Abstract
A novel cruciform amide-containing phthalonitrile monomer was synthesized from 4-(4′-aminophenoxy) phthalonitrile and 2,2′,5,5′-biphenyl tetraacyl chloride. Linear amide-containing phthalonitrile monomer was also prepared for comparison. Various spectroscopic techniques such as IR and 1H NMR confirmed the chemical structure of the monomers. X-ray powder diffraction analysis revealed the amorphous character of the cruciform monomer. Thermal behaviors of the monomers was investigated by rheology technique. Aromatic amide moiety played an important role in promoting the cure reaction of the phthalonitrile resins. Cruciform monomer displayed a larger processing window, relative to linear phthalonitrile monomer. The polymers derived from cruciform phthalonitrile monomer showed excellent thermal properties revealed by thermogravimetric analysis and differential scanning calorimetry. The IR spectra of the polymers confirmed that triazine formation may dominate the thermal polymerization reaction.
Introduction
Phthalonitrile-based resins (PN resins) are an attractive class of high-temperature thermosetting polymers that has a number of exceptional properties, i.e., high glass transition temperature, excellent mechanical properties, outstanding thermal and thermo-oxidative stability, low water absorptivity and superior fire resistance.[Citation1–10] Addition-type polymerization reaction between the cyano groups on phthalonitrile units produces void-free, three dimensional crosslinked network polymers. The fully cured phthalonitrile resins had a variety of applications in aerospace, marine, and microelectronic industries.[Citation3,6,10,11]
In the past 30 years, various spacers such as aromatic ether,[Citation11–13] thioether,[Citation12] imide,[Citation14–16] and sulfone linkages [Citation17,18] were introduced into phthalonitrile resins at Naval Research Laboratory (NRL). In addition to their excellent properties, low melt complex viscosity (0.01–1.0 Pa s) of the PN monomers or oligomers enables facile processing by the cost-effective, non-autoclavable processing techniques such as resin transfer molding (RTM), resin infusion molding and filament winding.[Citation19,20] However, the processing mode of the phthalonitrile resins investigated at NRL, is based on bisphthalonitrile monomers/curing agents composition system referred to as A/B composition system. In this case, extremely conditions such as high temperature or vigorous stirring are required to obtain the evenly molecular dispersed mixture. This is unfavorable both for the industrial application and fundamental studies on the cure behaviors of PN resins.
The previous investigation, in our laboratory, on amino or hydroxy-containing phthalonitrile model compounds (APN or HPN) demonstrated that APN or HPN showed a self-promoted thermal polymerization even in the absence of curing additives, which are required for conventional A/B composition systems.[Citation21–23] Therefore, curing of the phthalonitrile derivatives containing amino or hydroxy groups offers a new and alternative processing mode for PN resins. The further systematic study on the thermal polymerization behaviors of the HPN indicated that the polymerization proceeds more readily with stronger acidity of the hydroxy group.[Citation23]
Aromatic polyamides (aramide) are another class of high performance polymers with excellent combination of properities. Although strong chain-chain interactions (e.g. hydrogen-bonding) of aramide result in high melting or glass transition temperature, leading to processing difficulty, readily synthetic chemistry and acidity of the amide structure still inspired us to investigate its effect on promoting the cure reaction of phthalonitrile resins. If the self-promoted polymerization can be realized for amide-containing phthalonitrile monomer, the outstanding thermal processability of the PN resins would be combined into aramide system.
Recently, the cruciform π-conjugated compounds have received much attention for their unique nature.[Citation24–26] Such a cruciform shape disrupts the crystallization tendency or prevents strong intermolecular packing of the material as well as enhances the likelihood of glass formation.[Citation27]
Therefore, as a continuation of our research, in this report, aromatic amide structure was incorporated into PN monomer along with the cruciform moiety. The thermal behaviors, including thermal processability and polymerization behavior, of this novel cruciform amide-containing PN monomer were investigated. The results showed that aromatic amide played an important role in promoting the cure reaction of the phthalonitrile monomer and cruciform moiety is effective in disrupting its crystallization tendency and further improved the thermal processability.
