Abstract
The present work describes the synthesis, properties, and sensitive behavior of a set of copolymers based on itaconic anhydride with different molar ratios of 3,9-divinyl-2,4,8,10-tetraoxaspiro[5.5] undecane prepared through radical polymerization process, in the presence of 2,2-azobisisobutyronitrile as initiator. The chemical structure of the prepared copolymers was confirmed by FTIR and 1H NMR. The macromolecular compounds were characterized from the viewpoint of their thermal stability. The sensitivity of the new structures was evaluated by determining the hydrodynamic radius in interdependence with environmental conditions, and rheological properties were studied in terms of shear rate and viscosity. The versatility and untapped potential of these stimuli-sensitive polymeric systems generated by both comonomers – network formation, biodegradability and biocompatibility, gel formation capacity, binding properties, amphilicity, good oxidative and thermal stability, good films formers, acid pH sensitivity, the possibilities to modify the anhydride ring through suitably reactions – makes them promising agents for pharmaceutical delivery systems or support for bioactive compounds, among other applications.
Introduction
The synthesis of new multifunctional, stimuli-sensitive polymer systems with controlled structure has become a major component of the current state-of-the-art in the science and engineering of advanced materials used in medicine.[Citation1] In the research field of drug delivery, temperature-sensitive polymers are probably the most commonly studied class of environment-sensitive compound systems.[Citation2] In addition, the use of materials from renewable resources and high degree of recycling or degradation are essential advantages for highly demanding applications.[Citation3,4]
In a previous article, our group studied a copolymer based on maleic anhydride (MAh) and 3,9-Divinyl-2,4,8,10-tetraoxaspiro[5.5] undecane (U), used as polymeric matrices for potential bioapplications.[Citation5] Itaconic anhydride (ItA), owing to the functional similarity to MAh, has been mentioned as an alternative in applications comparable to those containing MAh.[Citation6] Though its free-radical homopolymerization and copolymerization with a variety of other monomers have been reported, ItA has not been analyzed as intensively. ItA can copolymerize and form polymers containing many anhydride rings that MAh cannot achieve due to its limitation of producing alternating copolymers only. Studies show that ItA is generally more reactive than MAh is, because it forms highly reactive tertiary radicals.[Citation7,8] Also, it is an environmentally friendly substitute (owning to its availability from abundant renewable resources) of MAh, which is prepared from petrochemical resources.[Citation9,10]
This approach employs ItA and U as starting materials, possessing various potential for bioapplications. Because of its unique structure and characteristics, ItA is a useful material for bioindustry and is frequently used in medicine. ItA is an environmentally friendly monomer, obtainable from renewable resources, by fermentation processes. Also, is attracting increasing attention, due to the fact that it is biocompatible, has a bioactive nature and the inclusion of ItA into macromolecular chain structure will induce partly biodegradable character for the prepared compounds. Another property that makes ItA a valuable compound is its structure. ItA has two functional groups: the anhydride ring, which can undergo different types of ring-opening reaction, and a double bond, which can take part in free radical polymerization. Moreover, these functional groups can be used as coupling sites for increasing the compound’s biocompatibility, bioinductivity, adhesion or other physical properties, and offer alternatives for obtaining controlled delivery systems or support for bioactive compounds.[Citation11–15]
ItA can be homopolymerized or copolymerized with a variety of other monomers [Citation16–18] and through the years it has been used for various purposes, such as: ionomeric materials,[Citation19] compatibilizers,[Citation20] ‘acid hardener’ for epoxide resins, photoresist materials for microlithography, transparent plastics, and others. In the pharmaceutical and medical area, derivatives of ItA have been shown to possess biological activity, especially for antitumor or cancer treatments,[Citation21,22] or they can be used as a support for drug release.[Citation23,24] ItA is relatively an innocuous material, with regard to toxicity.[Citation25] Besides its properties, which could provide a biomaterial-like usage, the monomer is highly reactive at normal temperature (due to anhydride ring) without the need of catalyzer or high temperature.[Citation26] This compound has an enormous potential in the area of biomaterials by virtue of its biocompatibility, bioactivity, structure, and reactivity.[Citation27]
U, a co-monomer with spiroacetal moiety and crosslinking capacity, owing to these functional groups induces by incorporation into macromolecular chains advantageous characteristics like good flexibility and tensile strength, thermal stability, biodegradability, binding properties, acid pH sensitivity, as well it enhances the solubility, biocompatibility and adhesive properties for its copolymers. The literature data describe developments in the synthesis of copolymers from spiroortho-esters, which also found applicability for bio-medical field.[Citation28,29]
In this context, copolymers based on ItA and U through radical polymerization were synthesized. The interest for this type of copolymers is entirely justified taking into consideration the advantages generated by both comonomers – network formation, biodegradability and biocompatibility, gel formation capacity, binding properties, amphilicity, good oxidative and thermal stability, good films formers, acid pH sensitivity, the possibilities to modify the anhydride ring through suitably reactions – and thus the usefulness of these compounds in a wide range of applications is an understatement.
