Abstract
A novel spiroorthocarbonate with two thiol groups (SOC DITHIOL) was synthesized. This monomer was formulated with dimethacrylate monomers used in dental resins and photopolymerized with an LED dental lamp. The presence of the thiol groups in the monomer enabled it to react with the double bonds of the dimethacrylate monomers by means of the thiol–ene mechanism. Additionally, the SOC DITHIOL underwent cationic ring-opening polymerization, producing the corresponding poly(ether-carbonates). SOC DITHIOL was added to a formulation of a conventional acrylic resin, which includes a mixture of dimethacrylate monomers [glycerolate bisphenol A dimethacrylate (BIS-GMA)/urethane dimethacrylate (UDMA)/triethyleneglycol dimethacrylate (TEGDMA)] in 50/30/20 M ratio). Concentration of the prepared SOC was varied in the above dimethacrylate formulation in the range of 5–30 mol%. Kinetics of photopolymerization were determined by means of real time Fourier transform infrared spectroscopy, finding that increasing concentrations of the SOC DITHIOL in the formulations, not only did not affect the photopolymerization rate of the acrylic resins, but it helped to increase the final conversion of the methacrylate groups. It was also found that the presence of SOC DITHIOL increased the elastic modulus of the resulting cross-linked polymer. The addition of 30% of SOC DITHIOL reduced shrinkage 59% in comparison with a sample without the SOC.
1. Introduction
One of the main problems associated with dental resins is the shrinkage that occurs during the photopolymerization of dimethacrylate monomers.[Citation1] The formation of a polymer network with a denser structure than the monomer, results in volumetric contraction or shrinkage. Shrinkage is the result in the change of the van der Waals distances (3.4 Å) between the molecules of the monomer, to covalent bond lengths in the polymer (1.54 Å).[Citation2] This shrinkage provokes residual stresses within the composite, at the bonded interface between the organic and inorganic phases, leading to the debonding of the dental composite from the tooth. These stresses are undesirable because they are associated with defects, post-operative sensitivity, and secondary caries.[Citation3–5]
In the past few years, several strategies have been undertaken to develop dental resin systems with reduced shrinkage. One was the preparation of oligomers of methyl methacrylate with higher molecular weights than the BIS-GMA-based systems.[Citation6] Also, the inclusion of monomers that undergo cyclopolymerization has been investigated.[Citation7] Another approach is the use of expandable monomers. Bailey reported that bicyclic monomers, like the spiroorthocarbonates (SOCs) and the spiroorthoesters undergo expansion during polymerization.[Citation8] SOCs exhibit high-volume expansion due to the double ring-opening polymerization that leads to the formation of poly (ether-carbonates). The flexibility of these species reduces the shrinkage stress in the polymeric network. Thus, SOCs are rather interesting monomers to be used in dental resins, to decrease the shrinkage. Several kinds of SOCs monomers that are able to undergo both free radical and cationic polymerization in dental formulations have been reported.[Citation9–11]
Recently, thiol–ene resins have been investigated as an alternative to dimethacrylate resin-based composites.[Citation12] Thiol–ene resins polymerize via a step-growth mechanism, rather than the chain-growth mechanism characteristic of dimethacrylate resins, resulting in delayed gel point and enhanced control of the polymerization.[Citation13] Thiol–ene polymerization results in reduced volume shrinkage and with the delayed gelation promote significant reductions in shrinkage stress. Nonetheless, the reduction in volume shrinkage in thiol–ene systems implies a reduction in cross-link density; therefore, one of the drawbacks of thiol–ene systems is that they generally exhibit reduced mechanical properties in comparison with dimethacrylate-based systems.[Citation14] For this reason, the addition of a thiol–ene system to a conventional dimethacrylate dental resin has been attempted.[Citation15–17]
In this study, a novel SOC, the 1,4,6,9-tetraoxaspiro [4.4] nonane-2,7-diyldimethanethiol (SOC DITHIOL) was synthesized, characterized, and used in a typical dimethacrylate system Bis-GMA-TEGDMA-UDMA. The kinetics of photopolymerization, mechanical properties by dynamic mechanic analysis (DMA), and change of volume induced by the use of SOC DITHIOL were investigated.
