Abstract
A series of nanogels (NGs) were developed from N-vinylcaprolactam and hydroxyethyl methacrylate through free radical emulsion polymerization using methylene bis acrylamide as cross-linker. Curcumin, an anticancer agent was successfully loaded into these NGs via equilibrium in situ method. These NGs were characterized by Fourier transform spectroscopy (FTIR), differential scanning calorimetry (DSC), transmission electron microscopy (TEM), and dynamic light-scattering experimental (DLS) techniques. The formation of co-polymeric NGs was confirmed by FTIR analysis. DSC results reveal that the drug was molecularly dispersed in the NG networks. TEM results indicate the formation of NGs, in a spherical shape, with the size of 150 nm. The DLS results also support the formation and size of NGs. An in vitro release study indicates that the NGs may be potentially useful for targeted drug delivery applications.
Introduction
The limitations of the current therapy provide a compelling rationale for the development of alternative modalities for targeted delivery of therapeutics in the treatment of solid tumors.[Citation1–3] An exciting potential solution in cancer treatment is to encapsulate the drug in a biocompatible material, that can be injected into the blood stream with the intention of delivering the drug to the tumor site in response to an external thermal source such as a radio frequency generator, a source that provides radio waves to heat and kill cancer cells.[Citation2,3]
Recently, intense research efforts were made in order to prepare drug carriers, based on soluble polymers, nanoparticles, nano-capsules, cells, lipoproteins, liposomes, and micelles. Among them, hydrogel nanoparticles (nanogels (NGs)) combine the advantages of their small dimensions (i.e. improved bioavailability, large surface area for multivalent bio-conjugation and targeted delivery of drug). This is in addition to their stimuli-responsive behavior, that offers numerous opportunities for the construction of a new generation of carrier systems for drug or gene molecules, due to their unique properties. Such NGs, especially those showing multiple stimuli-responsive behavior that causes a change in temperature and/or pH of body tissue are of particular interest due to many different types of diseases (e.g. cancer, diabetes, pathological, etc.).[Citation4–8]
Such NGs are usually prepared by emulsion copolymerization with or without the addition of surfactants, such as sodium dodecyl sulfate (SDS) using NIPAAm, AAm, and AAc as monomers or co-monomers and N,N’-methylene bisacrylamide (BIS) as cross-linker. Depending on the monomers used, the resulting microgels may be sensitive to external stimuli, such as temperature, pH, and ionic strength of the aqueous solution.[Citation9,10] The stimuli-sensitive gels based on copolymers, have been intensively studied and significant progress has been achieved in recent years. Temperature is one of the most widely used stimuli for stimuli-sensitive gels, because it is easy to control and has practical advantages in both in vitro and in vivo studies. Temperature-sensitive gels undergo a volume phase transition or a sol–gel phase transition at critical temperatures, namely: lower critical solution temperature (LCST) or upper critical solution temperature (UCST). LCST polymers exhibit a hydrophilic-to-hydrophobic transition with increasing temperature, whereas the UCST systems undergo the opposite transition. In contrast to the UCST systems, the LCST systems have received more attention for drug delivery, since mixing of the UCST systems and drugs needs to be performed at relatively high temperature, which may be harmful to some unstable drugs or bio-molecules, hence leading to inconveniences in drug formulation.