Experimental
Materials
All reagents were of analytical purity and were used without further purification: 3,9-divinyl-2,4,8,10-tetraoxaspiro[5.5] undecane (U) (purity 98%, Sigma–Aldrich), ItA (purity 95%, Aldrich), 2,2′-Azobis(2-methylpropionitrile) (AIBN) (purity 98%, Sigma–Aldrich). The solvents used 1, 4 dioxane (≥99.0%) and diethyl ether (for precipitation) were obtained from Sigma-Aldrich. The water used in other experiments was purified using an Ultra Clear TWF UV System.
Synthesis processes
Four copolymer variants of poly(ItA-co-3,9-divinyl-2,4,8,10-tetraoxaspiro[5.5] undecane)(P(ItAU) were taken into study resulted from different molar ratio between the co-monomers, ItA:U, respectively, from 1:0.5 to 1:2.
The copolymers were synthesized through radical polymerization process using AIBN as initiator and 1,4-dioxane as solvent. The total monomer concentration was 20%, and the initiator concentration was 0.9% based on the concentration of the two comonomers. The continuous polymerization process was conducted under nitrogen atmosphere, at 75 °C, in a constant temperature bath, with a stirring rate of 250 rpm, and it was carried out for 17 h. After cooling, the reaction mixture was added dropwise into diethyl ether when the copolymer was precipitated, and then washed several times with diethyl ether and dried in a vacuum oven at room temperature and 600 mm HG vacuum for 24 h. The recipes of the reactions are presented in Table .
Table 1. Copolymerization recipe series (mmol).
Copolymer characterization
FT-IR spectra of the prepared polymer samples – P(ItAU) – were recorded on a Vertex Brucker Spectrometer in an absorption mode ranging from 400 to 4000 cm−1. The polymer sample was grounded with potassium bromide (KBr) powder and compressed into a disc to analysis. The spectra were acquired at 4 cm−1 resolution as an average of 64 scans.
1H NMR investigations were performed with a Bruker Avance DRX400 NMR spectrophotometer Rheinstetten (Germany) with 16 scans and 0.1 Hz FID resolution, equipped with a 5-mm broad band probe. The samples were dissolved in dimethyl sulfoxide. The analyses were carried out at 25 °C and chemical shifts were reported in ppm using tetramethylsilane as the internal reference, and confirms the compound composition.
The molecular weights of the synthesized copolymers were evaluated using Zetasizer Nano ZS instrument, and as well the second virial coefficient, A2, of the samples was determined. The Zetasizer Nano ZS measures the intensity of static light scattering, scattered light of various concentrations of sample at one angle (173°). The intensity of scattered light that a macromolecule produces is proportional to the product of the weight-average molecular weight (M) and the concentration of the macromolecule. The samples that have a relatively large size exhibit scattering profiles that are non-isotropic, with measured intensities that are dependent on the angle of observation. As the particle size decreases however, the sample scattering becomes isotropic and the angular dependence of the measured intensity is minimized. Taking this in consideration, the Zetasizer Nano system provides a method for measuring the molecular weight of polymers at only an angle using the relationship between the intensity of scattered light and their molecular weight given by the Rayleigh equation (Equation (Equation1(1) )). A plot of KC/Rθ vs. C is expected to be linear with an intercept equivalent to 1/M and a slope equal to the second virial coefficient A2. The following relations (Rayleigh equation) are valid:
(1)
where(2)
(3)
and(4)
where: K – optical constant, M – molecular weight, A2 – 2nd virial coefficient, C – polymer concentration, Rθ – Rayleigh ratio of the sample, P(θ) – shape factor; n0 is the refractive index of the solvent, λ is the laser wavelength, NA – the Avogadro constant, and dn/dc is the refractive index increment of the scattering species in the solvent used. Rg – radius of gyration; θ – measurement angle, IA – intensity of sample, IT – intensity of standard (toluene), nT – standard toluene refractive index, and RT – Rayleigh ratio of standard (toluene).