2. Materials and methods
2.1. Materials and equipment
The following materials, all reactive grades, were purchased from Sigma-Aldrich Quimica de L. (Toluca, Mexico): 1-thioglycerol; tetraethyl orthocarbonate (TEOC); p-toluenesulfonic acid (p-TsOH); molecular sieves; sodium carbonate; BIS-GMA; UDMA; TEGDMA; bis (2,4,6-trimethyl benzoyl) phenyl phosphine (BAPO); perylene. (Decyloxyphenyl) phenyl iodonium hexafluoroantimonate (DPPI) was prepared according to the method described by Crivello and Lee.[Citation18] The nuclear magnetic resonance (NMR) spectra were obtained with a JEOL 300 MHz NMR spectrometer (Peabody, MA) at room temperature. The infrared spectra and kinetic analyses were performed on a ThermoScientific Nicolet 6700 FTIR spectrometer (Middleton, WI). The DMA was performed in a dynamic mechanical analyzer (Rheometrics Scientific).
2.2. Synthesis of SOC DITHIOL
To start, 2.5 g of molecular sieves and 30 mL of dried diethyl ether were placed in a three-necked round bottom flask fitted with a condenser, nitrogen inlet, and magnetic stirring. Then, 2 g (0.018 mol) of thioglycerol was added dropwise, followed by 0.073 g (4.2 × 10−4 mol) of p-TsOH. Finally, 2.22 g (0.011 mol) of TEOC and 0.061 g (5.7 × 10−4 mol) of anhydrous sodium carbonate were added. The system was flushed with argon to set anhydrous conditions. The reaction was kept stirred at room temperature and terminated after 20 h. The ether solution was decanted from the molecular sieves and rotoevaporated, obtaining a viscous liquid. The liquid was purified by means of column chromatography using silica gel as support, and a mixture of hexane: acetate 95:5 as eluent. The purified compound SOC DITHIOL was obtained at 43% yield.
2.3. Determination of the kinetics of photopolymerization
The real-time Fourier transform infrared spectroscopy was used to analyze the kinetics of photopolymerization in the studied formulations. The dimethacrylate systems contained a mixture of BIS-GMA/UDMA/TEGDMA in a molar ratio of 50/30/20, respectively, and the SOC DITHIOL was added in a range between 5 and 30 mol% with respect to the total amount of moles of the diacrylate monomers. DPPI and BAPO were used as photoinitiators at 1 mol%. Given that iodonium salt does not absorb visible light, it was necessary to add 2 mg of perylene as photosensitizer. Though, this compound is suspected to be carcinogenic, in this study, our aim was to ensure that the SOC polymerized quantitatively. Several photosensitizers were tested and perylene had the best performance to promote cationic ring-opening polymerization of SOC DITHIOL. For further studies, more biocompatible photosensitizers will be evaluated. The IR spectrometer was fitted with an LED Blue Phase C8 dental lamp (Ivoclar Vivadent) with a band emission spectrum from 385 to 515 nm and light intensity of 800 mW cm−2. Series scans were recorded at a rate of approximately one scan per second for 60 s. Samples were placed between two thin PP films and further irradiated with the dental lamp. The decrease in the methacrylate group conversion was monitored using the band at 1646 cm−1, and the thiol group conversion was monitored with the decrease in the peak at 2568 cm−1. In the case of the appearance of the band corresponding to the carbonyl group of the produced polyether carbonate derived from the SOC DITHIOL, the peak at 1790 cm−1 was monitored. For each system, six trials were performed. All the kinetics experiments were conducted at 25 °C. The tip of the lamp was placed at 1.8 cm from the samples at an angle of 45° with respect to the infrared beam of the spectrometer.
2.4. Determination of the viscoelastic properties of the cured polymers by DMA
The DMA technique was used to determine the viscoelastic properties of the cross-linked polymers obtained. The test specimens were prepared with dimensions of 10 × 40 × 2 mm. The samples were produced by placing the formulations in a stainless steel mold, and irradiating with the LED Blue Phase dental lamp for 60 s on each side. The frequency used in the DMA analyzer was 1 Hz and the heating speed was 5 °C min−1 in the range of 30–200 °C.