Typical LCST polymers include poly(N-isopropylacrylamide) (NIPAAm),[Citation11,12] poly(N,N-diethylacrylamide),[Citation13] poly(methylvinylether),[Citation14,15] etc., are the most studied temperature-sensitive polymers. In comparison to their temperature-sensitive counterparts poly(N-vinylcaprolactam) (PNVC) is favored but less investigated.[Citation16–18] PNVC has many important properties, e.g. solubility in water and in other solvents,[Citation18,19] high stability to hydrolysis,[Citation20] and LCST varying between 32 and 33 °C similar to NIPAAm and also less cytotoxic than (NIPAAm).[Citation21–23]
Comonomer 2-hydoxyethyl methacrylate (HEMA) has been widely used as biomaterial because of its good biocompatibility nature. The poly(HEMA) gels are used in intraocular lenses and soft contact lenses, surface modification of biomaterials and in some other applications. Incorporation of hydrophilic acrylic monomers into poly(HEMA) significantly changes HEMA-swelling properties and promotes a gain in pH sensitivity as reported by Rao et al. [Citation24]. Copolymerization of HEMA and NIPAAm yields a thermo-sensitive hydrogel matrix with hydroxyl functionality.[Citation25,26] The swelling behavior of the NIPAAm/HEMA co-polymeric gels was related to the gel structure, the surrounding temperature, and the swelling media. The application of these co-polymeric gels in enzymatic activity and release of low molecular substances was reported by Lee and Hung [Citation27].
Curcumin has been used in traditional medicine in different countries, such as in India and China for many centuries.[Citation28,29] Curcumin, a naturally occurring polyphenolic phytoconstituent, chemically a yellow polyophenol, is a diferuloylmethane extracted from the rhizomes of turmeric (Curcuma longa). It possesses anticancer, antioxidant, anti-inflammatory, hyperlipidemic, antibacterial, wound healing, and hepatoprotective activities.[Citation30–32] The therapeutic efficacy of curcumin is limited, due to its poor oral bioavailability.[Citation33] Curcumin has been associated with the regression of pre-malignant lesions of the bladder, soft palate gastrointestinal tract, cervix and the skin, with treatment responses in established malignancy.[Citation34] The only factor that limits the use of free curcumin for cancer therapy is its poor solubility in water, which in turn limits its systemic bioavailability, when administered orally. Polymeric nano-particle-based drug delivery system is being investigated increasingly as this delivery route is known to overcome many obstacle associated with the delivery of free drugs.
These observations encouraged the author for further investigations using these materials. Thus, in the current study, the author formulated curcumin-encapsulated cross-linked three dimensional NG networks with Poly(N-Vinylcaprolactam-co-hydroxyl ethyl methacrylate) (poly(NVCL-co-HEMA)). The NGs exhibit temperature-responsive characteristics. The NGs were then characterized for encapsulation efficiency, particle size, and surface morphology. Furthermore, the curcumin NGs formulations were evaluated for their in vitro release profiles. The results indicated that temperature-sensitive NGs would be potentially useful for targeted cancer drug delivery applications and are presented in this work.
Materials
N-vinylcaprolactam and hydroxyl ethyl methacrylate were purchased from Aldrich Chemicals, Milwaukee, WI USA. N,N’-methylene bisacrylamide, sodium lauryl sulfate, potassium persulfate, and calcium chloride were purchased from S.d. fine chemicals, Mumbai, India. Curcumin was purchased from Himedia, India.
Experimental methods
Preparation of NGs
Poly(NVCL-co-HEMA) NGs were prepared by batch emulsion polymerization in a round bottom flask, equipped with a reflux condenser and a nitrogen gas inlet. SDS (1 g) and potassium persulfate (100 mg) were dissolved in 100mL of water and different amounts of NVCL, HEMA, and methylene bisacrylamide (BIS) (2, 4, 6 wt%) were added to the SDS solution. The mixture was bubbled with nitrogen gas for 45min, heated to 70 °C, and stirred vigorously at a speed of 800 rpm for 20 h. At the end of the reaction, the mixture was taken out, cooled to room temperature, and transferred to a beaker with 5 wt% CaCl2 solution added drop-wise in order to break the emulsion. Particles were isolated by centrifuging the product at 14,000 rpm speed for 15 min and washed with double-distilled water (DD water) and then particles were collected. The resulting particles were immersed in DD water for 2 days in order to remove any unreacted monomers and cross-linker and were again centrifuged at the above conditions and dried under vacuum at 40 °C for 24 h.