Thermal analysis of the copolymers was performed using a Jupiter STA 449 F1 (Netzsch) instrument. The samples were previously maintained in a controlled humidity atmosphere, respectively, in the presence of CaCl2 inorganic salt. 7.5–8 mg quantities of samples were heated in an open Al2O3 crucible, under 50 ml/min−1 nitrogen flow rate. Runs were performed in dynamic mode from room temperature up to 600 °C at a 10 °C/min heating rate.
Hydrodynamic diameter of the copolymers was estimated by dynamic light scattering technique using a Zetasizer model Nano ZS device, with red laser 633 nm He/Ne from Malvern Instruments, UK. The system uses a non-invasive back scatter technology (which reduces the multiple scattering effects) wherein the optics is not in contact with the sample, back scattered light being detected. During determinations, the Mie method is applied over the whole measuring range from 0.6 nm to 6 μm. The determinations were made on 2 ml sample of copolymers solution of maximum 1% concentration. The experiments were conducted to explore the dependence of hydrodynamic radius on the temperature effect, determination performed in the range between 20 and 40 °C.
Oscillatory and rotational rheological measurements
The rheological behavior of P(ItAU) copolymers was monitored with a Physica MCR 301 rheometer (Anton Paar), using a plate–plate geometry of 25 mm as measurement system. The measurements were performed at constant temperature (25 ± 0.1 °C). The samples were placed on the plate and were closed to a gap of 200 µm. Before the tests were started, the extra sample that extends beyond the circumference of the ‘25 mm diameter’ plate was removed.
Results and discussion
The new copolymer systems have an idealized structure as it is presented in Figure .
Figure 1. Schematic illustration of poly(ItA-co-3,9-divinyl-2,4,8,10-tetraoxaspiro[5.5]undecane) (P(ItAU)) copolymer structure.
![Figure 1. Schematic illustration of poly(ItA-co-3,9-divinyl-2,4,8,10-tetraoxaspiro[5.5]undecane) (P(ItAU)) copolymer structure.](/cms/asset/44bad090-6d82-4b91-89a7-1bb08adc38d6/tdmp_a_1078111_f0001_b.gif)
FT-IR spectra
The structure of the resulted copolymers was confirmed by FT-IR spectroscopy. The correspondingly aligned spectra of all samples are illustrated in Figure .
P(ItAU) IR spectra show characteristic absorption bands for both comonomers, in agreement with the literature data.[Citation30] The absorptions for ItA are at 1856 cm−1 (low intensity) due to asymmetric stretching modes of carbonyl (C=O), while the band at ~1780 cm−1 (high intensity) belongs to the symmetric stretching of carbonyl (C=O). These peaks are characteristic for the 5-member anhydride unit, indicating that the polymerization did not disrupt the ItA moieties. Also, characteristic peaks for anhydride group appear at ~1216–1164 cm−1 (cyclic C–O–C) and ~1008 cm−1 due to the acyclic C–O. The spiroacetal moieties inclusion of U monomer is confirmed by strong bands in the region of 1000–1200 cm−1 (due to C–O–C stretching) which are consistently more intense with the addition of U in the synthesis.
The new bonds intervened between ItA and U are observed as strong bands among 2959 and 2856 cm−1 indicating the streching of C–H group, and which are also confirmed by the attenuation of peaks corresponding to C=C double bond at ~1610 cm−1. New hydrogen bonds are revealed in the 3500–3200 cm−1 range as broad absorption. The increase of U content brought as well supplementary vibration peaks from 615 to 730 cm−1 and among 1024–1076 cm−1 (specific to the spiroacetal ring) which are changing their intensity and shape depending on the amount of the monomer. The characteristic peaks of the copolymers resulted from FT-IR spectra are presented in Table .
Table 2. The absorption peaks corresponding to P(ITAU)n copolymers.
1H-NMR spectra
1H-NMR spectroscopy sustains the IR analysis and confirms the composition of the synthesized copolymers. The 1H-NMR spectra of P(ItAU) copolymers are illustrated in Figure .
