Abstract
A series of π-conjugated polymers bearing conjugated side chain were prepared by Migita–Kosugi–Stille coupling polycondensation reaction. The polymers consisting of phenylene–thiophene (CCPTO), phenylene–isothianaphthene (CCPIO), and phenylene-3,4-ethylenedioxythiophene (CCPEO) conjugated backbones in the main chains showed optical absorption peaks in the same region, while fluorescence peak of CCPTO was at larger wavelengths compared with those of CCPIO and CCPEO. The polymers showed optical rotary dispersion, indicating the optically active substituents induced one-handed helicity to the polymers.
1. Introduction
Conjugated polymers exhibit electrical conductivity upon doping of electron acceptor or donor, visible–near-infrared light absorption, and optical emission. Today, conjugated polymers have been developed for applications of optical and electrical devices. Organic field-effect transistors,[Citation1] photovoltaic cells,[Citation2,3] and light-emitting diodes [Citation4] have attracted much attention. Various designs of the chemical structures of conjugated polymers have been studied to achieve improved performances. Since a conjugated structure consists of rigid structure, alkoxyl groups [Citation5], or ethylene glycol [Citation6,7] introduced into the main chains have been reported in order to obtain solubility and processability. Design of conjugated backbones is considered to draw optical and electrical functionalities. The backbones such as polyacetylene,[Citation8,9] polythiophene,[Citation10] and polyphenylenevinylene [Citation11] can be used. As an approach to obtain expanded conjugated structures, introduction of conjugated side chains to the conjugated main chain has been considered.[Citation12–14] Extension of effective conjugation length and enhancement of charge carrier mobility are expected by this approach.[Citation15,16] The cross-conjugated structures can form two-dimensional structures.
We designed a series of conjugated polymers bearing conjugated side chains connected directly to conjugated structures of the main chains. These polymers have phenylenevinylene structures in the side chains and phenylene–thiophene structures in the main chain. Thiophene, isothianaphthene (benzo[c]thiophene), and 3,4-ethylenedioxythiophene (EDOT) rings were employed as parts of backbones. Isothianaphthene is known as one of the most desirable thiophene derivatives for low bandgap conjugated polymers.[Citation17,18] Poly(EDOT) has also been attracted because of high conductivities and moderate bandgaps of polymers.[Citation19] In this paper, we employed Migita–Kosugi–Stille coupling polycondensation for preparing conjugated polymers. Optical properties of these polymers were examined to consider relationship between the conjugated side chain and the conjugated main chains.
2. Experimental
2.1. Materials and instruments
Tetrahydrofuran (THF) and methanol were purchased from Nacalai Tesque (Japan). Chloroform-d was purchased from Sigma–Aldrich Japan (Japan). Toluene was purchased from Wako Chemical (Japan). Tetrakis(triphenylphosphine)palladium was purchased from Tokyo Chemical (Japan). Chloroform was purchased from Kanto Chemical (Japan). Molecular weights of the polymers were estimated by gel permeation chromatography (GPC) with a MIXED-D HPLC column (Polymer Laboratories), a PU-980 HPLC pump (Jasco) and an MD-915 multiwavelength detector (JASCO, Japan). THF was used as the solvent for the GPC measurement. Polystyrene standard was employed to determine the molecular weight calibration curve. 1H Nuclear magnetic resonance (NMR) spectra of the polymers were taken on an ECS 400 spectrometer (JEOL) with chloroform-d at room temperature. Fourier transform infrared (FTIR) absorption spectra were obtained using an FT/IR-300 spectrometer (Jasco) with KBr pellet method. Ultraviolet–visible (UV–vis) absorption spectra were recorded on a V-630 UV–vis optical absorption spectrometer (JASCO, Japan). Photoluminescence spectra were recorded on an F-4500 fluorescence spectrophotometer (Hitachi). Optical rotatory dispersion (ORD) spectra were obtained with a J-720 spectrometer (JASCO, Japan). Differential scanning calorimetry (DSC) and thermogravimetric analysis (TGA) were carried out under argon atmosphere with an EXSTAR7000 (Seiko Instruments Inc., Japan). Heating and cooling rates were 10 °C/min for these measurements. Polarizing optical microscopic observation was carried out using an ECLIPS LV 100 high-resolution polarizing microscope (Nikon) with an LU Plan Fluor lens and a CFIUW lens (Nikon).