Characterization techniques
Differential scanning calorimetry (DSC) studies
Differential scanning calorimetry (DSC) curves of the placebo NVCL/HEMA NGs, plain drug, and drug-loaded NGs were recorded on a TA instrument (Model: STA, Q600 USA). 10–12 mg of the samples for DSC studies were heated from 30 to 400 °C at a heating rate of 10 °C/min in nitrogen atmosphere for DSC studies (flow rate 100 mL/min).
Transmission electron microscopy (TEM) studies
Approximately 10 mg/mL NGs dispersion were prepared in distilled water with constant stirring. One drop of dispersion was placed on a 400 mesh copper grid and allowed to air-dry for 5min. Excess solution was removed by delicately touching the edges of the grid with filter paper, followed by another 5–10min of drying. Transmission electron microscopy (TEM) images of the particles were taken on a Tecnai T-12 microscope operated at 80kV (FEI, Cambridge, England).
Dynamic light-scattering (DLS) studies
Mean diameter and size distribution of the NGs were determined by the dynamic light scattering (DLS) method using a Brookhaven BI-9000 AT instrument (Brookhaven Instruments Corporation, USA). The mean diameter was measured for each sample six times in order to calculate standard deviation.
Estimation of drug loading and encapsulation efficiency
Specific amount of drug-loaded dry NGs was vigorously stirred in a beaker containing 10mL of 7.4 pH buffer solution in order to extract the drug from the NGs. The solution was then filtered and analyzed by a UV spectrophotometer at λmax of 400 nm. These results of % CUR loading and encapsulation efficiency were calculated using Equations (1) and (2), respectively. The results are complied in Tables and , respectively.(1)
(2)
Table 1. Amount of reagents used in the different formulations for NGs preparation.
Table 2. % Encapsulation efficiencies and release kinetics parameters of different formulations of NGs.
In vitro release studies
In vitro release studies were carried out by dissolution experiments using the tablet dissolution tester (Lab India, DS 8000, Mumbai, India) equipped with eight baskets. Dissolution rates were measured at 37 °C at a rotation speed of 100rpm. Drug release from the NGs was studied in the gastric fluid (pH 1.2) and intestinal atmospheric-like fluids (7.4 pH phosphate buffer) with tween 80 (0.5% w/w). At regular intervals of time, aliquot samples were withdrawn and analyzed using a UV spectrophotometer at a fixed λmax value of 400 nm. Measurements were taken three times for the estimation of standard deviation. After every sample collection, the same amount of fresh medium, at the same temperature, was added to the release medium to and maintain the sink condition. All measurements were carried out in triplicate and values were plotted with standard deviation errors.
Results and discussion
The different formulations of poly(NVCL-co-HEMA) NGs,are prepared by varying NVCL, HEMA, drug, and cross-linker and the details of these are shown in Table .
Fourier transform infrared (FTIR) studies
The strategy of preparing the NGs involves a simple one-pot emulsion polymerization method. In this method the monomer and cross-linker units are polymerized in the core of sodium dodecylsulphate (SDS) via a free radical initiation technique. NGs formation was confirmed by Fourier transforms infrared (FTIR) techniques. Figure shows the FTIR spectra of poly(NVCL-co-HEMA) NGs. In the FTIR spectrum of poly(NVCL-co-HEMA) which was cross-linked with methylene bis acrylamide (MBA), a broad band in the region 3500–3400 cm−1 corresponds to the –NH stretching of (MBA) which overlapped with the –OH stretching vibrations. The sharp stretching vibration bands of –CH and –CH2 groups are observed at 2919 and 2850 cm−1, respectively. The peaks observed at 1472 and 1383 cm−1, correspond to the –CH2 and –OH bending vibrations. The –C–N band gives an absorption band at 1246 and 1214 cm−1. The characteristic peaks in the region 1200–1050 cm−1 corresponds to the –C–N, –C–C–, and –C–O groups. The bands at 1620 cm−1 corresponds to the CO group of the caprolactam ring, and the amide group, which overlapped each other. In addition, a new peak appeared at 1720 cm−1 which corresponds to the ester group of the HEMA units. All the functional groups are present in the co-polymeric NGs, but peaks that belong to double bonds, completely disappeared. These FTIR spectra clearly indicate the formation of co-polymeric NGs.