Characteristic signals in agreement with the molecular structure [Citation4] of monomers are shown at around from 3.2 to 3.9 ppm– the protons correlated to H–C–O–ethers group of U and the hydrogen from the methylene group in the anhydride ring; at 4.3, 4.9 ppm (–CH2=C–), 5.3, 5.4 ppm (=CH2–CH–) – characteristics for U, and at 5.8 ppm (=CH–) – specific for U and ItA. The signals registered in the region between 1 and 2 ppm corresponded to R–CH2, R–CH and are attributed to the shift δ at 2.1 ppm which can be assigned to the new–CH– bond formed between the comonomers. The attenuation or almost disappearance of the signal corresponding to the vinylidene protons of ItA at 6.4 ppm confirms the synthesis of the copolymer. Differences between the compositions of the copolymer variants are also evidenced in the spectra. Thus, the intensity of the signals corresponding to U protons is in correlation with the monomer content in the synthesis. The solvent peak is exhibited at 2.5 ppm.
Molecular weights
The determined molecular weight of the synthesized copolymers is in good agreement with the ratio between the comonomers and the envisaged structure, as it is presented in Figure , of the synthesized macromolecular compounds. Figure illustrates the evolution of the molecular weight in interdependence with the ratio between ItA and U during synthesis. For example, at higher content of U, which corresponds at a cross-linked polymer structure, an increased molecular weight was determined. Thus, the molecular weight of P(ItAU)1:0.5 was 31.95 kDa, while that of P(ItAU)1:1,5 was 69.60 kDa.
Thermal analysis
The main characteristic data resulted from thermal investigations are presented in Table . TG and DTG curves concerning the thermal behavior and changes in thermal stability of the synthesized copolymer variants are depicted in Figure . Thermograms underline the influence of composition on thermal stability of the polymeric structures.
Table 3. Thermal parameters of studied copolymers.
Significant differences can be observed in the behavior at heating of the synthesized polymeric compounds. As it can be seen, P(ItAU)1:1,5 and P(ItAU)1:2 copolymers are more stable and starts degradation later than the other two samples with content reduced of U. P(ItAU)1:0,5 and P(ItAU)1:1 shows three thermal degradation stages, while P(ItAU)1:1,5 and P(ItAU)1:2 have only two stages of degradation. The copolymers exhibited a slightly loss of mass prior to the main degradation process, which is believed to be due to water absorption.
All of copolymers present one main process of thermal degradation between 370 and 470 °C with important weight loss: 49.55 wt.% for P(ItAU)1:0.5, 51.90 wt.% for P(ItAU)1:1, 63.76 wt.% for P(ItAU)1:1.05, respectively, 51.70 wt.% in case of P(ItAU)1:2.
Analyzing the thermal stability as a function of T10 and T20 temperatures corresponding to 10 or 20 % weight loss, it can be observed the reduced thermal stability for P(ItAU)1:0.5 sample. This thermal decomposition process can be attributed to the scission of C–C linkages and release of aliphatic fragments of different molecular weights, and the reduced amount of U corresponds to less cross-linked chains concretized as well in reduced thermal stability. A small amount of carbonaceous residue for the analyzed copolymers (13.37, 12.17, 13.37, respectively, 10.90 wt.%) remained at 600 °C. The char is considered to be a condensed cross-linked structure formed by decarboxylation or decarbonylation of the anhydride ring, which needs oxygen to completely decompose.[Citation31]
The addition of U comonomer in different molar ratio improves the thermal behavior of the copolymer as it can be observed from the TG curves, owing to the cross-linked generated polymer structure. In terms of thermal stability is observed that P(ItAU)1:1,5 and P(ItAU)1:2 copolymers are more stable than P(ItAU)1:0.5 and P(ItAU)1:1.
Hydrodynamic radius of P(ItAU) copolymers
The particle size trend, investigated by the hydrodynamic diameter variation, was observed in interdependence with the environmental conditions, respectively, between 20 and 40 °C domain of temperature.
The sensitivity of the copolymers with temperature is evidenced in Figure where it is illustrated the variation of the particle size of the copolymers. Relatively similar behavior was registered for the synthesized copolymer variants. Thus, the particles size of the copolymers first is slowly decreased, followed by a suddenly decrease in the range from 30 to 34 °C. We can appoint that to a transition at 34 °C followed by an increase in hydrodynamic diameter and a second transition at 37 °C. These transitions are more evident for an increased amount of U comonomer, and they reach the utmost form for the copolymer variant with the maximum content of U.