Experimental
Materials
4-(4′-Aminophenoxy)phthalonitrile (APN) was synthesized from 4-nitrophthalonitrile and 4-aminophenol according to Ref. [Citation28]. 2,2′,5,5′-biphenyl tetraacyl chloride was synthesized according to a previous published procedure with minor modification.[Citation29] 4,4′-Bis(3,4-dicyanophenoxy)biphenyl was purchased from N-methyl-2-pyrrolidone (NMP) and N,N-dimethylacetamide (DMAc) were purified by distillation under reduced pressure over calcium hydride. Other chemicals were used as received without further purification.
Measurements
1H NMR (400 MHz) were measured on a Bruker Avance-400 NMR spectrometer with DMSO-d6 as the solvent and tetramethylsilane as the internal standard. IR spectra were recorded with a Nicolet FTIR-460 Fourier transform infrared spectrometer by KBr pellet. Elemental analysis was performed on an Italy CARLO ERBA 1106 elemental analyzer. Thermogravimetric analysis (TGA) were carried out with a TA instrument Q500 Thermogravimetric analyzer at a heating rate of 10 °C/min in nitrogen or air. Differential scanning calorimetry (DSC) were performed on a TA instrument Q200 Differential Scanning Calorimeter at a heating rate of 20 °C/min and a nitrogen flow rate of 50 mL/min. The rheological tests were performed by TA Instruments Rheometer AR-2000 at low strain values (2.5 × 10−4) and with a frequency of 1 Hz at different temperatures in air. X-ray diffractograms were obtained in a reflection mode with Ni-filtered Cu Kα radiation on a Philips X’Pert Pro X-ray diffractometer.
Synthesis of monomers
Monomer 1
A solution of DMAc (30 mL) containing pyridine (1.000 g) and 6.00 mmol of APN (1.175 g) was introduced into a 100 mL three-necked flask, which was cooled to 0 °C in an ice-water bath. Then a solution of 2,2′,5,5′-biphenyl tetraacyl chloride (0.606 g, 1.50 mmol) in DMAc (10 mL) was added dropwise into the flask from a dropping funnel over 20 min under nitrogen. The mixture was stirred at 0 °C for 2 h. The reaction proceeded for ten more hours at room temperature. The resulting reaction mixture was poured slowly with stirring into 300 mL of water. The off-white precipitate that formed was filtered off, washed several times with deionized water, and dried in a vacuum oven. The crude product was purified by column chromatography over silica gel with ethyl acetate/hexane (3:1) as the mobile phase. Monomer 1 was obtained as a white powder. Yield: 66%. IR (KBr, cm−1): 3315 (N–H stretching), 2231 (C≡N stretching), 1655 (C=O stretching), 1088 (C–O–C stretching). 1H NMR (DMSO-d6): δ 10.08–10.05 (d, 2H), 8.21–8.14 (d, 1H), 8.11–8.04 (m, 2H), 7.96–7.89(m, 4H), 7.77–7.71 (dd, 2H), 7.65–7.63 (d, 2H), 7.39–7.29 (m, 2H), 7.22–7.20 (d, 2H), 7.11–708 (d, 2H). Elemental analysis: Calcd for C72H38N12O8: C, 72.12; H, 3.19; N, 14.02. Found: C, 71.52; H, 3.16; N, 13.91%.
Monomer 2
The synthetic procedure of monomer 2 was similar to that of monomer 1. The purification of monomer 2 was performed by recrystallization from tetrahydrofuran/ methanol (1:1 in volume) mixed solvents, which was obtained as a white crystal. Yield: 85%. Mp = 309 °C (DSC). IR (KBr, cm−1): 3453, 3370 (N–H symmetrical and asymmetrical stretching), 2228 (C≡N stretching), 1716 (C=O stretching), 1671 (C=O stretching), 1088 (C–O–C stretching). 1H NMR (DMSO-d6): δ 10.59–10.50 (s, 1H), 8.17–8.06 (m, 3H), 7.99–7.88 (d, 2H), 7.82–7.74 (s, 1H), 7.44–7.34 (dd, 1H), 7.29–7.17 (d, 2H).