Loading of drug into NGs
Recently, our group also synthesized NGs which were composed of poly(N-vinylcaprolactam-co-acrylamidoglycolicacid).[Citation35] In the present study, the NGs prepared exhibited high stability with dual responsive characteristics (pH and temperature sensitive). In this study, NGs also exhibited higher stability because of the hydrogen bonding interactions emanating from the presence of –OH groups of (HEMA) and caprolactam ring (NVCL). Generally as curcumin is a water insoluble drug, it is not possible to incorporate it into NGs in order to study the % equilibrium swelling. Hence ,in order to carry out the swelling studies while incorporating curcumin, we have polymerized these by adding acetone via in situ process. Figure shows the dispersion of pure NGs in water (a) and drug-loaded NGs in water (b), which revealed that, this drug was successfully loaded into the NGs. The % encapsulation efficiencies were also calculated based on the equation proposed by Ritger and Peppas [Citation38] and are shown in Table . On careful observation of the % EE values, it is obvious that the values depend on the structure of NGs during the formation. Furthermore, NGs were characterized by DSC analysis for the confirmation of drug-loading into the NGs and also to study the thermal transitions of the samples under inert atmosphere. DSC thermo grams of drug-loaded NGs (a) placebo NGs (b), pristine drug, and (c) (Curcumin), are displayed in Figure . Figure (c) shows a sharp peak at 176 °C for the pure drug at its melting temperature. Figure (b) shows a peak at 100 °C in the case of placebo NGs, which is due to the endothermic transition as a result of loss of moisture from the NGs. The other peak, appearing at 275 °C, is due to the melting temperature of the NGs. The drug-loaded NGs also show (Figure (a)), a decrease in melting temperature which is due to the hydrogen bonding interactions between drug and NGs and a further analysis indicated that no peaks are observed at 176 °C in the case Figure (a), highlighting the peak at which the melting point of the drug disappeared in the drug-loaded NGs because of the change in its physical state from its original crystalline state to an amorphous state.
Morphology and particle size of NGs
Figure (A) and (B) are TEM images of NGs that were prepared using 70:30 of NVCL:HEMA with 10% of curcumin and 2% MBA as cross-linker. Figure (A) shows the TEM images of the NGs; Figure (B) shows the individual NGs. The NGs were spherical and well dispersed, with an average size of approximately 150 nm. Particle size has a crucial impact on the in vivo fate of a colloidal drug delivery system,[Citation36,37] therefore the effective control over the carrier size is of great importance. In the present case, the copolymer composition, % of drug loading, and % of cross-linker are affecting the size of NGs. The results of the mean particle size with standard errors are presented in Table . The size distribution curve for a typical formulation containing 70:30 of NVCL:HEMA with 10% of curcumin and 2% MBA is displayed in Figure . It is found that the size distribution is narrow (polydispersity is 0.194) and the mean diameter of the NGs is 180 nm.The particle size of different formulations was calculated and included in Table .
In vitro release studies
Effect of HEMA content
In order to understand the nature of drug release from curcumin-loaded NGs of cross-linked poly(NVCL-co-HEMA), in vitro release experiments were carried out at different temperatures (25 and 37 °C). The effect of HEMA content in the formulations: NVCL–HEMA1, NVCL–HEMA2, and NVCL–HEMA3 on the release rates is presented in Figure at 25 °C and Figure at 37 °C, respectively.
Figure 8. % Cumulative release of curcumin through NGs containing different amounts of drug. NVCL–HEMA2 (15%), NVCL–HEMA4 (20%), and NVCL–HEMA5 (30%) at 37 °C.
