Abstract
In order to obtain amorphous thin films of a polydiacetylene, a polyisophthalate containing a push–pull diacetylene chromophore was synthesized by the reaction of p-(N,N-diethanolamino)-p’-nitrodiphenylbutadiyne with isophthaloyl chloride in anhydrous 1-methyl-2-pyrrolidone. The polymer was soluble in organic solvents, such as chloroform, tetrahydrofuran, dimethylformamide, etc., and films with good optical quality were obtained by spin coating from its N-methyl-2-pyrrolidone solution. It was characterized by 1H-NMR, IR, DSC, UV–Visible, X-ray, etc. The diacetylene groups underwent polymerization in the film when irradiated with UV light at 120 °C under Ar atmosphere, and it contained free radicals with a radical concentration of 1016 radicals/g. The third-order nonlinear optical susceptibility, χ(3), of the polymer film measured by the Z-scan technique at 1064 nm was not noticeable, but when the film was heated and irradiated with UV light, a χ(3) value of 6.4 × 10−10 esu was obtained due to increase in π-conjugation by the formation of polydiacetylene in the side chain.
1. Introduction
Since Wegner reported the solid-state polymerization of diacetylenes (DAs) to form crystalline polydiacetylenes (PDAs) in 1969,[Citation1] a great number of studies with main interest in their third-order nonlinear optical properties (3-NLO) have been developed.[Citation2–7] But the lack of processability due to their crystalline nature, limit its optical applications, although some DAs having large substituents are known to give soluble polymers.[Citation8–10] Langmuir–Blodgett membranes of PDAs have also been investigated,[Citation11–13] but the method does not seem adequate for practical applications because of film fragility. Amorphous films with conjugated structures can be obtained by heating or irradiation of aromatic DAs. They form diradicals, which couple each other giving amorphous oligomers.[Citation14] However, many aromatic DAs have high melting points and the products are generally difficult to process. Molten-state polymerization of diphenylbutadiyne with gamma irradiation above its melting point has been reported obtaining amorphous oligomers soluble in solvents.[Citation15] There is an alternative choice for obtaining PDA films by their preparation from soluble polymers containing DA groups either in their main chains or side chains, followed by their polymerization to develop PDA network in the films. In the case, when the DA groups are aligned in polymer crystallites in the manner that topochemical polymerization takes place, films containing PDA network are obtained.[Citation16,17] However, the films are often opaque due to light dispersion by the crystallites, since it is not easy to obtain crystallites smaller than the wavelength of visible light. When the DA-containing polymers are amorphous, this topochemical polymerization does not occur, but random cross-linking takes place when heated or irradiated giving polymer films with excellent optical quality.[Citation18–22] In this work, a soluble polyisophthalate containing an aromatic DA was synthesized and characterized, and its third-order nonlinear optical susceptibility was determined by the Z-scan technique.
2. Experimental
All reagents employed were supplied by Aldrich and used without further purification, with the exception of isophthaloyl dichloride that was recrystallized from hexanes before use.
2.1. Diacetylene preparation
4-N,N-Bis(hydroxyethyl)aminophenyl-4’-nitrophenylbutadiyne was prepared using the same procedure as previously reported [Citation22] except that the N,N-bis(hydroxyethyl)-4-iodoaniline was prepared employing the method reported by Cross and Davis [Citation23]. The desired chromophore (Scheme , I) was isolated by solubility difference and recrystallized from acetone to afford orange crystals in 33% yield and melting at 215 °C (DSC). UV–vis, λmax (THF): 424 nm. FT-IR (ν, cm−1): 3314 (OH), 2929 (CH2), 2203 (C≡C), 1597 (C = Carom). 1H-NMR (400 MHz, acetone-d6), δ (ppm): 3.63 (t, 4H), 3.77 (m, 4H), 4.10 (t, 1H), 6.79 (d, 2H), 7.39 (d, 2H), 7.81 (d, 2H), 8.28 (d, 2H). The 1H-NMR spectrum is shown in Figure , where the peaks at 2.76 and 2.05 correspond to the acetone-d6. Elemental analysis % Calcd: C, 68.56; H, 5.18; N, 8.00. % Found: C, 68.30; H, 5.04; N, 7.87.