Results and discussion
Synthesis of monomers
The structure and synthetic route of the cruciform amide-containing phthalonitrile monomer 1 are outlined in Schemes and , respectively. Monomer 1 was synthesized from 2,2′,5,5′-biphenyl tetraacyl chloride via solution condensation with APN using pyridine as acid acceptor. Its structure was identified by 1H NMR, FT-IR and elemental analysis. Assignments of each proton were given in Figure and the spectrum agreed well with the proposed molecular structure. It is noted that the single peaks of amide protons a and b have different chemical shifts of 10.84 and 10.58 ppm, indicating the molecular asymmetrical character of the monomer 1. Linear monomer 2 without cruciform moiety was synthesized in a similar manner for comparatively study. Its structure was confirmed by IR and 1H NMR techniques (Schemes and ).
Figure 2. X-ray powder patterns of two monomers: (a) cruciform phthalonitrile monomer 1 and (b) linear monomer 2.
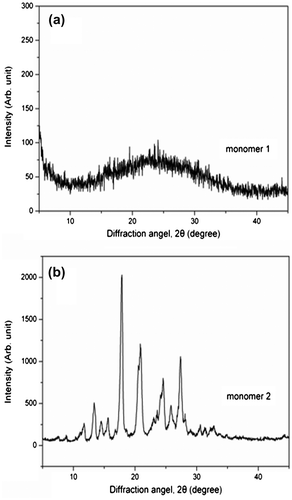
Figure 6. Structure of 4,4′-bis(3,4-dicyanophenoxy)biphenyl [Citation5].
![Figure 6. Structure of 4,4′-bis(3,4-dicyanophenoxy)biphenyl [Citation5].](/cms/asset/216c673b-a660-4827-8370-fb60e1d80856/tdmp_a_1045331_f0006_b.gif)
Thermal processability
X-ray powder diffraction (XRD) analysis showed a broad band around 25° for monomer 1, compared with a series of very sharp peaks for monomer 2, as shown in Figure . This result confirmed the amorphous nature of monomer 1, which can be attributed to the introduction of cruciform moiety. The amorphous characteristics of monomer 1 in the solid state was further verified by DSC (Figure ). A sharp endothermic peak at 309 °C was observed on the DSC curve of the monomer 2, which was corresponding to the melting temperature (Tm). In contrast, monomer 1 showed a weak endothermic step-transition around 193 °C, which was corresponding to its softening temperature.
Then, rheological measurement was conducted to elucidate the thermal processability of monomer 1. The complex viscosity of the monomer 1 was recorded as a function of temperature from 50 to 400 °C, as shown in Figure . As a comparison, the linear monomer 2 was also plotted. The viscosity of the monomer 1 started to decrease gradually on softening at around 180 °C with a minimum value of the viscosity at approximately 290 °C. In contrast, the viscosity of the monomer 2 did not exhibit changes in magnitude within the same temperature range until its melting at around 310 °C. The results further indicated the influence of introducing the cruciform moiety on the thermal processability of phthalonitrile resins. The relatively low viscosity of the cruciform monomer 1 at moderate temperatures indicated a larger processing window. This is one key feature that advantageously affects their thermal processability. On the other hand, above 375 °C for monomer 1 and 390 °C for monomer 2, both of their viscosities increased as the cure reaction progressed. The thermal polymerization behavior of the monomers will be discussed later.
Thermal polymerization behaviour
At first, the thermal polymerization behaviors of the monomers were investigated by isothermal rheological analysis at 325 °C. As shown in Figure , both of the viscosities of the monomers increased gradually. In contrast, model phthalonitrile monomer, 4,4′-bis(3,4-dicyanophenoxy)biphenyl (Figure ),[Citation5] did not show an increase in viscosity even after 2.5 h dwell at 325 °C. The result unambiguously verified the role of aromatic amide structure in promoting the polymerization rate of the phthalonitrile resins. To our knowledge, no previous study confirmed the effects of amide group on promoting phthalonitrile thermal polymerization. On the other hand, the viscosity of the monomer 1 increased at a slower rate than that of the monomer 2. This thermal polymerization feature is more advantageous for monomer 1 to be processed into thick composite sections by RTM or resin infusion methods. Therefore, thermal properties of monomer 1 and based polymers were further characterized by TGA and DSC techniques.
The TGA curves of the monomer 1 and derived polymers were shown in Figure . The thermal stability was found to be a function of the curing cycle (Table ). As the cure temperature was increased from 300 to 375 °C, an improvement in the thermal stability was observed. Further heating of monomer 1–375 °C showed a less pronounced progression in the thermal properties. Glass transitions of the polymers measured by DSC also increased from 243 °C (polymer a) to 327 °C (polymer c). The data were tabulated along with the thermal stability in Table . The disappearance of the Tg for the post-cured polymer d reveal that its cross-linking density had increased to a point where little molecular mobility was present within the polymeric backbone. The insolubility in concentrated sulfuric acid of the polymer d further indicated the occurrence of the crosslinking reaction.