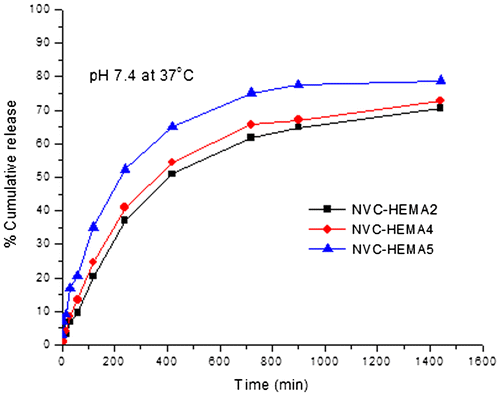
Figure 9. % Cumulative release of curcumin through NGs containing different amounts of cross-linker. NVCL–HEMA2 (1%), NVCL–HEMA6 (2%), and NVCL–HEMA7 (3%) at 37 °C.
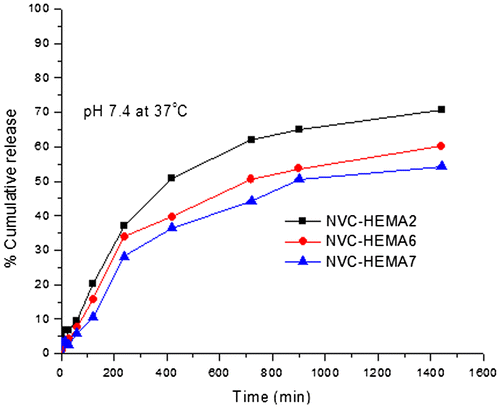
From Figures and , the % cumulative release is highest in the case of high amount of HEMA (NVCL–HEMA3) than NVCL–HEMA1 and NVCL–HEMA2, resulting from the high content of HEMA (i.e. hydrophilicity nature increases) in the polymer matrix. At 25 °C (below LCST), the release of curcumin from gels increased, but at a higher temperature (above LCST) of 37 °C, the % cumulative release decreased. This is due to the quick hydration of NGs that are fully swollen at low temperature but at high temperature, the polymer network structure was in a collapsed state and thus, exhibited lesser tendency to water uptake or buffer solution, leading to a decrease in the drug diffusion rate from the matrix. This result confirms the fact that the NGs prepared are thermoresposive. The schematic representations of curcumin release from NGs at different temperatures are shown in Scheme .
Effect of drug
Figure shows the release profiles of curcumin-loaded NGs at different amounts of drug loadings and at 37 °C. Release data showed that formulations containing the highest amount of drug (30% NVCL–HEMA5) displayed the fastest and highest release rates than formulations containing a small amount of drug (15% NVCL–HEMA2, 20% NVCL–HEMA4). A prolonged release was observed for the formulation (15% NVCL–HEMA2) containing a lower amount of drug. In other words, with decreasing amounts of drug in the matrix, there is the availability of more free void spaces through which a fewer number of drug molecules will be transported.
Effect of cross-linker
The % cumulative release data vs. time plots for varying amounts of cross-linker (BIS) i.e. 1, 2, and 3wt% (NVCL–HEMA2, NVCL–HEMA6, and NVCL–HEMA7) at a fixed amount of the drug (15%) are displayed in Figure . The % cumulative release is quite fast and large at the lower amount of BIS (i.e. 1% NVCL–HEMA2), whereas the release is slower at higher amounts of BIS (i.e. 2% NVCL–HEMA6, 3% NVCL–HEMA7). The cumulative release is slower when a higher amount of BIS was used probably because at higher concentrations of BIS, polymeric chains become rigid due to the contraction of micro voids, thus decreasing the % cumulative release of Curcumin through the polymeric matrices. As expected, the release becomes slower at higher amounts of BIS, but becomes faster at lower amounts of BIS.