Figure 4. DSC thermogram of diacetylenic chromophore (solid line) and DA-containing polyisophthalate (broken line).
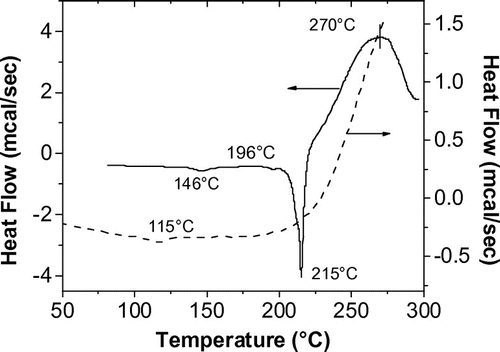
Figure 5. TGA of the diacetylenic chromophore (solid line) and DA-containing polyisophthalate (broken line).
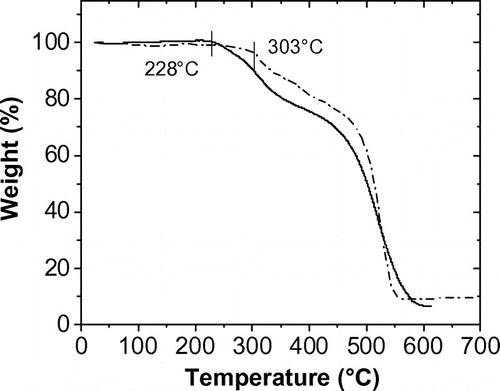
Figure 6. UV–vis absorption spectra of chromophore (solid line) and polymer (dotted line) in THF solution at 1 × 10−4 M and polymer film (broken line).
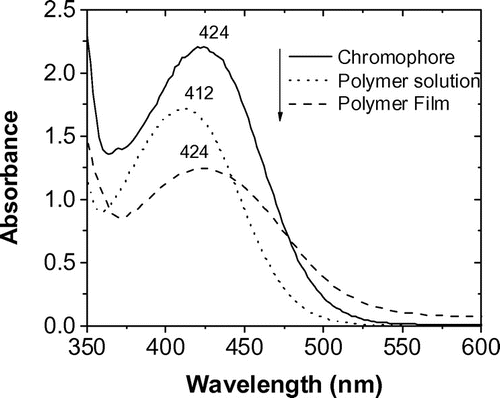
Figure 7. UV–vis absorption spectra of the polymer films. Before (a) and after (b) heating at 120 °C with UV (1 h) in Ar.
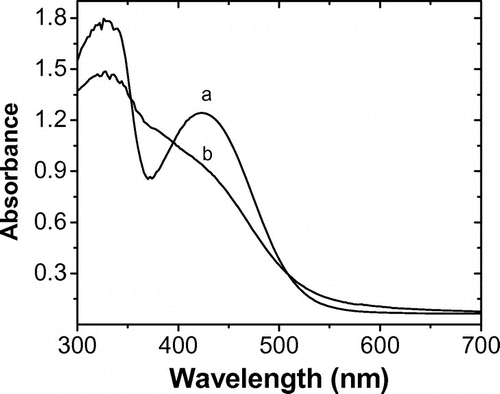
2.2. Polymer preparation
The polymer (Scheme , II) was obtained by the condensation of the chromophore (I) with equimolar amount of isophthaloyl chloride in anhydrous N-methyl-2-pyrrolidone (NMP). After 5 h, the solution was poured into ethanol and an orange polymer was obtained. It was purified twice by reprecipitation from chloroform/ethanol and washed with acetone. 1H-NMR of the polymer taken in DMF-d7 is shown in Figure . The peaks at 8.03, 3.5, 2.92 and 2.75 are of DMF-d7. The residual peaks at 2.1 and 1.08 are from remaining acetone and ethanol used for purification. FT-IR spectra of the chromophore and the polymer are shown in Figure . Elemental analysis % Calcd: C, 70.00; H, 4.20; N, 5.80. % Found: C, 69.08; H, 4.30; N, 5.65. Tg = 115 °C, ηinh = 0.12 dl/g.