Table 1. Sequences of heat treatment for monomer 1.
Table 2. Thermal stabilities of monomer 1 and polymers.
FTIR analysis
The polymerization reaction was monitored by infrared spectroscopy, as shown in Figure . Although the spectra of the polymers are broadened and reduced in intensity, due to a difficulty in grinding the sample to a small particle size, the FTIR spectra could provide more insight into the cure chemistry. Nitrile group absorption peak was at around 2232 cm−1. Clearly, after normalized to internal standard peak at 828 cm−1, nitrile absorptions gradually diminished in intensity for monomer 1 upon heat treatment in N2 (Figure ). New absorption peaks at 1358 cm−1 were observed which indicated the formation of triazine ring derived from the polymerization of cyano groups (Figure ),[Citation30] and grow in intensity with increasing extent of polymerization (Figure ). Furthermore, the nitrile absorptions shifted to lower wavenumbers, similar to a previous report when cyano groups were converted to triazine ring.[Citation30] Burchill also reported that one-third of cyano groups remain unreacted for PN resin even after post-cure, if polymer chain extension was mainly originated from triazine formation.[Citation31] In Figure , around 37% (0.32/0.86 × 100%) cyano groups was unreacted for polymer d, which agreed well with burchill’s report.
Conclusions
A novel cruciform amide-containing phthalonitrile monomer can be readily synthesized from 4-(4′-aminophenoxy) phthalonitrile (APN) and 2,2′,5,5′-biphenyl tetraacyl chloride (monomer 1). Amorphous character of the monomer 1 was confirmed by combination of XRD and DSC measurements. Rheological test unambiguously verified the role of aromatic amide structure in promoting the thermal polymerization reaction of PN resins, and revealed that cruciform monomer 1 show larger processing window relative to linear monomer 2. The polymers derived from monomer 1 showed excellent thermal properties with T5% in the range of 429–514 °C and Tg 243–327 °C. Formation of triazine ring was confirmed for the polymers by IR technique. Further studies on the relationship between the cure procedure and other properties such as mechanical and electric properties are in progress.
Disclosure statement
No potential conflict of interest was reported by the authors.
Additional information
Funding
References
- Keller TM, Price TR. Amine-cured bisphenol-linked phthalonitrile resins. J. Macromol. Sci. Chem. A. 1982;18:931–937.10.1080/00222338208077208
- Warzel ML, Keller TM. Tensile and fracture properties of a phthalonitrile polymer. Polymer. 1993;34:663–666.10.1016/0032-3861(93)90570-Z
- Sastri SB, Armistead JP, Keller TM. Phthalonitrile-carbon fiber composites. Polym. Compos. 1996;17:816–822.10.1002/(ISSN)1548-0569
- Zhang JH, Liu XH, Wen HR, et al. Investigation of the properties of phthalocyanine resin containing bismaleimide groups. Polym. Int. 1997;42:363–366.10.1002/(ISSN)1097-0126
- Sastri SB, Keller TM. Phthalonitrile cure reaction with aromatic diamines. J. Polym. Sci. Part A: Polym. Chem. 1998;36:1885–1890.10.1002/(ISSN)1099-0518
- Dominguez DD, Jones HN, Keller TM. The effect of curing additive on the mechanical properties of phthalonitrile-carbon fiber composites. Polym. Compos. 2004;25:554–561.10.1002/(ISSN)1548-0569
- Laskoski M, Dominguez DD, Keller TM. Synthesis and properties of a bisphenol A based phthalonitrile resin. J. Polym. Sci. Part A Polym. Chem. 2005;43:4136–4143.10.1002/(ISSN)1099-0518
- Keller TM, Dominguez DD. High temperature resorcinol-based phthalonitrile polymer. Polymer. 2005;46:4614–4618.10.1016/j.polymer.2005.03.068
- Dominguez DD, Keller TM. Properties of phthalonitrile monomer blends and thermosetting phthalonitrile copolymers. Polymer. 2007;48:91–97.10.1016/j.polymer.2006.11.003
- Keller TM, Roland CM. High temperature adhesive. United States patent US 5,242,755. 1993.
