °C for 24 h. The conversion was determined gravimetrically. The copolymerization of CHMA with St is shown in Scheme , second row. Monomer compositions and copolymer conversions are given in Table .
Table 1. Monomer and copolymer composition data, molecular weights, and PI indices of copolymers.
2.4. Characterization
Infrared spectra were obtained with a Perkin–Elmer Spectrum One FT-IR system and recorded using universal ATR sampling accessory within the wavelengths of 4000–650 cm−1. 1H NMR spectra of copolymers were recorded in CDCl3 solution on a 400 MHz Bruker AVIII 400 using tetramethylsilane as an internal reference. Thermal stabilities and the glass transition temperatures of the polymers were investigated on a Perkin–Elmer TGA/DTA 7300 thermal analysis systems, under N2 flow with a heating rate of 20 °C/min. The molecular weights and polydispersity index (PI) were determined through the use of gel permeation chromatography (GPC). The GPC system consists an Agilent Iso Pump, a refractive index detector and both Mixed ‘D’ and Mixed ‘E’ columns. THF was used as the eluent and poly(methyl metacrylate) standards were performed for calibration.
3. Results and discussion
3.1. Characterization of the copolymer
The FT-IR spectra of CHMA–St copolymers showed characteristic bands at 3085–3055 cm−1 (aromatic C–H), 3025 cm−1 (C=C–H in cyclohexene ring), 1722 cm−1 (ester carbonyl of CHMA), 1651 cm−1 (CH=CH vibrations of the cyclohexene group), 1205 cm−1 (C–O stretching of the ester group), 700 cm−1 (aromatic C–H stretching vibration for the mono substitute benzene). 1H NMR spectrum of the homo and copolymers are given in Figure . Signals of the aromatic ring protons are between 7.15 and 6.93 ppm. The signal at 5.67 ppm belongs to CH=CH protons of the cyclohexene group. The signal at 3.80 ppm represents OCH2 proton of CHMA units. Signals determined at 1.57–2.17 ppm are due to the CH2 protons of both monomer units. At 1.57 and 0.89 ppm, there obtained signals arisen out of α-methyl protons of CHMA units.
The number average molecular weights (Mn) and PI (Mw/Mn) of the polymer samples are given in Table . PI of the copolymers range from 1.53 to 1.59, which means that the chain termination of polymers takes place predominantly by disproportionation rather than coupling.[Citation18]
3.2. Monomer reactivity ratio calculations
The most common method to determine the copolymer composition is 1H NMR technique.[Citation19,Citation20] Copolymer compositons determined by 1H NMR are given in Table .
The ratio of the rates of entry of the comonomers is given by the Mayo–Lewis copolymerization equation.[Citation21](1)
Here [A] and [B] are the molar concentrations of the two comonomers in the feed, and d[A]/d[B] is the molar ratio of the two monomer entering the copolymer. The parameters ra and rb are known as the monomer reactivity ratios. The composition of the copolymer depends not only on the feed composition but also on the monomer reactivity ratio. For this reason the knowledge of these ratios is essential for the manufacture of copolymers with desired properties.
The reactivity ratios of monomers CHMA, (A) and St, (B) were calculated by KT,[Citation14] EKT,[Citation15] and NLLS method, respectively.[Citation16] The initial CHMA and St concentrations, the molar conversions, and the final mole fraction of CHMA ([A]_fin) and St ([B]_fin) are given in Table .
Designating the initial monomer ratio by and resulting copolymer ratio by
and further arranging X and Y in the form of
and
and
and using
and
Mayo–Lewis equation is transformed into the linearized Kelen–Tüdös version,
(2)
The parameters of KT and EKT equations are presented in Tables and . The KT plot is given as Figure . The KT coefficient α = 1.48 indicates that the experimental planning was moderately good. The data lie on the straight line with very little scatter. The reactivity ratios are found as ra = 0.20 and rb = 0.76.
Table 2. KT parameters for CHMA-co-St copolymer system.
Table 3. Extended KT parameters for CHMA-co-St copolymer system.
The KT method has the advantages that the data are uniformly distributed and the results do not depend on which monomer is labeled as the monomer (A) and which one is labeled as the monomer (B). Its disadvantage is that, it uses the initial ratio of the two monomers rather than the reaction weighted cumulative average. When one monomer enters the reaction faster and is depleted more rapidly than the other one, the feed composition continuously drifts during the reaction. The KT method fails to take into account this drift. Kelen and Tüdös later proposed an extended version of their method which takes the composition drift into account.[Citation15] Since experiments are stopped above 10% conversion, the composition drift can affect the results. For this reason the data were reanalyzed using the EKT method.