2.3. Characterization
1H-NMR spectra were recorded on a Bruker Avance 400 MHz spectrometer. FT-IR spectra were taken on a Thermo Scientific Nicolet 6700 FT-IR spectrometer. X-ray powder diffraction (XRD) was recorded on a Siemens D-500 diffractometer using Cu-Kα radiation of 1.540 Å. UV-Visible spectra were taken on a Varian Cary 400 UV–vis spectrophotometer, in THF solution at 1 × 10−4 M. Thermal analyses were performed using a thermogravimetric analyzer (TGA), model TGA 2950; a differential scanning calorimeter (DSC), model MDSC 2910 and a thermomechanical analyzer (TMA), model TMA 2940 from TA Instruments, with a heating rate of 10 °C/min under nitrogen atmosphere. The inherent viscosity was determined in dimethylformamide (DMF) solution, with a concentration of 0.78 g/dl at 25 °C using an Ubbelohde viscometer. The elemental analyses for C, H, and N contents were performed by RIKEN materials characterization team. The electron spin resonance (ESR) spectra were taken using a JEOL ESR spectrometer Model RE3X. The polymer was placed in a quartz tube with an inner diameter of 3 mm supplied by Wilmad LabGlass, and the tube was degassed and sealed in vacuum. The radical concentration was estimated from the double numerical integration of the first derivative of the resonance curve.[Citation24]
2.4. Optical measurements
2.4.1. Z-scan
Polymer films were prepared by spin-coating at 70 °C from its NMP solution (20 wt.%) onto precleaned glass substrates (Micro Slide Glass, Matsunami Glass Ind., Ltd.). Before spin coating, the polymer solution was filtered twice and glass substrates were preheated at 90 °C, in order to remove any moisture on the surface of the glass substrate and allow the maximum evaporation of NMP. The rotation speed of the spin coater was around 2000 rpm for 20 s to distribute homogeneously the polymer solution on the glass substrate and then the speed was reduced to zero. After preparation, the polymer films were dried in high vacuum at 80 °C for 5 h to remove residual solvent. Films thickness was determined by a Sloan Dektak III profilometer. The refractive index was determined using a Jobin–Yvon Model Uvisel spectroscopic ellipsometer. Three separate measurements were done near the Brewster angle, i.e., from 50º to 70º, with an increase by 10º in each step. The wavelength range of these measurements was 260–830 nm, with an increment of 10 nm per step, controlled by an automatic monochromator. DeltaPsi2 software was used in order to fit ellipsometric experimental data to optical properties. A refractive index of 1.6 was determined for the film, obtained by extrapolation to 1064 nm.
The Z-scan experiment was carried out using a 30 ps pulse laser from a Nd:YAG laser at wavelength of 1064 nm at 4.2 μJ, with a repetition rate of 20 Hz. The sample was moved along the path of the laser, from the far field toward a focusing lens. The laser beam was focused onto a 70 micron spot. The sample was scanned along a path 3.5 cm (35 mm) long. The known Z-scan response of a quartz plate and a 1 mm solution of CS2 were used as references to calibrate the experimental setup. After calibration, the third order nonlinear coefficient values of the sample could be calculated from the difference between the normalized peak and valley transmittances, following the standard Z-scan method. The difference between the normalized peak and valley transmittance, ΔTp-v, was measured for the reference and the sample. The third order nonlinear coefficient was calculated using the following equation:
Where Lsample/ref is the optical length of the sample/reference.
