ABSTRACT
Hydrolytic and enzymatic degradation of resin adhesives over time has been mainly attributed to secondary caries formation of methacrylate-based tooth-colored resin-based composite restorations. Ability of resin adhesive monomers to infiltrate into demineralized dentin forming stiff polymer matrix and potentially bonding to tooth structure is also a crucial property. The only commercially available antibacterial monomer, 12-methacryloyloxydodecyl pyridinium bromide (MDPB), is a quaternary ammonium methacrylate. This methacrylate monomer undergoes hydrolytic degradation, and could not bond to tooth structure. In this study, a new hydrolytic resistant monomer HMTAF was synthesized. It is methacrylamide-based monomer that, unlike methacrylate, is highly resistant to hydrolysis. Its molecular structure has particular functional groups; quaternary ammonium fluoride salt with potential antibacterial fluoride-releasing activity, hydroxyl and amide group with hydrogen bonding potential to dentin collagen. Hydroxyl group also increases monomer hydrophilicity for better penetration into water-saturated dentin and sufficient resin-dentin bond. The synthesized HMTAF and its polymer showed no hydrolytic degradation in acidic environment, while MDPB and its polymer were partially decomposed under this challenge. The conversion of monomer HMTAF to polymer was illustrated by FT-IR. The results indicated that HMTAF is highly resistant to hydrolysis, polymerizable and non-cytotoxic to Vero cell lines. It is a potential monomer to be incorporated into resin adhesives for improving hydrolytic and enzymatic resistance.
Introduction
Tooth-colored resin-based composite has been increasingly used in clinical practice due to esthetic concerns and the phase down of amalgam because of its toxicity. However, this material becomes defective and requires replacement nearly twice as often as amalgam [Citation1,Citation2]. Moreover, the cost for placing resin-based composite is about 1.5 times that of placing amalgam. Since the phase down of amalgam could not be refused, improving the longevity of resin-based composite restorations is essential. Three-step etch-and-rinse adhesive system remains the gold standard for bonding resin-based composite restorative materials to tooth structure [Citation3]. The three steps consist of the application of an etchant, primer, and bonding agent, respectively (). While bonding to enamel is effective, bonding to dentin is compromised due to collagen and moisture rich nature of dentin. After etching with 35–37% phosphoric acid and water rinsing, inorganic part of dentin is demineralized leaving water-saturated collagen matrix for subsequently bonding. A primer is then applied into the collagen matrix followed by light air-blow. Solvents in primers accelerate water evaporation from the collagen matrix during the air-blow, while monomers in primers penetrate in-between collagen fibrils. Last, a thin layer of bonding is applied to cover all cavity walls and light cured using visible blue light. Upon light curing, monomers in a bonding are co-polymerized with monomers in a primer providing micromechanical lock to the tooth structure before placing a resin composite [Citation4]. Ideally, monomers in primers should be relatively hydrophilic for efficient infiltration into water-saturated dentin and able to bond to hydroxyapatite or collagen in dentin. Monomers in a bonding should be relatively hydrophobic and have cross-linking potential for strong and durable bond to tooth structure.
Previous studies reported that the failure of resin-based composite restoration was mainly due to the marginal leakage at the resin-tooth interface resulting in secondary caries formation [Citation5,Citation6]. The average lifetime of amalgam is about 8.7 years, while the lifetime of resin adhesive is only about 5 years [Citation2]. Hydrolytic and enzymatic degradation of ester bond of resin adhesives and lacking of their antibacterial property are main causes of short lifetime of resin-based composite [Citation7]. Esterase is a hydrolase enzyme produced by cariogenic bacteria that breaks down the ester bonds in methacrylate monomers. As a consequence, hydrolytic resistance, antibacterial property, and ability of monomers to infiltrate into collagen matrix and form stiff and durable resin matrix are required in order to extend longevity of resin-based composite restorations. To prevent hydrolytic degradation, relatively hydrophobic monomers, such as Bis-GMA and TEGDMA, are preferred over hydrophilic monomers, such as HEMA. However, hydrophobic monomers are not efficiently infiltrated into hydrophilic demineralized collagen matrix. Consequently, hydrophilic monomers are still incorporated in the primer in order to facilitate monomer infiltration[Citation8]. Commonly used resin adhesives are acrylates, usually methacrylates, which can be hydrolyzed in aqueous environment of the oral cavity [Citation9]. For example, hydrolysis of HEMA provides methacrylic acid and ethylene glycol, and combined hydrolytic and enzymatic degradation of Bis-GMA affords bisphenol A which is harmful for the reproductive system () [Citation10]. There was a report that unpolymerized HEMA may cause allergy [Citation11]. Nevertheless, Bis-GMA and HEMA are still popularly used. Bis-GMA forms stiff resin matrix and HEMA is biocompatible and greatly soluble in ethanol and acetone [Citation12].