- Keller TM. High-performance, electrically conductive polymers. Chemtech. 1988;18:635–639.
- Keller TM. Fluorinated high temperature phthalonitrile resin. Polym. Commun. 1987;28:337–339.
- Keller TM. Phthalonitrile resin as a carbon source. Polym. Commun. 1990;31:229–231.
- Keller TM. Synthesis of oligomeric imide-containing phthalonitriles. Polym. Commun. 1991;32:2–4.
- Keller TM. Imide-containing phthalonitrile resin. Polymer. 1993;34:952–955.10.1016/0032-3861(93)90213-T
- Zeng K, Zou Y, Yang G. Synthesis and properties of polyimides derived from a new phthalonitrile-containing diamine with high polyaddition reactivity. Des. Monomers Polym. 2014;17:186–193.10.1080/15685551.2013.840500
- Keller TM. Synthesis of polysulphone phthalonitriles. Polym. Commun. 1984;25:42–44.
- Keller TM. Polymerization of polysulphone phthalonitriles. Polym. Commun. 1985;26:48–50.
- Yang XL, Lei YJ, Zhong JC, et al. Preparation and thermal properties of novel phthalonitrile oligomer containing biphenyl ethernitrile/bisphthalonitrile blends. J. Appl. Polym. Sci. 2011;119:882–887.10.1002/app.v119:2
- Yang XL, Zhang JD, Lei YJ, et al. Effect of different aromatic amines on the crosslinking behavior and thermal properties of phthalonitrile oligomer containing biphenyl ethernitrile. J. Appl. Polym. Sci. 2011;121:2331–2337.10.1002/app.v121.4
- Zeng K, Zhou K, Tang WR, et al. Synthesis and curing of a novel amino-containing phthalonitrile derivative. Chin. Chem. Lett. 2007;18:523–526.10.1016/j.cclet.2007.03.035
- Zhou H, Wang Y, Liu T, et al. Synthesis, characterization and self-promoted cure behaviors of a new phthalonitrile derivative 4-(4-(3,5-diaminobenzoyl) phenoxy) phthalonitrile. Polym. Bull. 2009;62:581–591.10.1007/s00289-009-0041-3
- Zeng K, Zhou K, Zhou SH, et al. Studies on self-promoted cure behaviors of hydroxy-containing phthalonitrile model compounds. Eur. Polym. J. 2009;45:1328–1335.10.1016/j.eurpolymj.2008.12.036
- He F, Cheng G, Zhang HQ, et al. Synthesis, characteristics and luminescence properties of oligo(phenylenevinylene) dimers with a biphenyl linkage center. Chem. Commun. 2003;2206–2207.10.1039/b306694k
- Wilson JN, Josowicz M, Wang YQ, et al. Cruciform π-systems: hybrid phenylene-ethynylene/phenylene-vinylene oligomers. Chem. Commun. 2003;24: 2962–2963.10.1039/b312156a
- Saragi TPI, Spehr T, Siebert A, et al. Spiro compounds for organic optoelectronics. Chem. Rev. 2007;107:1011–1065.10.1021/cr0501341
- Shirota Y. Photo- and electroactive amorphous molecular materials – molecular design, syntheses, reactions, properties, and applications. J. Mater. Chem. 2005;15:75–93.10.1039/b413819h
- Buzin PV, Yablokova MY, Kuznetsov AA, et al. New AB polyetherimides obtained by direct polycyclocondensation of aminophenoxy phthalic acids. High Performance Polym. 2004;16:505–514.10.1177/0954008304039991
- Li L, Zhang SB, Zhang XS, et al. Polyamide thin film composite membranes prepared from isomeric biphenyl tetraacyl chloride and m-phenylenediamine. J. Membr. Sci. 2008;315:20–27.
- Griffith JR, Snow AW, Marullo NP. Syntheses and characterization of heteroatom-bridged metal-free phthalocyanine network polymers and model compounds. Macromolecules. 1984;17:1614–1624.
- Burchill PJ. On the formation and properties of a high-temperature resin from a bisphthalonitrile. J. Polym. Sci. Part A Polym. Chem. 1994;32:1–8.10.1002/pola.1994.080320101