3. Results and discussion
3.1. Morphology and thermal properties
X-ray powder diffraction showed a broad halo indicating that the polymer is amorphous. It was soluble in solvents such as chloroform, THF, DMF, etc. Although its inherent viscosity was rather low, 0.12 dl/g, it gave films with excellent optical quality by spin coating from DMF or NMP.
The thermal behaviors of the chromophore and the polymer are shown in Figure . The DSC of the chromophore showed two small endothermic peaks at 146 and 196 °C which were attributed to crystal arrangement before melting at 215 °C, followed immediately by a large exotherm that had an onset at 220 °C and a maximum peak at 270 °C, which corresponded to the thermal reactions of DA groups.[Citation14] Such behavior seems to be a characteristic of aromatic DAs, which do not undergo topochemical polymerization.[Citation15] The polymer had a glass transition temperature, Tg, around 115 °C, which was in accordance with its dimensional change observed in the thermomechanical analysis. The large exothermic peak is due to the thermal oligomerization of DA groups. Both the chromophore and the polymer are thermally stable up to 220 °C as shown in Figure .
The UV–Visible absorption spectra of the chromophore (I) and the polymer (II) in THF solution at 1 × 10−4 M, and that of polymer film are shown in Figure . The maximum absorption of polymer film and the chromophore was the same of 424 nm, while that of polymer solution was blue-shifted to 412 nm. This is due to the intermolecular dipole–dipole interaction in different media. The monomers in a dilute solution are solvated by the solvent molecules having no interaction among them, while the chromophore molecules in the polymer are situated closely to each other maintaining the dipole–dipole interaction in its solution. In the case of spin-coated film, the dipole–dipole interaction is prevented by the centrifugal force during film formation, thus the bathochromic shift is observed.
When the polymer is heated above its Tg the molecular motion of the polymer allowed DA groups to approach each other, and simultaneous UV irradiation forms diradicals which couple each other resulting in conjugated structures [Citation14,25], as shown in Scheme .
The changes on the UV–Visible absorption spectra of the spin coated polymer films before and after heating at 120 °C in argon atmosphere with UV irradiation for 1 h are shown in Figure . The color change is observed as the orange film became brownish after exposure to light and heat. The polymer film without treatment exhibits two absorption bands, one at high energy (330 nm) related to the π–π* aromatic transitions, and other at low energy (424 nm) assigned as the intramolecular charge transfer.[Citation26] When the film was heated and irradiated, the peak at 424 nm disappeared (Figure ), indicating that the DA groups reacted each other, giving a typical absorption spectrum of amorphous PDAs, which is different to that of topochemically polymerized DAs having a clear absorption peaks in the visible region (500–700 nm).[Citation27]
Thermal- and photo-oligomerization of aromatic DAs, which do not undergo topochemical polymerization, proceeds via formation of diradicals which combine each other to form oligomeric species with higher conjugation and more stable diradicals,[Citation14,25] as can be seen in Scheme . The ESR spectra confirmed the presence of stable radicals (Figure ), whose concentration was estimated to be in the order of 1016 radicals/g. These values are smaller than those of aromatic DAs heated at 160–180 °C (1017 radicals/g).[Citation14] This is probably due to restricted molecular motion of DAs linked to polymer backbone.
3.2. Nonlinear optical properties
3.2.1. Z-scan
The films without treatment did not show 3-NLO response as the polymer does not have a conjugated structure, however, when the film was subjected to heat at temperatures around its Tg (120 °C) with simultaneous UV irradiation for 55 min resulted in the formation of oligomeric species as shown in Scheme giving a χ(3) value of 6.4 ± 0.5 x 10−10 esu. This value lies in the majority of organic conjugated molecules. From the Z-scan graph, it can be seen that the sign of the nonlinear refractive index, which is related to the real part of χ(3), is negative. This is indicated by the valley-peak sequence of the experimental curve shown in Figure , which is opposite the peak-valley sequence of the reference sample CS2 (not shown), which has a positive nonlinear refractive index.