Kinetics of invitro release studies
Drug release kinetics was analyzed by plotting the cumulative release data vs. time and by fitting these data to the exponential equation of the type.[Citation38](3)
where Mt/M∞ represents the fractional drug released at time t, k is a constant characteristic of the drug-polymer system and n is an empirical parameter characterizing the release mechanism. Using the least square procedure, the values of n and k for all the nine formulations are calculated and these values are given in Table . If n=0.5, the drug diffuses and releases from the polymer matrix following a Fickian diffusion. For n>0.5, anomalous or non-Fickian type drug diffusion occurs. If n=1, a completely non-Fickian or Case II release kinetics is operative. The intermediary n values (Table ) ranging between 0.5 and 1.0 are attributed to the anomalous type transport.[Citation39] From Table , it is also noticed that the values of n have shown a dependence on the extent of crosslinking, % drug loading and HEMA content of the matrix. The values of n for NGs of all formulations are in the range 0.36–0.49, which further indicates Fickian diffusion transport mechanism.
Conclusions
Temperature-responsive curcumin-loaded poly(NVC-co-HEMA) NGs were prepared by emulsion polymerization. FTIR data of the NGs suggest the formation of co-polymeric NGs. DSC data indicated the dispersion of Curcumin particles in the NGs at a molecular level. The results of TEM and particle size determination studies show that the NGs are of uniform size and spherical in shape. The in vitro release profiles suggested the potentiality of NGs with temperature-responsive nature for CR of Curcumin. Due to the presence of large hydroxyl groups in the NGs networks, they may be useful for temperature-responsive targeted cancer drug delivery applications.
Acknowledgments
One of the authors (K. Sudhakar) is highly grateful to UGC, New Delhi for providing grants of the UGC BSR Meritorious fellowship and Tshwane University of Technology, Pretoria, South Africa, for their support.
Disclosure statement
No potential conflict of interest was reported by the authors.
Additional information
Funding
References
- Meyer DE, Shin BC, Kong GA, Dewhirst MW, Chilkoti A. Drug targeting using thermally responsive polymers and local hyperthermia. J. Control. Release. 2001;74:213–224.10.1016/S0168-3659(01)00319-4
- Li J, Wang B, Liu P. Possibility of active targeting to tumor by local hyperthermia with temperature-sensitive nanoparticles. Med. Hypotheses. 2008;71:249–251.10.1016/j.mehy.2008.03.023
- Sanoj Rejinold N, Muthunarayanan M, Divyarani VV, et al. Curcumin-loaded biocompatible thermoresponsive polymeric nanoparticles for cancer drug delivery. J. Colloid Interface Sci. 2011;360:39–5110.1016/j.jcis.2011.04.006
- Soppimath KS, Liu LH, Seow WY, et al. Multifunctional core/shell nanoparticles self-assembled from pH-Induced thermosensitive polymers for targeted intracellular anticancer drug delivery. Adv. Funct. Mater. 2007;17:355–362.10.1002/(ISSN)1616-3028
- Liu Y, Cao X, Luo M, Le Z, Xu W. Self-assembled micellar nanoparticles of a novel star copolymer for thermo and pH dual-responsive drug release. J. Colloid Interface Sci. 2009;329:244–252.10.1016/j.jcis.2008.10.007
- Ulanski P, Rosiak JM, Nalwa HS. Encyclopaedia of nanoscience and nanotechnology. Vol. 8. Stevenson Ranch, CA: American Scientific; 2004. p. 845.