Thus, the transition at 34 °C exhibited by the copolymer variants observed in Figure (a)–(c) is influenced by the copolymer composition, as it can be seen in Figure (d). It can be also noticed a recursive relation between the hydrodynamic diameter and the monomers ratio. An increase in the polymeric particles size can be observed with the addition of U in the synthesis (1:0.5; 1:1; 1:1.5 M ratios) as it is expected if we take a look to the schematic illustration of the copolymer structure. The increase in the copolymers particle radius is normal taking into account the occurring steric hindrances as a result of the axial conformation of the spiroacetal moiety. But an additional quantity of U monomer in the synthesis (1:2 M ratio) determines the decrease in polymer size. This behavior can be understood by the random coil conformation of the copolymers, which can be folded and packed through physical interactions intervened between macromolecular chains. Thus, the spiroacetal moiety, which has as well acid pH sensitivity, has the capability for interactions on ether oxygen, such as hydrogen bonds or coordinate bonds with other functional groups that inducing dynamic change of the stereochemistry through anomeric effect performed once with an increase in temperature and in condition of thermal agitation.[Citation32] The spiroacetal moiety is getting an appropriate conformation, which can justify the decrease in the copolymer particles dimension. This investigation confirms the sensitivity of the synthesized compounds at temperature.
Oscillatory and rotational rheological measurements
As it is well-known polymers exhibit a random chain (or Gaussian) conformation, which corresponds to a random distribution of trans and gauche states and to a favorable chain configuration due to intermolecular interactions.[Citation33] Our synthesized compounds were rheological investigated confirming the changes in configuration and conformation as a result of different ratios between comonomers.[Citation34]
From the rotational tests, the viscosity of the polymeric solutions as a function of shear rate was determinate. In Figure is illustrated the shear viscosity η plotted against shear rate γ for poly(ItAU) solutions. From the profile of the viscosity curve of the P(ItAU)1:2 sample, a Newtonian plateau is observed at higher shear rates. Also, this sample exhibits the lowest values of shear viscosity, over the shear rate range 0.01–100 s−1, at the same time, for P(ItAU)1:1 copolymer was recorded the highest values of viscosity. This behavior attests the composition of the copolymers and as well the absence of crosslinks in case of P(ItAU)1:2, initial steric hindrances in case of P(ItAU)1:1 and P(ItAU)1:0.5.
Figure 7. Variation of viscosity with shear rate for the copolymers solutions of various concentrations.
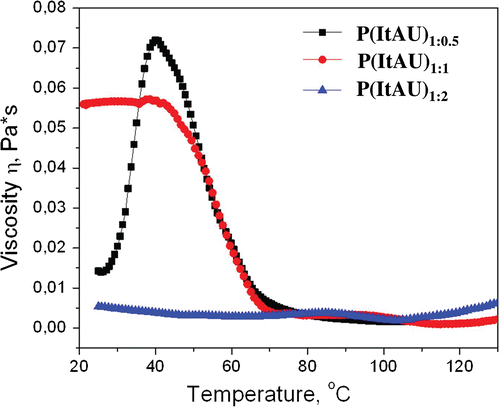
It is observed that for the P(ItAU)1:0.5 and P(ItAU)1:1 copolymers, the shape of the viscosity curves clearly shows decreasing viscosity values with an increase in shear rate, indicating that the solutions behave as non-Newtonian (pseudoplastic), shear-thinning fluids. This suggests that at higher shear rates, the intermolecular junctions are disrupted, and shear thinning is observed.
Conclusions
The general goal was to investigate the polymerization process between ItA and U, in order to obtain polymeric network structure with sensitivity to temperature and the ability further to react or host various molecular compounds. Copolymers of various compositions with different contents of U were successfully synthesized.
The chemical structure of P(ItAU) copolymers was confirmed by FT-IR and 1H-NMR investigations. The sensitive behavior of the copolymers was evaluated in interdependence with the variation of temperature. Thermosensitive character is attributed to the presence of spiroacetal moiety. Thermal properties correlated with the changing of the U ratios, as a single structural modification, demonstrates an interesting connection between U ratios and thermal properties. Also, it can be concluded that higher content of U co-monomer corresponds to higher thermal stability. From the rotational and oscillatory test it was observed that P(ItAU)1:1 has the highest viscosity and complex viscosity in comparison with the other samples, behavior in agreement with the copolymer structure.