4. Conclusion
An amorphous film of a PDA was obtained by thermal and UV irradiation of a DA-containing polyisophthalate film. The UV–Visible spectra clearly showed a typical formation of a random conjugated structure coming from the crosslinking reaction between DAs groups. The π-conjugation of the PDA in the side chain gave a negative χ(3) of 6.4 ± 0.5 × 10−10 esu, determined by the Z-scan technique at 1064 nm. The presence of aromatic DA units in soluble polymers either in the main or side chain may be useful to obtain amorphous films of PDA with high 3-NLO susceptibility.
Disclosure statement
No potential conflict of interest was reported by the authors.
Funding
This work was supported by the DGAPA-UNAM [grant number IN114810, IT100112]; CONACYT [grant number 165507].
Acknowledgements
The authors are grateful to A. Tejeda, J. Barreto, M.A. Canseco and G. Cedillo for their assistance in the X-ray diffraction, ESR spectroscopy, thermal analyses and NMR spectroscopy measurements, respectively. The authors are also grateful to RIKEN materials characterization team for elemental analyses.
References
- Wegner G. Topochemical reactions of monomers with conjugated triple bonds. III. Solid-state reactivity of derivatives of diphenyldiacetylene. J. Polym. Sci., Part B: Polym. Lett. 1971;9:133–144.10.1002/pol.1971.110090211
- Bloor D, Chance RR, editors. Polydiacetylenes. Dordrecht: Martinus Nijhoff Publishers; 1985.
- Chemla DS, Zyss J, editors. Nonlinear optical properties of organic molecules and crystals. Vol. 2. Orlando, FL: Academic Press; 1987.
- Cantow H-J, editor. Advances in polymer science. Polydiacetylenes. Vol. 63. Berlin: Springer-Verlag; 1984.
- Kajzar F, Messier J. Third order nonlinear optical effects in conjugated polymers. In: Bredas JL, Silbey R, editors. Conjugated polymers. Dordrecht: Kluwer Academic Publishers; 1991. p. 517–541.
- Nakanishi H, Matsuda H, Okada S, et al. Nonlinear optical properties of polydiacetylenes with conjugation between the main chain and the substituents. In: Kobayashi T, editor. Nonlinear optics of organics and semiconductors. (Springer Proc. Phys., 36). Berlin: Springer-Verlag; 1989. p. 155–162
- Nalwa HS. Organic materials for third-order nonlinear optics. Adv. Mater. 1993;5:341–358.10.1002/(ISSN)1521-4095
- D’Amore F, Zappettini A, Facchini G, et al. Third order optical characterisation of a π-conjugated polydiacetylene by Maker fringes technique. Synth. Met. 2002;127:143–146.10.1016/S0379-6779(01)00605-1
- Hsu CC, Kawabe Y, Ho ZZ, et al. Comparison of the χ(3) values of crystalline and amorphous thin films of 4-butoxy-carbonyl-methyl-urethane polydiacetylene at 1.06 and 1.3 μm. J. Appl. Phys. 1990;67:7199–7203.10.1063/1.344552
- Zhang Y, Wada T, Sasabe H, et al. Third-order nonlinear optical properties of new soluble poly{1,4-bis[2,4,6-tri(4-n-heptylphenyloxy)-3,5-difluorophenyl]butadiyne}. J. Fluorine Chem. 1996;7:83–85.
- Masse CE, Vander Wiede K, Kim WH, et al. Novel unsymmetrical polydiacetylene as materials for second-and third-order nonlinear optics. Chem. Mater. 1995;7:904–908.10.1021/cm00053a015
- Kajzar K, Messier J. Resonance enhancement in cubic susceptibility of Langmuir–Blodgett multilayers of polydiacetylene. Thin Solid Films. 1985;132:11–19.10.1016/0040-6090(85)90451-1
- Carreon MP, Burillo G, Ogawa T, et al. Third order nonlinear optical susceptibility of Langmuir–Blodgett membranes of some aromatic diacetylenes determined by Z-scan technique. Mol. Cryst. Liq. Cryst. Sci. Tech. B: Nonlinear Opt. 2000;24:27–33.