2.2. Synthesis
Chemical structures and reaction schemes for these polymers are described in Scheme . These polymers were abbreviated as CCPTO, CCPIO, and CCPEO, respectively.
2.2.1. General procedure for the Migita–Kosugi–Stille coupling polycondensation
A Schlenk flask with a magnetic stirrer was evacuated and filled with argon gas. A cross-conjugated monomer 1,4-dibromo-2,5-bis[(E)-2-[4-(3,7-dimethyloctyloxy)phenyl]vinyl]benzene (CCMO, 1 eq), a stannyl derivative (1 eq), and toluene were added into the flask and stirred for 30 min at 50 °C. The toluene was freshly distilled and purified. Tetrakis(triphenylphosphine)palladium (Pd(PPh3)4, 0.04 eq) was then added to the solution and refluxed for 2 days at 90 °C. After cooling, the mixture was poured into large volume of methanol to precipitate the polymer. The precipitate was recovered by suction filtration and dissolved into small amount of toluene. The precipitation was repeated three times. The final precipitate was isolated by suction filtration, and dried in vacuo to afford the desired polymer in the solid form.
2.2.2. Synthesis of CCPTO
2,5-Bis(trimethylstannyl)thiophene and CCMO were prepared by the previously reported methods.[Citation20,21] Migita–Kosugi–Stille coupling polycondensation was carried out according to the general procedure between 2,5-bis(trimethylstannyl)thiophene (111 mg, 0.271 mmol) and CCMO (202 mg, 0.268 mmol) in the presence of Pd(PPh3)4 (12.4 mg, 0.011 mmol) in toluene (15 mL). CCPTO was obtained as a yellow solid (173 mg, 94.9%). 1H NMR (400 MHz, δ from TMS (ppm), CDCl3): δ 0.86 (d, 12H, –CH(CH3)2, J = 6.8 Hz), 0.93 (d, 6H, –CH(CH3) –CH2–, J = 6.4 Hz), 1.13–1.85 (br, m, 20H, –O–CH2–CH2–CH(CH3) – (CH2)3–CH(CH3)2), 3.96 (brs, 4H, –O–CH2–C9H19), 6.86 (d, 4H, 3,5H (side chain benzene), J = 7.2 Hz), 6.90 (m, 2H, –Ar–CH=CH–Ar–), 7.11 (d, 2H, –Ar–CH=CH–Ar–, J = 14.8 Hz), 7.25 (m, 2H, 3,4H (thiophene)), 7,42 (d, 4H, 2,6H (side chain benzene), J = 8.4 Hz), 7.91 (brs, 2H, 3,6H (main chain benzene)). IR (KBr, cm−1): 3032, 2953, 2926, 2867, 2839, 1604, 1574, 1510, 1471, 1383, 1302, 1287, 1250, 1174, 1111, 1069, 1020, 961, 853, 843, 817.
2.2.3. Synthesis of CCPIO
1,3-Bis(trimethylstannyl)isothianaphthene and CCMO were prepared by the previously reported methods.[Citation20,21] Migita–Kosugi–Stille coupling polycondensation was carried out according to the general procedure between 1,3-bis(trimethylstannyl)isothianaphthene (125 mg, 0.271 mmol) and CCMO (200 mg, 0.265 mmol) in the presence of Pd(PPh3)4 (12.7 mg, 0.010 mmol) in toluene (15 mL). CCPIO was obtained as a light green solid (170 mg, 88.2%). 1H NMR (400 MHz, δ from TMS (ppm), CDCl3): δ 0.88 (d, 12H, –CH(CH3)2, J = 6.4 Hz), 0.96 (d, 6H, –CH(CH3)–CH2–, J = 6.4 Hz), 1.14–1.86 (br, m, 20H, –O–CH2–CH2–CH(CH3)–(CH2)3–CH(CH3)2), 4.03 (brs, 4H, –O–CH2–C9H19), 6.76 (m, 2H, 3H or 4H (isothianaphthene)), 6.91 (d, 4H, 3,5H (side chain benzene), J = 8.4 Hz), 7.01 (d, 2H, –Ar–CH=CH–Ar–, J = 16.0 Hz), 7.11 (m, 2H, 3H or 4H (isothianaphthene)), 7.22 (d, 2H, –Ar–CH=CH–Ar–, J = 16.0 Hz), 7,49 (d, 4H, 2,6H (side chain benzene), J = 8.4 Hz), 7.84 (m, 2H, 3,6H (main chain benzene)). IR (KBr, cm−1): 3032, 2953, 2926, 2867, 2843, 1696, 1604, 1574, 1510, 1470, 1383, 1302, 1289, 1250, 1174, 1111, 1051, 1018, 961, 851, 817, 753.