2.5. Determination of volume change
The volume change of the acrylic cross-linked polymers was determined by measuring the density of the formulations and that of the polymers. The formulation density before curing was measured by weighing a precise amount of liquid formulation and measuring accurately the volume. The same rectangular specimens prepared for DMA analysis were used for these measurements. The density of polymers was measured by means of a Sartorius balance YDK01 with density determination kit. The solid immersed in a liquid is exposed to the force of buoyancy. The value of this force is the same as that of the weight of the liquid displaced by the volume of the solid. The density measurements were performed with the Sartorius hydrostatic balance which enables to weigh the solid in air as well as in a liquid, the specific gravity of the solid is determined when the density of the liquid causing buoyancy is known, through the following formula:
where ρ = specific gravity of the solid, ρ(l) = density of the liquid, W(α) = weight of the solid in air, and W(l) = weight of the solid in liquid.
The chosen liquid was distilled water with a density values of 25 °C of d25 = 0.9976 g mL−1. The specific volume of the system before and after curing was defined as the inverse of the density and the shrinkage was calculated with the following formula:
where ΔV is the volume change, Vp is the specific volume of the solid test specimen, and Vl is the specific volume of the uncured formulation. For each sample, six trials were performed, and the presented data are the corresponding average.
3. Results and discussion
In this study, it was of interest to investigate the double benefit of having a monomer with thiol groups that can react by the thiol–ene mechanism, and at the same can reduce the shrinkage in the dental resin. Thus, with this purpose the monomer SOC DITHIOL was prepared. The synthesis of this monomer is simple and straightforward. The novel SOC was obtained by the reaction of 1-thioglycerol with TEOC, in the presence of p-TsOH in dry ether. The resulting reaction mixture, containing a mixture of five- and six-membered ring SOCs, was resolved using chromatographic column purification. The chemical structure of SOC DITHIOL was determined by FT-IR and NMR spectroscopy. Figure depicts the FTIR spectrum of the SOC DITHIOL which shows clearly the band at 2568 cm−1 that corresponds to the stretching absorption band of the thiol group. The asymmetric and symmetric stretching bands that characterize the C–O–C moiety of the SOC are present between 1024 and 1214 cm−1.
In the 1H NMR spectrum (Figure ), the peak at 1.49 ppm corresponds to the proton of the thiol groups (Hd). The peaks at 2.6 and 2.8 ppm represent the protons of the methylene adjacent to the thiol group (Ha). Signals corresponding to the methylene of the SOC ring (Hb), appear at 3.85 and 4.15 ppm. And finally, the peak of the proton of the methine (Hc) appears at 4.35 ppm.
3.1. Photopolymerization studies
Figure shows the obtained dimethacrylate conversion profile of the formulations including SOC DITHIOL compared against a control sample without the SOC. It was observed that the dimethacrylate-thiol systems, displayed higher methacrylate conversions than the dimethacrylate control resin, though with the same photopolymerization rate. The dimethacrylate control resin achieved 42% conversion in the first 10 s, and 47% after 30 s of curing. As the concentration of SOC DITHIOL was increased in the system, the conversion augmented from 50% for the 5 mol% formulation to 62% for the 30 mol% of SOC DITHIOL.
Figure 3. Methacrylate conversion vs. irradiation time for dimethacrylate control resin and the dimethacrylate-thiol system, with different concentrations of SOC DITHIOL.