EKT equation is the same as KT equation shown in Equation (Equation2(2) ). The difference between KT and EKT methods is the calculation of F and G. In EKT method, they are calculated through
,
, where
, and
,
. Here [A]0 and [B]0 are the initial molar monomer fractions.
The EKT plot is given as Figure . The reactivity ratios are found as; ra = 0.17 and rb = 0.74.
In the NLLS method used, the data is fitted to a numerical solution of the Mayo–Lewis copolymerization equation. Thus, errors emanating from linearization are eliminated. For each ra, rb pair, the copolymerization Equation (Equation1(1) ) is integrated numerically from initial monomer concentrations to final concentration of monomer B. The squares of the differences of the final calculated value of the monomer (A) from its experimental value,
(3)
are added for each experiment and the chi-square for that set of reactivity ratios are calculated.(4)
Here δA is the error bar for the relevant point. The procedure is then repeated for each pair of ra and rb in the scanning range. Thus, the produced map is used for the best parameter estimate and the confidence intervals. The resulting contour map is given as Figure and the reactivity ratios are found as; ra = 0.21 and rb = 0.89.
Figure 3. Probability contours and the best fit point (+) obtained by NLLS. Results of EKT (Δ) and KT (*) are also shown on the graph.
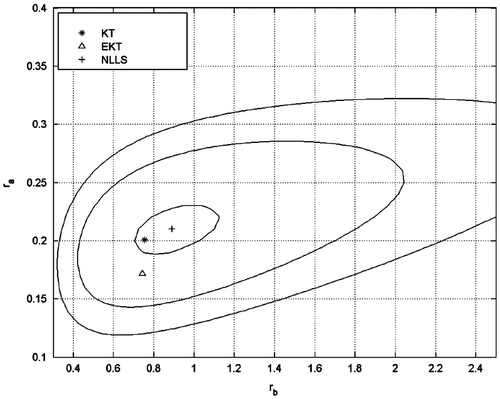
Using three independent methods to evaluate the reactivity ratios ensures that no algorithmic errors are present. In addition the differences of reactivity ratios calculated by the three methods give an estimate on the dependence of the results on the method of evaluation and the weighting of data points by the three methods. The KT, EKT, and NLLS results are close to each other.
Figure shows the numerical integration of the copolymerization equation beginning from the experimental initial conditions for each experiment using the reactivity ratios given by the three calculation techniques. The three lines starting from each initial point are the numerical integration results and the squares are the experimental data. As the reactivity ratios found by the three methods are close, the curves are similar and although results differ from experiment to experiment, overall NLLS results give the best fit.
Figure 4. Evolution of the concentrations of the comonomers for all experiments. The molar concentrations are given as percentages of the initial total molar concentration.
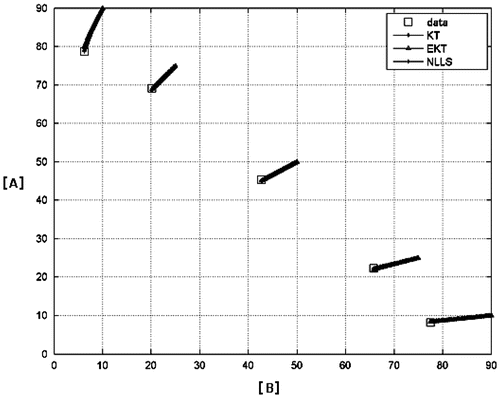
The results are summarized in Table . As can be seen from the table, the reactivity ratio of St is higher than CHMA in all case; this is probably due to the more resonance stability of radicals stemming from styrene. EKT and NLLS results for the ra/rb ratio are nearly equal (0.23) while KT results are slightly higher (0.26). This is due to the composition drift which is not taken into account in KT analysis.
Table 4. The reactivity ratios calculated by the three methods.