Quaternary ammonium compounds (QACs) are broad-spectrum antibacterial agents with low toxicity [Citation13,Citation14]. They have a wide variety of applications including incorporation in resin adhesives to prevent secondary caries. 12-Methacryloyloxydodecyl pyridinium bromide (MDPB) () is the only antimicrobial monomer being used in a commercial resin adhesive (Clearfil Protect Bond, Kuraray Co., Ltd., Tokyo, Japan). MDPB is a quaternary ammonium monomethacrylate with positively charged pyridinium group [Citation15]. The positive charges could attract the negative charges of bacterial cell wall resulting in cell lysis. However, this monomer still undergoes hydrolysis. Significant reduction of resin-dentin microtensile bond strength after in vitro 12-month storage was observed [Citation16]. Therefore, there are still areas to explore new antimicrobial monomers that can effectively bond to tooth structure and withstand hydrolytic and enzymatic degradation for durable bond. Quaternary ammonium methacrylate monomers were synthesized and tested for their antibactierial and mechanical properties, such as N, N-dimethylaminohexylmethacrylate (DMAHM) and dimethylaminododecylmethacrylate (DMADDM) () [Citation17]. There are a few literature precedents on (meth)acrylamide-based resin adhesives, mostly without antibacterial moiety, such as 5-NMSA (N-methacryloyl-5-aminosalicylic acid) [Citation18] and DEBAAP (N, N’-diethyl-1,3-bis(acrylamido)-propane) [Citation9] (). 5-NMSA has salicylic acid functional group for binding to calcium, and amide bond which can form hydrogen bonding with amides of collagen. In 2012, Xu and coworkers reported antibacterial fluoride-releasing methacrylamide monomer containing quaternary ammonium group (monomer I) () [Citation19]. However, quaternary ammonium (meth)acrylamide with specific functionalities designed for dentin primers has not widely been synthesized. The objectives of this work were to synthesize and characterize a new quaternary ammonium fluoride methacrylamide-based monomer, HMTAF, containing hydrophilic hydroxyl and amide groups for hydrogen bonding to dentin collagen. Hydroxyl group also increases monomer hydrophilicity for better penetration into water-saturated dentin and sufficient resin-dentin bond. The desirable properties of hydrolytic resistance, polymerization capability of methacrylamide group and cytotoxicity of the monomer were also proved.
Experimental
General information
Unless otherwise stated, all reactions were performed under a nitrogen atmosphere in oven- or flamed-dried glassware. Please see synthesis and characterization data of each compound in Supplementary Data). Solvents were used as received from suppliers or distilled prior to use following standard procedures. All other reagents were obtained from commercial sources and used without further purification. Column chromatography was performed on SiliaFlash® G60 Silica (60–200 μm, Silicycle). Thin-layer chromatography (TLC) was performed on SiliaPlateTM R10011B-323 (Silicycle) or Silica gel 60 F254 (Merck). H1, and C13 NMR spectroscopic data were recorded on a 300 MHz Bruker FT-NMR Ultra Shield spectrometer using tetramethylsilane as an internal standard. Infrared (IR) spectra were recorded on a Perkin Elmer 783 FTS165 FT-IR spectrometer (Bruker Corp., Billerica, MA, USA). High-resolution ESI mass spectra were obtained on a liquid chromatograph-mass spectrometer (2690, LCT, Waters, Micromass). Gel permeation chromatography (GPC) was performed using an Agilent Technologies 1260 Infinity II (for MDPB polymers) or PL-GPC 220 (for HMTAF polymers) equipped with two PLgel 10 μm MIXED-B columns (Agilent, for MDPB polymers) or two PLgel 5 μm MIXED-C columns (Agilent, for HMTAF polymers) and a guard column (Agilent). Tetrahydrofuran (THF) was used as the eluent at a flow rate of 1 mL/min at 40 °C. A refractive index detector was used and the molecular weights were calibrated against twelve linear polystyrene standards ranging from 162 to 364,000 (Mp[g/mol]).