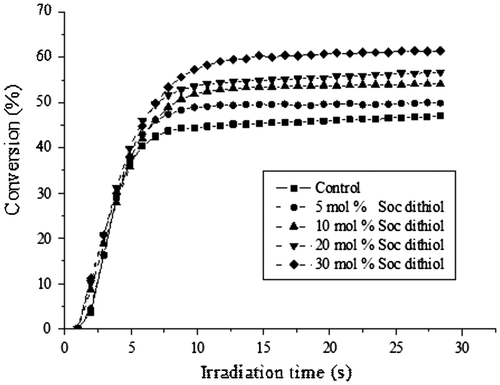
This increase in conversion can be attributed to two different effects: first, the delay in the gelation point, characteristic of the thiol–ene polymerization, results in the delay of the vitrification process of the acrylic network, leading to a higher functional group conversion; second, to the flexibilization of the polymeric acrylic matrix originated by the formation of the poly (ether-carbonates) produced in the ring-opening polymerization of the SOC. This flexibilization effect, allows higher mobility of the radical species, resulting in higher conversions of the methacrylate groups. It also needs to be considered the dilution effect of SOC DITHIOL in the formulation. The polymerization of methacrylate is strongly diffusion controlled, thereby, increasing amount of the SOC may also play a role in the final conversion of the acrylate groups. Thus, this augment in conversion due to the presence of SOC DITHIOL, is an important feature to mark, as one of the main drawbacks in dental resins is precisely the relatively low conversions achieved using systems with BIS-GMA.
In a further study, the concentration of the SOC DITHIOL was increased at 50% relative to the mixture of the dimethacrylates. This study was performed to confirm the formation of the poly (ether-carbonates) when the sample was irradiated with the LED lamp. Figure shows the conversions of the methacrylate and thiol groups, as well as the appearance of the carbonyl group of the poly (ether-carbonates) produced in the ring-opening polymerization of the SOC DITHIOL. It was observed that the methacrylate groups reacted in the same way as in the previous experiment. In the case of the thiol groups, it was found that these groups reacted slightly slower than the acrylate groups, and the conversion after one minute of irradiation was around 32%. This behavior can be ascribed to the homopolymerization of the methacrylate groups which polymerize more rapidly with themselves than with the thiol groups. The ratio of the thiol–ene reaction is represented by the conversion of the thiol. In the case of the carbonyl group of the poly (ether-carbonates), an induction period of 10 s was observed. Preliminary studies demonstrated that this induction period can be attributed to the partial quenching of perylene by the presence of the aromatic rings of BIS-GMA, hindering in this way the photolysis of DPPI. However, after this induction period, the conversion of the SOC proceeds steadily, reaching a 50% conversion after 60 s of irradiation. This delay in the conversion of the SOC DITHIOL implies that the SOC can be inserted in the cross-linked polymeric methacrylic matrix, by means of the thiol–ene reaction; however, it is not opened until 15 s of irradiation. The ring-opening polymerization of the inserted SOC moieties resulted in an increase in the cross-link density of the polymeric matrix, as it was confirmed in the DMA analyses.
3.2. DMA Analysis
After photopolymerization, complex terpolymers of polyacrylates, polythioethers, and poly (ether-carbonates) are obtained. Figure depicted a simplistic view of the produced polymer. It can be seen the poly (ether-carbonate) inserted in the blue square, the polythioethers in green squares, and the polyacrylates in the red squares. The produced cross-linked polymers were transparent with a slightly yellow hue. Figure shows the storage modulus-temperature curves of the polymers derived from the dimethacrylate-thiol systems compared against a control sample without SOC DITHIOL. The polymer produced in the photopolymerization of the dimethacrylate resin control, displayed an elastic modulus of 1700 MPa at 30 °C, but when SOC DITHIOL was added at 5 mol% to the acrylate system, the elastic modulus increases markedly to 2445 MPa at the same temperature. This behavior may be ascribed to the increase in cross-linking density that occurs in the polymer network when the inserted SOC DITHIOL reacts further by ring-opening polymerization. This effect can be observed in the increase of the modulus in the rubbery region of the curve (70–130 °C) in comparison with the control resin. When the concentration of SOC DITHIOL was increased to 10 mol%, there was another increase in the modulus (2920 MPa) due to the higher induced level of cross-linking in the polymeric network. Again, it can be seen in the rubbery region that the polymer is tightly cross-linked. Further increase of SOC DITHIOL to 20 mol% did result in a new slight increase in modulus (3057 MPA). Nevertheless, the obtained polymer had noticeably decreased cross-linking level, attributed to the flexibilization effect of the produced poly (ether-carbonates). In the case of 30 mol% of SOC DITHIOL, the high concentration of poly (ether-carbonates) originated by the ring-opening polymerization of SOC DITHIOL, resulted in a diminution in modulus of the cross-linked polymer.