3.3. Glass transition temperature
The glass transition temperatures of the homopolymer and copolymers were measured by differential thermal analysis (DTA), under N2 atmosphere (Table ). The Tg of poly(CHMA) is 56 °C and Tg of PSt is 109 °C. The glass transition temperatures of copolymers measured are between 102 and 67 °C. The Tg values of all the copolymers were between those of the homopolymers, shown by the dotted line in Figure . Each copolymer observed has a single Tg, which indicates that a homogeneous phase. As known in the literature, an increase at molar mass of polymers increases the glass transition temperature. However, after molar mass reach to a specific level, glass transition temperature of polymers do not change so much. For that reason, the Tg increase in obtained CHMA–St copolymer series results from enrichment of copolymers by styrene units which have higher glass transition temperature rather than the increase at molar mass. The results clearly indicate that the Tg values of the copolymers mainly depend on the composition of comonomers and the value increases with increase of molar fractions of St in the copolymer.
Table 5. Thermal behavior and decomposition temperatures of the copolymers and homopolymers.
3.4. Thermogravimetric analysis
The thermogravimetric (TG) curves of some copolymers in comparison with the curves of the homopolymers are shown in Figure . Poly(CHMA) has presented an initiation temperature at about 250 °C and two maximum decomposition rates at 182 and 429 °C, whereas PSt has shown an initiation temperature at 328 °C and one maximum decomposition rate at 429 °C. If the temperatures corresponding to 50 wt% loss of the polymers were taken as a measure for their thermal stability, these temperatures would be 406 and 422 °C for poly(CHMA0.55-co-St) and poly(CHMA0.23-co-St), respectively. These values are valid for those of the homopolymers which are 402 and 428 °C for poly(CHMA) and PSt, respectively. Data in Figure clearly show that as CHMA units in copolymer have increased, copolymers have created a two-stage decay curve. By introducing a comonomer to the polymer chain, thermal behavior of a polymer can be affected appreciably. Therefore, it has been essential to investigate the thermal behavior of the prepared copolymers.
Figure 6. Thermal degradation curves of polymers; a: poly(CHMA), CHMA unit in copolymer; b: 0.55, c: 0.39, d: 0.23, e: 0.13, f: PSt.
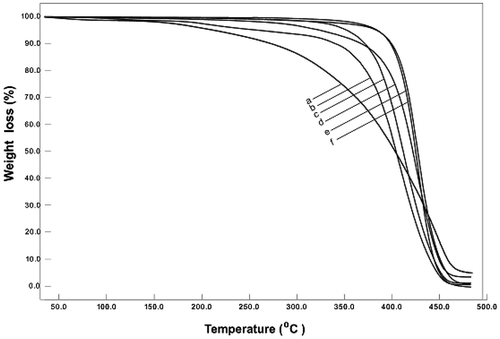
Some degradation characteristics of copolymers in comparison with the characteristics of homopolymers have been shown in Table .
4. Conclusion
Poly(CHMA) and the copolymers of CHMA with St were prepared by free radical polymerization. The reactivity ratios of CHMA and St are less than one, with rCHMA significantly less than rSt. This has mostly led to alternating copolymers and a composition drift with styrene depletion faster than the CHMA depletion.
As it has been implied by GPC data, the PI of the copolymers is between 1.53 and 1.59 and this implies a strong tendency for chain termination by disproportionation. DTA has indicated that the Tg of copolymers decreased with the increase at CHMA content of copolymers. Thermal analysis revealed that poly(CHMA) and poly(CHMA-co-St) have undergone a double step decomposition whereas, PSt has only undergone a single step decomposition. The thermal stability of the copolymers has decreased with the increase in the CHMA units of the copolymer. Using the copolymer composition and thermal analysis results together it is possible to obtain copolymers with desired thermal properties.
References
- Shirai M, Kawaue A, Okamura H, Tsunooka M. Synthesis of novel photo-cross-linkable polymers with redissolution property. Polymer. 2004;45:7519–7527.
- Shin YD, Kawaue A, Okamura H, Shirai M. Thermally crosslinkable–decrosslinkable system using diepoxy crosslinkers containing sulfonate ester moiety. React. Funct. Polym. 2004;61:293–302.
- Esquivel-Guzmán JA, Zaragoza-Galán G, Ortíz-Palacios J, Rivera E. Synthesis and characterization of novel polymers bearing fluorescein units: thermal and optical properties. Des. Monomers Polym. 2012;15:561–574.
- Chauhan NPS, Ameta R, Punjabi PB, Ameta SC. Synthesis, characterization, and antimicrobial properties of p-chloroacetophenone oxime-based furan resins. Int. J. Polym. Mater. 2012;61:1102–1114.