2.2.4. Synthesis of CCPEO
2,5-Bis(tributylstannyl)-3,4-ethylenedioxythiophene and CCMO were prepared by the previously reported methods.[Citation20,21] Migita–Kosugi–Stille coupling polycondensation was carried out according to the general procedure between 2,5-bis(tributylstannyl)-3,4-ethylenedioxythiophene (49.6 mg, 0.069 mmol) and CCMO (48.9 mg, 0.065 mmol) in the presence of Pd(PPh3)4 (3.4 mg, 0.0029 mmol) in toluene (1.3 mL). CCPEO was obtained as a dark red solid (27.5 mg, 57.5%). 1H NMR (400 MHz, δ from TMS (ppm), CDCl3): δ 0.87 (d, 12H, –CH(CH3)2, J = 6.4 Hz), 0.96 (m, 6H, –CH(CH3)–CH2–), 1.11–1.88 (br, m, 20H, –O–CH2–CH2–CH(CH3)–(CH2)3–CH(CH3)2), 3.95 (m, 2H, –O–CH2–CH2–O–), 4.01 (m, 4H, –O–CH2–C9H19), 4.24 (m, 2H, –O–CH2–CH2–O–), 6.85 (m, 4H, 3,5H (side chain benzene)), 6.89 (m, 2H, –Ar–CH=CH–Ar–), 7.12 (d, 2H, –Ar–CH=CH–Ar–, J = 18.0 Hz), 7,43 (m, 4H, 2,6H (side chain benzene)), 7.92 (m, 2H, 3,6H (main chain benzene)). IR (KBr, cm−1): 3032, 2953, 2926, 2867, 2838, 1604, 1574, 1510, 1473, 1456, 1383, 1362, 1302, 1288, 1250, 1174, 1111, 1020, 961, 853, 818.
3. Result and discussion
The polymerization results were summarized in Table . CCPTO shows number average molecular weight (Mn, 7900 g/mol) and weight average molecular weight (Mw, 16,000 g/mol). Weight-average degree of polymerization (DPw = Mw/mru, mru: mole of repeat unit) was to be 23.6, indicating the polymerization was achieved. Dispersity (Mw/Mn) of CCPTO was 2.02. In general, ideal linear polymers prepared by step polymerization should be polymers with Mw/Mn = 2, in accordance with the Flory–Schulz distribution.[Citation22,23] On the other hand, CCPIO and CCPEO showed relatively low Mn and Mw. Isothianaphthene and EDOT derivatives are known as high reactive compounds because of their electron-rich conjugated systems.[Citation24,25] However, steric hindrance between adjacent monomer unit results in low-molecular weights.
Table 1. Polymerization results.
Proton NMR spectra of the resultant polymers are shown in Figure . CDCl3 was employed as a solvent for the measurement. All the major peaks were well identified on the basis of the proton NMR spectra of CCMO.[Citation21] The spectra of CCPTO and CCPEO show broad peaks because of large molecular weights. On the other hand, relatively low-molecular weight of CCPIO results in the sharp peaks in the spectrum. Common peaks around 0.8–1.9 ppm derive from protons of alkyl chains of the terminal group. A recognizable peak at 4.0 ppm is attributed to protons connected to the alpha carbon of the alkoxyl group. Only the spectrum of CCPEO has two extra bands near the region. These peaks are due to ethylenedioxy group of the EDOT unit. Protons of π-conjugated moieties of these polymers exhibit peaks around 6.5–8.0 ppm. There are peaks around 7.25 ppm only in the spectrum of CCPTO, indicating existence of thiophene unit (3,4H). As for CCPIO, two characteristic bands at 6.8 and 7.1 ppm can be observed. According to a previous report by Okuda et al., these peaks are derived from protons of 3,4 positions of isothianaphthene unit.[Citation26] Molecular structures of these polymers were thus confirmed.