- Lee ES, Na K, Bae YH. Polymeric micelle for tumor pH and folate-mediated targeting. J. Control. Release. 2003;91:103–113.10.1016/S0168-3659(03)00239-6
- Demirel GB, von Klitzing R. A new multiresponsive drug delivery system using smart nanogels. ChemPhysChem. 2013;14:2833–2840.10.1002/cphc.v14.12
- Prabaharan M, Grailer JJ, Steeber DA, Gong S. Stimuli-responsive chitosan-graft-poly(N-vinylcaprolactam) as a promising material for controlled hydrophobic drug delivery. Macromol. Biosci. 2008;8:843–851.10.1002/mabi.v8:9
- Hatefi A, Amsden B. Biodegradable injectable in situ forming drug delivery systems. J. Control. Release. 2002;80:9–28.10.1016/S0168-3659(02)00008-1
- Krishna Rao KSV, Madhusudana Rao K, Nagendra Kumar PV, Chung II-D. Novel chitosan based pH sensitive micro-networks for the controlled release of 5-fluorouracil. Iran. Polym. J 2010;19:265–276.
- Schild HG. Poly(N-isopropylacrylamide): experiment, theory and application. Prog. Polym. Sci. 1992;17:163–249.10.1016/0079-6700(92)90023-R
- Shibayama M, Norisuye T, Nomura S. Cross-link density dependence of spatial inhomogeneities and dynamic fluctuations of poly(N-isopropylacrylamide) gels. Macromolecules. 1999;29:8746–8750.
- Idziak I, Avoce D, Lessard D, Gravel D, Zhu XX. Thermosensitivity of aqueous solutions of poly(N, N-diethylacrylamide). Macromolecules. 1999;32:1260–1263.10.1021/ma981171f
- Horne RA, Almeida JP, Day AF, Yu NT. Macromolecule hydration and the effect of solutes on the cloud point of aqueous solutions of polyvinyl methyl ether: a possible model for protein denaturation and temperature control in homeothermic animals. J. Colloid Interface Sci. 1971;35:77–84.10.1016/0021-9797(71)90187-1
- Mikheeva LM, Grinberg NV, Mashkevich AY, et al. Microcalorimetric study of thermal cooperative transitions in poly(N-vinylcaprolactam) hydrogels. Macromolecules. 1997;30:2693–2699.10.1021/ma9615112
- Van Durme K, Verbrugghe S, Du Prez FE, Van Mele B. Influence of poly(ethylene oxide) grafts on kinetics of LCST behavior in aqueous poly(N-vinylcaprolactam) solutions and networks studied by modulated temperature DSC. Macromolecules. 2004;37:1054–1061.10.1021/ma035319t
- Schmaljohann D. Thermo- and pH-responsive polymers in drug delivery. Adv. Drug Deliv. Rev. 2006;58:1655–1670.10.1016/j.addr.2006.09.020
- Makhaeva EE, Thanh LTM, Starodoubtsev SG, Khokhlov AR. Thermoshrinking behavior of poly(vinylcaprolactam) gels in aqueous solution. Macromol. Chem. Phys. 1996;197:1973–1982.10.1002/macp.1996.021970616
- Vihola H, Laukkanen A, Hirvonen J, Tenhu H. Binding and release of drugs into and from thermosensitive poly(N-vinyl caprolactam) nanoparticles. Eur. J. Pharmaceut. Sci. 2002;16:69–74.10.1016/S0928-0987(02)00076-3
- Makhaeva EE, Tenhu H, Khokhlov AR. Conformational changes of poly(vinylcaprolactam) macromolecules and their complexes with ionic surfactants in aqueous solution. Macromolecules. 1998;31:6112–6118.10.1021/ma980158s
- Cooperstein MA, Canavan HE. Assessment of cytotoxicity of (N-isopropyl acrylamide) and poly(N-isopropyl acrylamide)-coated surfaces. Biointerphases. 2013;8:19–32.10.1186/1559-4106-8-19
- Çavuş S, Çakal E. Synthesis and characterization of novel poly(N-vinylcaprolactam-co-itaconic acid) gels and analysis of pH and temperature sensitivity. Ind. Eng. Chem. Res. 2012;51:1218–1226.
- Rao KM, Mallikarjuna B, Krishna Rao KSV, et al. Synthesis and characterization of pH sensitive poly (hydroxy ethyl methacrylate-co-acrylamidoglycolic acid) based hydrogels for controlled release studies of 5-fluorouracil. Int. J. Polym. Mater. Polym. Biomater. 2013;62:565–571.