Figure 5. Schematic representation of the polymer obtained by photopolymerization of the formulations of dimethacrylates monomers with SOC DITHIOL.
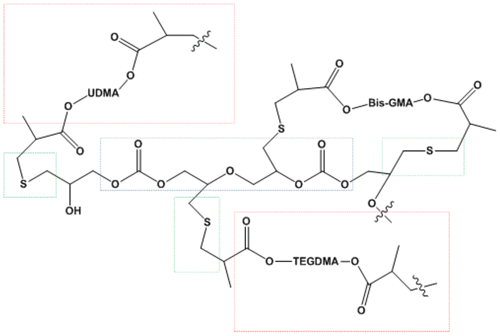
Figure 6. Results of DMA analysis of the acrylic polymers photopolymerized in the presence of SOC DITHIOL in a range between 5 and 30 mol%.
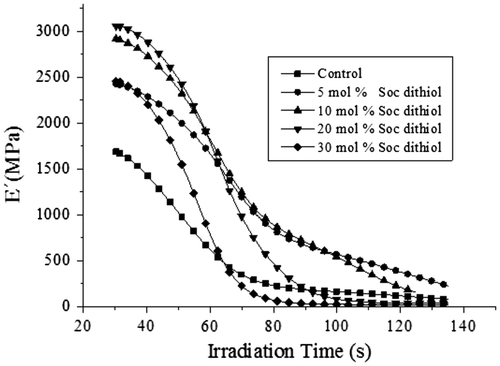
Another interesting feature of the acrylic polymers produced is that increasing concentration of SOC DITHIOL improved network homogeneity as a result of the involvement of the step-growth mechanism, which can be inferred by the narrower Tan delta peaks produced at 20 and 30 mol% of the SOC (see Figure ). Besides, the addition of SOC DITHIOL did not decrease significantly the Tg of the corresponding polymers even at the higher concentration studied of the SOC. The Tg of the control sample was observed at 80 °C, while the samples with 5 and 10 mol% displayed nearly the same Tg. In the case of the sample with 20 mol% of the SOC, the Tg was observed at 90 °C. This shift to higher temperatures is in agreement with the higher storage modulus observed in Figure and indicates higher cross-link density of the polymer at this specific concentration of SOC DITHIOL. However, increasing the concentration at 30 mol% resulted in a reduction of the value of the Tg, which was 75 °C. As it was mentioned above, this effect can be attributed to the higher concentration of poly(ether-carbonates) produced in the cationic ring-opening polymerization of SOC DITHIOL.
3.3. Determination of volume change
Table lists the results obtained of the volume change analysis of the dental composites. It can be seen that the polymer derived from the control formulation without SOC displayed a volume change of −5.37%. It was found that increasing amounts of SOC, resulted in a diminution of the shrinkage, achieving a reduction of 59% when SOC DITHIOL was added at 30 mol%. This is a significant reduction of the shrinkage considering the level of concentration of the SOC. It was not attempted to increase further the concentration of the SOC, as it would result in a decrease of the mechanical properties of the dental resin.
Table 1. Densities, specific volumes, and volume changes during photopolymerization of dental formulations studied.
4. Conclusions
A novel five-membered ring SOC with two thiol groups was successfully prepared and characterized. When this compound was evaluated in a typical dental formulation containing dimethacrylate monomers, it was found that the presence of the SOC had a beneficial effect on the dental resins as it helped to increase the conversion of the methacrylate groups as well as the mechanical properties as a result of the further cross-linking induced by the ring-opening polymerization of SOC DITHIOL. The shrinkage was reduced 59% in comparison with a sample without the SOC.
Acknowledgments
The authors thank the Mexican National Council of Science and Technology (CONACYT) for funding this project 151489. Assistance in analysis of samples by Guadalupe Mendez, Aracely Noxpanco, Fabiola Castellanos, Jose Luis Saucedo, Sergio Zertuche and Rodrigo Cedillo is gratefully acknowledged.