FTIR spectra of these polymers and CCMO as a monomer are shown in Figure . These polymers show absorption bands at 3032, 2953, 2926, 2867, 1604, 1574, 1510, 1470, 1250, and 1174 cm−1. Absorption bands at 3032, 1604, and 1510 cm−1 are originated from the C–H stretching vibration derived from benzene groups, the C=C asymmetric stretching vibration, the C=C symmetric stretching vibration, respectively. Asymmetric and symmetric stretching vibrations of the thiophene ring are observed at 1574 and 1470 cm−1. The bands at 2953, 2926, and 2867 cm−1 are assigned to –CH3 stretching vibration and two –CH2– stretching vibration mode of alkyl chain substituted to the terminal of the conjugated side chains. Alkoxyl C–O–C asymmetric and symmetric stretching vibrations also appear at 1250 and 1174 cm−1, respectively. As for the monomer CCMO, stretching vibrations due to the benzene and the alkoxyl group are also observed. In the FTIR spectrum of CCPTO, C–H in-plane thiophene deformation and C–H out-of-plane thiophene deformation are observed at 1069, and 843 cm−1, respectively. Absorption bands at 1362 and 1090 cm−1 are observed only in the spectrum of CCPEO. These are assigned to C=C vibration of quinonoidal thiophene and C–O–C stretching vibration in the EDOT structure.[Citation27,28] The quinonoidal vibration indicates CCPEO is slightly oxidized because of generation of bipolarons consisting of quinonoid structure. An absorption band at 753 cm−1 appears only in the case of CCPIO. This is derived from C–H out-of-plane vibration of the benzene ring of the isothianaphthene group.[Citation29] Although CCPIO and CCPEO have low-molecular weights, these characteristic absorptions confirm the structure of desired polymers.
UV–vis absorption and emission spectra of the monomer and the polymers are shown in Figure . Peak tops of the absorption and the emission are listed in Table . All of the polymers appeared absorption bands in the same wavelength region. These absorption bands are shifted to longer wavelengths compared with that of CCMO. This is because effective conjugated length was developed by the formation of thiophene unit sequence after polymerization. These absorption bands are due to π–π* transition of the conjugated backbones of the polymers. The absorption edge of CCPTO locates longer absorption wavelengths than those of the others. This can be due to the fact that effective conjugation length of CCPTO is developed compared with those of CCPIO and CCPEO. Emission spectra of the polymers show the same tendency. CCPTO displays a broad emission in longer wavelengths, while CCPIO and CCPEO show emission peaks at the same wavelengths of CCMO. Since CCMO has only π-conjugation structure in the side chain, the emissions of CCPIO and CCPEO mainly come from their conjugated side chain moieties. This indicates geometry relaxations of CCPIO and CCPEO during excitation and emission are similar to that of CCMO. In contrast, highly developed conjugated main chain structure allows CCPTO showing large Stokes shift. This may be due to effective geometry relaxation.
Figure 3. UV–vis absorption (solid) and emission (dashed) spectra of CCPTO, CCPIO, CCPEO, and CCMO in CHCl3 solution.
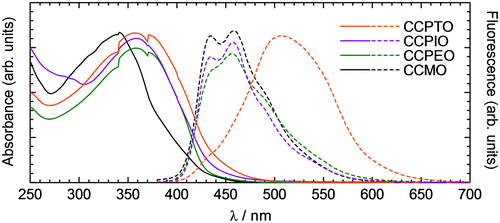
Figure 5. Results of thermal analyses for the polymers. (a) DSC curves of the polymers at 2nd heating and cooling. (b) A TGA curve of CCPEO from 200 to 580 °C. (c) TGA curves of the polymers from 270 to 420 °C.
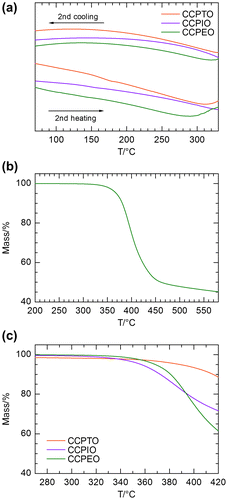
Figure 6. POM images of CCPTO. Images were taken in the same area under (a) crossed nicol and (b) open nicol at 240 °C. (c) Schlieren-like texture around an air bubble under crossed nicol at 200 °C. (d) Droplets texture during transition between isotropic phase and liquid crystalline phase under crossed nicol at 210 °C.