- Çiçek H, Tuncel A. Preparation and characterization of thermoresponsive isopropylacrylamide-hydroxyethylmethacrylate copolymer gels. J. Polym. Sci. A. 1998;36:527–541.
- Lee W-F, Shieh C-H pH-thermoreversible hydrogels. I. Synthesis and swelling behaviors of the (N-isopropylacrylamide-co-acrylamide-co-2-hydroxyethyl methacrylate) copolymeric hydrogels. J. Appl. Polym. Sci. 1999;71:221–231.
- Lee W-F, Huang Y-L. Thermoreversible hydrogels XIV. Synthesis and swelling behavior of the (n-isopropylacrylamide-co-2-hydroxyethyl methacrylate) copolymeric hydrogels. J. Appl. Polym. Sci. 2000;77:1769–1781.10.1002/(ISSN)1097-4628
- Shishodia S, Sethi G, Aggarwal BB. Curcumin: getting back to the roots. Ann. New York Acad. Sci. 2005;1056:206–217.10.1196/annals.1352.010
- Kunwar A, Barik A, Pandey R, Priyadarsini KI. Transport of liposomal and albumin loaded curcumin to living cells: an absorption and fluorescence spectroscopic study. Biochim. Biophys. Acta. 2006;1760:1513–1520.10.1016/j.bbagen.2006.06.012
- Kumar A, Dogra S, Prakash A. Protective effect of curcumin (Curcuma longa), against aluminium toxicity: possible behavioral and biochemical alterations in rats. Behav. Brain Res. 2009;205:384–390.10.1016/j.bbr.2009.07.012
- Kumar A, Dogra S, Prakash A. Protective effect of curcumin (Curcuma longa), against aluminium toxicity: possible behavioral and biochemical alterations in rats. Behav. Brain Res. 2009;205:384–390.10.1016/j.bbr.2009.07.012
- Bharat A, Anushree K, Bharti CA. Anticancer potential of curcumin: preclinical and clinical studies. Anticancer Res. 2003;23:363–398.
- Kurien BT, Scofield RH. Increasing aqueous solubility of curcumin for improving bioavailability. Trends Pharmacol. Sci. 2009;30:334–335.10.1016/j.tips.2009.04.005
- Mukerjee A, Vishwanatha JK. Formulation, characterization and evaluation of curcumin-loaded PLGA nanospheres for cancer therapy. Anticancer Res. 2009;29:3867–3875.
- Madhusudana Rao K, Mallikarjuna B, Krishna Rao KSV, Siraj S, Chowdoji Rao K, Subha MCS. Novel thermo/pH sensitive nanogels composed from poly(N-vinylcaprolactam) for controlled release of an anticancer drug. Colloids Surf. B. 2013;102:891–897.
- Tapia C, Costa E, Moris M, Sapag-Hagar J, Valenzuela F, Basualto C. Study of the influence of the pH media dissolution, degree of polymerization, and degree of swelling of the polymers on the mechanism of release of diltiazem from matrices based on mixtures of chitosan/alginate. Drug Develop. Ind. Pharm. 2002;28:217–224.10.1081/DDC-120002455
- Nel AE, Mädler L, Velegol D, et al. Understanding biophysicochemical interactions at the nano-bio interface. Nat Mater. 2009;8:543–557.10.1038/nmat2442
- Ritger PL, Peppas NA A simple equation for description of solute release II. Fickian and anomalous release from swellable devices. J. Control. Release. 1987;5:37–42.10.1016/0168-3659(87)90035-6
- Siepmann J, Peppas NA. Modeling of drug release from delivery systems based on hydroxypropyl methylcellulose (HPMC). Adv. Drug Deliv. Rev. 2001;48:139–157.10.1016/S0169-409X(01)00112-0