References
- Kim LU, Kim JW, Kim CK. Effects of molecular structure of the resins on the volumetric shrinkage and the mechanical strength of dental restorative composites. Biomacromolecules. 2006;7:2680–2687.10.1021/bm060453h
- Luck RM, Sadhir RK. Monomers that expand during polymerization. In: Sadhir RK, Luck RM, editors. Expanding monomers, synthesis and characterization, and applications. Boca Raton (MA): CRC Press; 1992. p. 21–61.
- Tantbirojn D, Versluis A, Pintado MR, DeLong R, Douglas WH. Tooth deformation patterns in molars after composite restoration. Dent. Mater. 2004;20:535–542.10.1016/j.dental.2003.05.008
- Mjor IA. Clinical diagnosis of recurrent caries. J. Am. Dent. Assoc. 2005;136:1426–1433.10.14219/jada.archive.2005.0057
- Dickens SH, Cho BH. Interpretation of bond failure through conversion and residual solvent measurements and Weibull analyses of flexural and microtensile bond strengths of bonding agents. Dent. Mater. 2005;21:354–364.10.1016/j.dental.2004.05.007
- Culbertson BM, Wan QC, Tong YH. Preparation and evaluation of visible light-cured multi-methacrylates for dental composites. J. Macromol. Sci. Pure Appl. Chem. 1997;34:2405–2421.10.1080/10601329708010056
- Butler GB. Cyclopolymerization. Encyc. Polym. Sci. Technol. 1966;4:568–599.
- Bailey WJ. Matrixes that expand on curing for high-strength composites and adhesives. Mater. Sci. Eng. A. 1990;126:271–279.10.1016/0921-5093(90)90133-N
- Stansbury JW, Bailey WJ. Evaluation of spiroorthocarbonate monomers capable of polymerization with expansion as ingredients in dental composite materials. Polym. Mater. Sci. Eng. 1988;59:402–406.
- Byerley TJ, Eick JD, Chen GP, Chappelow CC, Millich F. Synthesis and polymerization of new expanding dental monomers. Dent. Mater. 1992;8:345–350.10.1016/0109-5641(92)90016-6
- Millich F, Jeang L, Eick JD, Chappelow CC, Pinzino CS. Elements of light-cured epoxy-based dental polymer systems. J. Dent. Res. 1998;77:603–608.10.1177/00220345980770041301
- Lu H, Carioscia J, Stansbury JW, Bowman CN. Investigations of step-growth thiol-ene polymerizations for novel dental restoratives. Dent. Mater. 2005;21:1129–1136.10.1016/j.dental.2005.04.001
- Hoyle CE, Lee TY, Roper T. Chemistry of the past with promise for the future. J. Polym. Sci., Part A: Polym. Chem. 2004;42:5301–5338.10.1002/(ISSN)1099-0518
- Lee TY, Carioscia J, Smith Z, Bowman CN. Thiol-Allyl Ether-Methacrylate ternary systems. Evolution mechanism of polymerization-induced shrinkage stress and mechanical properties. Macromolecules. 2007;40:1473–1479.10.1021/ma0624954
- Cramer NB, Couch CL, Schreck KM, Carioscia J, Boulden JE, Stansbury JW. Investigation of thiol-ene and thiol-ene-methacrylate based resins as dental restorative materials. Dent. Mater. 2010;26:21–28.10.1016/j.dental.2009.08.004
- Boulden JE, Cramer NB, Schreck KM, Couch CL, Bracho-Troconis C, Stansbury JW, Bowman CN. Thiol-ene methacrylate composites as dental restorative materials. Dent. Mater. 2011;27:267–272.10.1016/j.dental.2010.11.001
- Cramer NB, Couch CL, Schreck KM, Carioscia JA, Boulden JE, Stansbury JW, Bowman CN. Investigation of the thiol-ene and thiol-enemethacrylate based resins as dental restorative materials. Dent. Mater. 2010;26:21–28.10.1016/j.dental.2009.08.004
- Crivello JV, Lee JL. Alkoxy substituted diaryliodonium salt cationic photoinitiators. J. Polym. Sci., Part A: Polym. Chem. 1989;27:3951–3968.10.1002/pola.1989.080271207