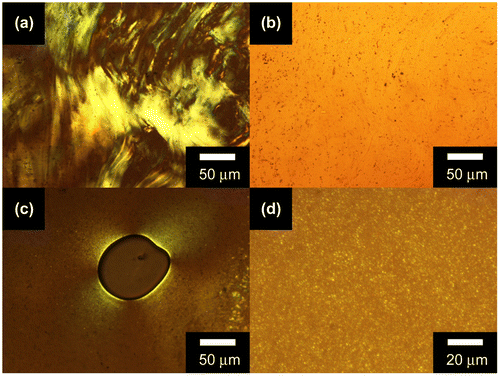
Figure 7. POM images of a CCPTO bulk sample under crossed nicol condition at (a) 220 °C, (b) 240 °C, (c) 260 °C, (d) 280 °C, and (e) 300 °C during the heating.
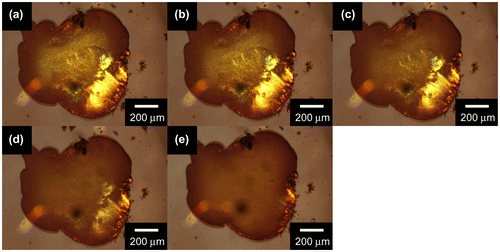
Table 2. Optical data of the polymers in CHCl3 solution.
These compounds have a pair of chiral centers at the terminal alkoxyl group as shown in Scheme , showing optical activities. Figure displays results of ORD spectroscopy for the polymers and CCMO. All of the compounds exhibited positive optical rotation bands at around 420 nm. Although absorption bands due to the chiral alkyl chains appear in the shorter wavelengths (<200 nm), these polymers appear optical rotations at the same wavelengths as the polymers. As for CCPTO, optical rotation maximum is observed at 420 nm. This indicates that the main chain forms chiral structure such as helical structure. Introduction of one-handed helical conformation can be performed from the chiral centers at the terminal alkyl chains.
Thermal analyses of the polymers were carried out. The results are shown in Figure . Among the polymers, only CCPTO exhibits a small peak in a DSC heating curve at around 175 °C as shown in Figure (a). This indicates phase change to a mesophase occurred at this temperature. At around 300 °C, broad peaks are observed in the curves of CCPTO and CCPEO. These are due to phase transition to isotropic phases. TGA was also carried out. Figure (b) is the TGA curve of CCPEO to 580 °C. The mass started to decrease after 300 °C. As for CCPTO and CCPIO, samples were foamed during the analysis at around 430 °C. Gasses may be evolved by the heating at this temperature. The masses could be no longer monitored over the temperature range because of shaking by the foaming. Thermal stability of these polymers estimated by the TGA curves is shown in Figure (c). Temperatures at which 5% degradation of CCPTO, CCPIO, and CCPEO are 389, 357 and 369 °C, respectively. This result suggests larger molecular weight enhances their thermal stabilities.
Phase transition of these polymers was confirmed by DSC measurement Furthermore, the phase transition of CCPTO was visually confirmed using polarizing optical microscopy (POM). The polymer samples were sandwiched by cover glasses (15 mm in diameter), and the samples were placed on a hot stage during the POM observation. CCPIO and CCPEO showed direct phase transitions from a solid to a liquid (isotropic) phase during the heating processes. On the other hand, CCPTO exhibited a mesophase between solid and liquid phase at wide temperature range of 180–260 °C. Figure shows POM images of CCPTO taken during the mesophase. In this phase, CCPTO was viscous fluid, which is easily moved by mechanical press onto the glass cell. As shown in Figure (a), retardation color and light–dark contrast were observed under crossed nicol condition. These forms were not observed under open nicol (Figure (b)). The retardation color indicates that CCPTO shows birefringence in this phase. Besides, the contrast and the color of CCPTO were changed by external mechanical pressure. These results indicate that this mesophase is attributed to nematic liquid crystal (LC) phase of CCPTO. This polymer has conjugated side chains connected to the conjugated main chain. These rigid structures behave as a super mesogen core.[Citation30,31] Such super mesogen cores can be observed for liquid crystal polymers having rigid structures both in the side chains and the main chains.[Citation30] Therefore, CCPTO can also be regarded as a liquid crystal polymer forming super mesogen. Generally, rigid main chains are required to exhibit liquid crystallinity for polymers in order to form a structural anisotropy. Therefore, only CCPTO having long main chain exhibits liquid crystallinity. Figure (c) shows birefringence around an air bubble. The birefringence comes from inflection of directors of LC around the bubble boundary. Moreover, the small droplets were observed during transition between isotropic phase and the liquid crystalline phase as shown in Figure (d). These small dots can be nematic droplets. These observation results demonstrated that CCPTO shows thermotropic nematic LC.