- Výprachtický D, Kmínek I, Pokorná V, Kaňková D, Cimrová V. New two-step synthesis of N-(2-ethylhexyl)-2,7-diiodocarbazole as a monomer for conjugated polymers. Des. Monomers Polym. 2013;16:31–37.
- Kumar SJS, Subramanian K. Synthesis, characterization, antimicrobial activity, and adhesive property of amino benzyl group substituted acrylic/methacrylic polymers and their copolymers. Int. J. Polym. Mater. 2013;62:627–634.
- Degirmenci M, Acikses A, Genli N. Well-defined cyclohexene oxide mid-chain functional polystyrene macromonomer: synthesis, characterization and photoinitiated cationic homo- and copolymerization. Macromol. Chem. Phys. 2010;211:2193–2200.
- Degirmenci M, Izgin O, Yagci Y. Synthesis and characterization of cyclohexene oxide functional poly(ε-caprolactone) macromonomers and their use in photoinitiated cationic homo- and copolymerization. J. Polym. Sci., Part A: Polym. Chem. 2004;42:3365–3372.
- Degirmenci M, Acikses A, Genli N. Cyclohexene oxide mid-chain functional macromonomer of poly(ε-caprolactone): synthesis, characterization, and photoinitiated cationic homo- and copolymerization. J. Appl. Polym. Sci. 2012;123:2567–2573.
- Bakar AA, Cheng KW, Abdul Ghani SW. Flexural and impact properties of poly(vinyl chloride) and acrylic-impact modified poly(vinyl chloride) composite filled with poly(methyl methacrylate) grafted to oil palm empty fruit bunches. Int. J. Polym. Mater. 2012;61:263–275.
- Chen J, Dai P, Liu M. Rapid responsive behaviors of the dual stimuli-sensitive poly(DEA-co-DMAEMA) hydrogel via comb-type grafted polymeriziation. Int. J. Polym. Mater. 2012;61:177–198.
- Uguzdogan E. Synthesis and characterization of hydrophilic and spherical poly(glycerol dimethacrylate-co-glycerol-1,3-diglycerolate diacrylate) microbeads. Des. Monomers Polym. 2013;16:250–262.
- Swamy BY, Prasad CV, Rao KC, Subha MCS. Preparation and characterization of poly (hydroxy ethyl methyl acrylate-co-acrylic acid) microspheres for drug delivery application. Int. J. Polym. Mater. 2013;62:700–705.
- Kelen T, Tüdös F. Analysis of the linear methods for determining the copolymerization reactivity ratios I. A new improved linear graphic method. J. Macromol. Sci. Part A: Pure Appl. Chem. 1975;9:1–27.
- Kelen T, Tüdös F, Turcsanyi B, Kennedy JP. Analysis of the linear methods for determining copolymerization reactivity ratios. IV. A comprehensive and critical reexamination of carbocationic copolymerization data. J. Polym. Sci. 1977;15:3047–3074.
- Sünbül D, Çatalgil-Giz H, Reed WF, Giz A. An error-in-variables method for determining reactivity ratios by on-line monitoring of copolymerization reactions. Macromol. Theory Simul. 2004;13:162–168.
- Subramanian K, Krishnasamy V, Nanjundan S, Rami Reddy AV. Photosensitive polymer: synthesis, characterization and properties of a polymer having pendant photocrosslinkable group. Eur. Polym. J. 2000;36:2343–2350.
- Teramachi S, Hasegawa A, Akatsuka M, Yamashita A, Takemoto N. Molecular weight distribution and correlation between chemical composition and molecular weight in a high conversion copolymer of styrene-mehylacrylate. Macromolecules. 1978;11:1206–1210.
- Vijayanand PS, Kato S, Koyama M, Satokawa S, Kojima T. Co-polymerization of 4-biphenyl methacrylate with methyl methacrylate: synthesis, characterization and determination of monomer reactivity ratios. Des. Monomers Polym. 2007;10:375–388.
- Darvishi A, Zohuriaan Mehr MJ, Marandi GB, Kabiri K, Bouhendi H, Bakhshi H. Copolymers of glycidyl methacrylate and octadecyl acrylate: synthesis, characterization, swelling properties, and reactivity ratios. Des. Monomers Polym. 2013;16:79–88.
- Mayo FR, Lewis FM. Copolymerization. I. A basis for comparing the behavior of monomers in copolymerization; the copolymerization of styrene and methyl methacrylate. J. Am. Chem. Soc. 1944;66:1594–1601.