Taking into account the special characteristics and possibilities of the new prepared systems, further studies as extension, on these polymer properties and on their application are under progress in our laboratory.
Disclosure statement
No potential conflict of interest was reported by the authors.
Additional information
Funding
References
- Aguilar MR, Elvira C, Gallardo A, et al. Smart polymers and their applications as biomaterials. In: Ashammakhi N, Reis R, Chiellini E, editor. Topics in tissue engineering. 2007. p. 1–5. Available from: http://www.oulu.fi/spareparts/ebook_topics_in_t_e_vol3/
- Gil ES, Hudson SM. Stimuli-reponsive polymers and their bioconjugates. Prog. Polym. Sci. 2004;29:1173–1222.10.1016/j.progpolymsci.2004.08.003
- Gandini A. The irruption of polymers from renewable resources on the scene of macromolecular science and technology. Green Chem. 2011;13:1061–1083.10.1039/c0gc00789g
- Okuda T, Ishimoto K, Ohara H, et al. Renewable biobased polymeric materials: facile synthesis of itaconic anhydride-based copolymers with poly(l-lactic acid) grafts. Macromolecules. 2012;45:4166–4174.10.1021/ma300387j
- Chiriac AP, Nita LE, Tudorachi N, et al. Upon synthesis of a polymeric matrix with pH and temperature responsiveness and antioxidant bioactivity based on poly (maleic anhydride-co-3, 9-divinyl-2, 4, 8, 10-tetraoxaspiro (5.5) undecane) derivatives. Mater. Sci. Eng. C Mater. Biol. Appl. 2015;50:348–357.10.1016/j.msec.2015.02.022
- Bell SY, Cowie JMG, McEwen IJ. The miscibility of copolymer blends involving the monomers itaconic anhydride, methyl methacrylate, styrene and acrylonitrile. Polymer. 1994;35:786–790.10.1016/0032-3861(94)90877-X
- Sharabash MM, Guile RL. Homopolymerization of maleic anhydride. J. Macromol. Sci. 1976;10:1033–1054.
- Otsu T, Yang JZ. Radical polymerization of itaconic anhydride and reactions of the resulting polymers with amines and alcohols. Polym. Int. 1991;25:245–251.10.1002/(ISSN)1097-0126
- Ke T, Sun X. Physical properties of poly(lactic acid) and starch composites with various blending ratios. Cereal Chem. 2000;77:761–768.10.1094/CCHEM.2000.77.6.761
- Yamaguchi S, Tanha M, Hult A, et al. Green polymer chemistry: lipase-catalyzed synthesis of bio-based reactive polyesters employing itaconic anhydride as a renewable monomer. Polym. J. 2013;46:1–13.
- Li ZC, Jin W, Li FM. Vesicles prepared from amphiphilic copolymers. React. Funct. Polym. 1999;42:21–30.10.1016/S1381-5148(98)00059-5
- Glauser T, Ranger M, Kalra B, et al. Stabilization of core-shell polymeric assemblies through reversible nucleosides interaction. Polym. Prepr. 2003;44:624–625.
- Cismaru L, Hamaide T, Popa MT. Itaconic anhydride based amphiphilic copolymers: synthesis, characterization and stabilization of carboxyl functionalized. Eur. Polym. J. 2007;43:4843–4851.10.1016/j.eurpolymj.2007.09.023
- Zhou J, Wang L, Wang C, et al. Synthesis and self-assembly of amphiphilic maleic anhydride–stearyl methacrylate copolymer. Polymer. 2005;46:11157–11164.10.1016/j.polymer.2005.08.070
- Michlovská L, Vojtová L, Mravcová L, et al. Functionalization conditions of PLGA-PEG-PLGA copolymer with itaconic anhydride. Macromol. Symp. 2010;295:119–124.10.1002/masy.v295:1
- Miles AF, Cowie JMG. Preparation and characterization of (monomethyl itaconate)-(methyl methacrylate) and of (itaconic anhydride)-(methyl methacrylate) copolymers. Eur. Polym. J. 1991;27:165–170.10.1016/0014-3057(91)90217-C
- Mormann W, Ferbitz J. Copolymers from tert-butyl methacrylate and itaconic anhydride–reactivity ratios and polymer analogous reactions. Eur. Polym. J. 2003;39:489–496.10.1016/S0014-3057(02)00246-X
- Javakhishvili I, Kasama T, Jankovaa K, et al. RAFT copolymerization of itaconic anhydride and 2-methoxyethyl acrylate: a multifunctional scaffold for preparation of ‘‘clickable’’ gold nanoparticles. Chem. Commun. 2013;49:4803–4805.