The phase change of CCPTO occurs gradually during the heating and the cooling. Figure shows POM images for a bulk sample of CCPTO during the second heating. This heating was conducted after the first heating from room temperature to 300 °C, and the subsequent cooling to 50 °C. The light–dark contrast and the retardation color disappeared gradually with an increase in temperature. Finally, CCPTO reached to the isotropic phase (liquid phase) at 300 °C. This gradual transition of CCPTO is due to high viscosity and the molecular weight distribution of CCPTO. Alkoxyl chains substituted to the terminal of the conjugated side chains have flexibility, which is to be advantage to show liquid crystalline phases.
4. Conclusion
A series of conjugated polymers having phenylene–thiophene backbones and conjugated phenylene–vinylene side chains were synthesized by Migita–Kosugi–Stille type coupling polycondensation. CCPTO has the highest molecular weight among them due to high polymerization activity. UV–vis absorption and photoluminecence spectra were examined for the polymer. Optical activity of the polymer indicates that chiral groups in the substituents induce helical structure of the polymers. Liquid crystallinity of the polymer comes from a super mesogen consisting of main chain and side chain, showing nematic LC.
Acknowledgment
We would like to thank the Chemical Analysis Center of University of Tsukuba and glass work shop of University of Tsukuba.
Disclosure statement
No potential conflict of interest was reported by the authors.
References
- McCulloch I, Heeney M, Bailey C, et al. Liquid-crystalline semiconducting polymers with high charge-carrier mobility. Nat. Mater. 2006;5:328–333.10.1038/nmat1612
- Granström M, Inganäs O. White light emission from a polymer blend light emitting diode. Appl. Phys. Lett. 1996;68:147–149.
- Yu G, Gao J, Hummelen JC, Wudl F, Heeger AJ. Polymer photovoltaic cells: enhanced efficiencies via a network of internal donor-acceptor heterojunctions. Science. 1995;270:1789–1791.10.1126/science.270.5243.1789
- Chen J, Cao Y. Development of novel conjugated donor polymers for high-efficiency bulk-heterojunction photovoltaic devices. Acc. Chem. Res. 2009;42:1709–1718.10.1021/ar900061z
- Moroni M, Le Moigne J, Luzzati S. Rigid rod conjugated polymers for nonlinear optics: 1. Characterization and linear optical properties of poly(aryleneethynylene) derivatives. Macromolecules. 1994;27:562–571.10.1021/ma00080a034
- Kim IB, Erdogan B, Wilson JN, Bunz UHF. Sugar-poly(para-phenylene ethynylene) conjugates as sensory materials: efficient quenching by Hg2+ and Pb2+ ions. Chem. Eur. J. 2004;10:6247–6254.10.1002/(ISSN)1521-3765
- Xue C, Velayudham S, Johnson S, et al. Highly water-soluble, fluorescent, conjugated fluorene-based glycopolymers with poly(ethylene glycol)-tethered spacers for sensitive detection of Escherichia coli. Chem. Eur. J. 2009;15:2289–2295.10.1002/chem.v15:10
- Shirakawa H. Synthesis and characterization of highly conducting polyacetylene. Synth. Met. 1995;69:3–8.10.1016/0379-6779(94)02340-5
- Anger E, Iida H, Yamaguchi T, et al. Synthesis and chiral recognition ability of helical polyacetylenes bearing helicene pendants. Polym. Chem. 2014;5:4909–4914.10.1039/C4PY00692E
- Ohshimizu K, Takahashi A, Rho Y, Higashihara T, Ree M, Ueda M. Synthesis and characterization of polythiophenes bearing aromatic groups at the 3-position. Macromolecules. 2011;44:719–727.10.1021/ma102661s
- Zhu X, Plunkett KN. Controlled regioregularity in oligo(2-methoxy-5-(2′-ethylhexyloxy)-1,4-phenylenevinylenes. J. Org. Chem. 2014;79:7093–7102.10.1021/jo501266g
- Greenwald Y, Cohen G, Poplawski J, Ehrenfreund E, Speiser S, Davidov D. Transient photoconductivity of acceptor-substituted poly(3-butylthiophene). J. Am. Chem. Soc. 1996;118:2980–2984.10.1021/ja953762g
- Shi S, Shi K, Qu R, et al. Alkylphenyl substituted naphthodithiophene: a new building unit with conjugated side chains for semiconducting materials. Macromol. Rapid Commun. 2014;35:1886–1889.