- Beristain MF, Muñoz E, Fomine F, et al. Thermal reactions of aromatic diacetylenes: an insight to amorphous state polymerization of diacetylenes. Mol. Cryst. Liq. Cryst. 2006;447:251–263.
- Beristain MF, Muñoz E, Ogawa T, et al. Polymerization of diphenylbutadiyne by gamma ray irradiation in the molten state. Mol. Cryst. Liq. Cryst. 2010;521:237–245.10.1080/15421401003716130
- Beckham HW, Rubner MF. Synthesis and optical properties of a new class of polyamides containing reactive diacetylene group. Macromolecules. 1989;22:2130–2138.10.1021/ma00195a022
- Ogawa T. Diacetylenes in polymeric systems. Prog. Polym. Sci. 1995;20:943–985.10.1016/0079-6700(95)00013-6
- Carreon MP, Fomina L, Fomine S, et al. Third-order nonlinear susceptibility of polydiacetylene-containing polymeric systems. In: Jenekhe SA, Wynne KJ, editors. Photonic and optoelectronic polymers (ACS Symposium Series 672). Washington, DC: American Chemical Society; 1997. Chapter 14, p. 199–216.
- Badarau C, Wang ZY. Synthesis and optical properties of thermally and photochemically cross-linkable diacetylene-containing polymers. Macromolecules. 2004;37:147–153.10.1021/ma035566x
- Yu X, Lou YD, Yan Q, et al. Synthesis and properties of thermally cross-linkable main chain azobenzene polymers containind diacetylene moieties. Eur. Polym. J. 2008;44:881–888.10.1016/j.eurpolymj.2007.12.003
- Fomina L, Fomine S, Ogawa T. Studies on diacetylenic vinyl compounds. VII. Synthesis and polymerization of alka-3-5-dinylmethacrylates and 1,8-bis(methacryloxy)octa-3,5-diyne. Polym. Bull. 1995;34:547–554.10.1007/BF00423350
- Castañon SL, Beristain MF, Ortega A, et al. Synthesis, characterization and third-order nonlinear optical property of poly(2,5-dipropargyloxybenzoate) containing a polar aromatic diacetylene. Dyes Pigm. 2011;88:129–134.10.1016/j.dyepig.2010.05.012
- Cross TA, Davis MC. Synthesis of hydroxyalkyl-substituted, push–pull chromophores based on diphenylacetylenes and 1,4-bis(phenylethynyl) benzenes. Synth. Commun. 2008;38:499–516.10.1080/00397910701796584
- Chang T, Kahn AH. Standard reference materials: electron paramagnetic resonance intensity standard: SRM-2601; Description and Use, NBS Spec. Publ. 260–59. Washington, DC: National Bureau of Standards; 1978.
- Beristain MF, Fomine S, Muñoz E, et al. UV-irradiated formation of diradicals of diphenylbutadiyne and some of its p,p′-disubstituted derivatives. Bull. Chem. Soc. Jpn. 2005;78:1986–1993.10.1246/bcsj.78.1986
- Stiegman AE, Miskowski VM, Perry JW, et al. A series of donor–acceptor molecules of the form NH2(C6H4)(C≡C)n(C6H4)NO2. Unusual effects of varying n. J. Am. Chem. Soc. 1987;109:5884–588610.1021/ja00253a070
- Comoretto D, Ottonelli M, Musso GF, et al. Photoinduced absorption spectra in polydiacetylenes for non linear optical applications. Synth. Met. 2003;138:75–78.10.1016/S0379-6779(02)01272-9