- Al-Salah HA. Ionomeric blends of poly (ethylene oxide) with poly (styrene-co-maleic anhydride) ionomer: miscibility, crystallization, and melting behavior. J. Appl. Polym. Sci. 2000;77:1–7.10.1002/(ISSN)1097-4628
- Park S, Choe S. The compatibilizing effect of maleic anhydride in ethylene-vinylAcetate (EVA)/ethylene-α-olefin copolymers blends. Macromol. Res. 2005;13:297–305.10.1007/BF03218457
- Drougas J, Guile RL. Copolymerization of itaconic anhydride and styrene, determination of reactivity ratios. J. Polym. Sci. 1961;55:297–302.10.1002/pol.1961.1205516128
- Hodnett EM, Amirmoazzami J, Tai JTH. Anionic polymers and biological activities. Effects of some new polycarboxylic acids on the ascitic sarcoma 180 of mice. J. Med. Chem. 1978;21:652–657.10.1021/jm00205a012
- Glauser T, Ranger M, Kalra B, et al. Preparation of nucleoside methacrylates, PEG-poly (nucleoside) diblocks, and their formation of supramolecular assemblies. Abstr. Pap. Am. Chem. Soc. 2003;225:651–653.
- Cismaru L, Hamaidez T, Popa M. Polymeric surfactants used to stabilize the nanoparticles. Mater. Plast. 2007;44:243–245.
- Booth AN, Taylor J, Wilson RH, et al. The inhibitory effects of itaconic acid in vitro and in vivo. J. Biol. Chem. 1952;195:697–702.
- Grebinişan D, Holban MN, Lionte C, et al. Drug-polymer conjugates with tuberculostatic activity, based on poly (N-vinyl pyrrolidone-alt-itaconic anhydride) and novel aminoacid hydrazides. Polym-Plast. Technol. Eng. 2013;52:1213–1219.10.1080/03602559.2013.798824
- Shang S. Synthesis of comb-like copolymers from renewable resources: itaconic anhydride, stearyl methacrylate and lactic acid. Ann Arbor (MI): ProQuest 2009. p. 9–10.
- Chiriac AP, Nistor MT, Nita LE, et al. Poly(N,N-dimethylacrylamide-co-3,9,divynil-2,4,8,10-tetraoxaspiro (5.5) undecane) synthesis as matrix ensuring intramolecular stratergies for further coupling applications. Rev. Roum. Chim. 2013;58:129–136.
- Nita LE, Chiriac AP, Nistor MT. Upon the emulsion polymerization of 2-hydroxyethyl methacrylate with 3,9-divinyl-2,4,8,10-tetraoxaspiro[5.5]-undecane. Colloids Surf., A. 2011;381:111–117.10.1016/j.colsurfa.2011.03.037
- Osman SM, Newehy MH, Al-Deyab SS, et al. Microwave synthesis and thermal properties of polyacrylate derivatives containing itaconic anhydride moieties. Chem. Cent. J. 2012;6:6–85.
- Shang S, Huang SJ, Weiss RA. Synthesis and characterization of itaconic anhydride and stearyl methacrylate copolymers. Polymer. 2009;50:3119–3127.10.1016/j.polymer.2009.05.012
- Chiriac AP, Nita LE, Nistor MT. Nano-network with dual temperature and pH responsiveness based on copolymers of 2-hydroxyethyl methacrylate with 3,9-divinyl-2,4,8,10-tetraoxaspiro[5.5]-undecane. J. Nanopart. Res. 2011;13:6953–6962.10.1007/s11051-011-0605-7
- Gedde U. Polymer physics. Dordrecht: Springer Science & Business Media; 1995. p. 16–39.
- Halley PJ, George GA. Chemorheology of polymers from fundamental principles to reactive processing. Cambridge: Cambridge University Press; 2009. p. 169.10.1017/CBO9780511581403