- Li H, Parameswaran M, Nurmawati MH, Xu Q, Valiyaveettil S. Synthesis and structure−property investigation of polyarenes with conjugated side chains. Macromolecules. 2008;41:8473–8482.10.1021/ma801506c
- Cui C, Min J, Ho CL, et al. A new two-dimensional oligothiophene end-capped with alkyl cyanoacetate groups for highly efficient solution-processed organic solar cells. Chem. Commun. 2013;49:4409–4411.10.1039/c3cc38920k
- Duan C, Chen KS, Huang F, et al. Synthesis, characterization, and photovoltaic properties of carbazole-based two-dimensional conjugated polymers with donor-π-bridge-acceptor side chains. Chem. Mater. 2010;22:6444–6452.10.1021/cm1027157
- Meng H, Wudl F. A robust low band gap processable n-type conducting polymer based on poly(isothianaphthene). Macromolecules. 2001;34:1810–1816.10.1021/ma001689v
- Innami Y, Kawashima H, Kiebooms RHL, Aizawa H, Matsuishi K, Goto H. Synthesis and properties of poly(isothianaphthene methine)s with chiral alkyl chain. Materials. 2012;5:317–326.10.3390/ma5020317
- Kiebooms RHL, Aleshin A, Hutchison K, Wudl F, Heeger AJ. Doped poly(3,4-ethylenedioxythiophene) films: thermal, electromagnetical and morphological analysis. Synth. Met. 1999;101:436–437.10.1016/S0379-6779(98)01121-7
- Kawabata K, Goto H. Synthesis and optical properties of 1,1-binaphthyl-thiophene alternating copolymers with main chain chirality. J. Mater. Chem. 2012;22:23514–23524.10.1039/c2jm35594a
- Kawashima H, Kawabata K, Goto H. Synthesis and double doping behavior of a poly(p-phenylenevinylene)s bearing conjugated side chains. J. Polym. Sci. A Polym. Chem. 2012;50:1530–1538.10.1002/pola.v50.8
- Young RJ, Lovell PA. Introduction to polymers. 3rd ed. London: Chapman & Hall; 1991. Chapter 3, Step polymerization; p. 21–41.10.1007/978-1-4899-3176-4
- Lassahn PG, Janiak C, Oh JS. Borane activators for late-transition metal catalysts in norbornene polymerization. Macromol. Rapid Commun. 2002;23:16–20.10.1002/(ISSN)1521-3927
- Lee Y, Sadki S, Tsuie B, Reynolds JR. A new narrow band gap electroactive polymer: poly[2,5-bis{2-(3,4-ethylenedioxy)thienyl}silole]. Chem. Mater. 2001;13:2234–2236.10.1021/cm010138w
- Neugebauer H, Kvarnström C, Brabec C, et al. Infrared spectroelectrochemical investigations on the doping of soluble poly(isothianaphthene methine) (PIM). J. Chem. Phys. 1999;110:12108–12115.10.1063/1.479146
- Okuda Y, Lakshmikantham MV, Cava MP. A new route to 1,3-disubstituted benzo[c]thiophenes. J. Org. Chem. 1991;56:6024–6026.10.1021/jo00021a012
- Choi JW, Han MG, Kim SY, Oh SG, Im SS. Poly(3,4-ethylenedioxythiophene) nanoparticles prepared in aqueous DBSA solutions. Synth. Met. 2004;141:293–299.10.1016/S0379-6779(03)00419-3
- Ocampo C, Oliver R, Armelin E, Alemán C, Estrany FJ. Electrochemical synthesis of poly(3,4-ethylenedioxythiophene) on steel electrodes: properties and characterization. Polym. Res. 2006;13:193–200.10.1007/s10965-005-9025-7
- Chen WT, Bowmaker GA, Seakins JM, Cooney RP. Modified synthetic routes to poly(isothianaphthene). Synth. Met. 2002;128:215–220.10.1016/S0379-6779(02)00022-X
- Kawabata K, Goto H. Liquid crystalline π-conjugated copolymers bearing a pyrimidine type mesogenic group. Materials. 2009;2:22–37.10.3390/ma2010022
- Ohkawa S, Ohta R, Kawabata K, Goto H. Polymerization in liquid crystal medium: preparation of polythiophene derivatives bearing a bulky pyrimidine substituent. Polymers. 2010;2:393–406.10.3390/polym2040393