Study of hydrolytic stability of HMTAF, MDPB and their polymers
First, 1 mL of 0.1 molar hydrochloric acid (pH = 1) was added to a solution of 10 mg HMTAF, MDPB or their polymers in 50 µL methanol. The resulting solutions were then stored at room temperature for 7 days. After that, methanol was evaporated under reduce pressure, and water was removed by freeze-drying process [Citation1]. H NMR spectra of hydrolyzed HMTAF and MDPB were observed. GPC traces of HMTAF and MDPB polymers before and after hydrolysis were collected.
Photopolymerization of HMTAF and MDPB
50 mg of HMTAF or MDPB was mixed with photoinitiators: 0.25% camphoquinone and 1% ethyl 4-(dimethylamino)benzoate (EDMAB) in methanol, and dried with a gentle stream of air. The thin film was irradiated with a halogen lamp (Valo LED curing, 1300 mW/cm2, Ultradent Products Inc., St Louis, MO, USA) for 15 min. FT-IR spectra of HMTAF before and after photopolymerization were observed.
Cytotoxicity assays
The non-cancerous monkey kidney fibroblast Vero cell lines (ATCC® CCL-81™) were seeded onto 96-well culture plate at a density of 2.5 × 103 cells/well and incubated overnight at 37 °C in humidified atmosphere containing 5% carbon dioxide. Cells were exposed to 0–200 µM of HMTAF in 0.2% v/v DMSO for 72 h. Doxorubicin treatment was also performed as a positive control. Cytotoxicity of HMTAF from triplicate experiments (N = 9) was determined by MTT assay as method reported in Iawsipo et al [Citation20]. Plots of % cell viability vs concentration of HMTAF and doxorubicin were observed.
Results and discussion
Resin adhesive monomer HMTAF was synthesized in 7 steps as shown in . At first, epoxide ring opening of epichlorohydrin was performed using benzaldehyde and ammonium hydroxide, followed by hydrolysis with hydrochloric acid to give compound 1[Citation21]. Then amino group of compound 1 was protected with tert-butyl carbamate (Boc) to provide 78% of compound 2 over 2 steps. Chlorinated compound 2 was converted to iodinated compound 3 in 70% using sodium iodide. Next, compound 3 was transformed to amino compound 4 by ammonium hydroxide in quantitative yield. Methacrylamide 5 was constructed in 95% yield by reacting amine 4 with methacrylic anhydride. Boc protecting group was removed by concentrated hydrochloric acid, followed by methylation with methyl iodide to provide quaternary ammonium iodide salt HMTAI in 99% yield. Finally, iodide ion in HMTAI was exchanged with fluoride ion from silver fluoride to yield HMTAF in 59%. The overall yield of synthesizing HMTAF was 30%. Synthesis of the reference monomer, MDPB, the only commercial antibacterial resin adhesive, was performed as shown in . Condensation of 12-bromoundecanol with methacrylic acid and catalytic sulfuric acid was carried out to afford methacrylate 6 in 94%. Substitution of compound 6 with pyridine afforded MDPB in 68%.
In order to study the hydrolytic resistance of synthesized monomers, HMTAF and the reference monomer, MDPB, were individually subjected to an acidic aqueous solution of hydrochloric acid (0.1 M, pH = 1) for a week. H1 NMR spectra of HMTAF before and after treating with the acid were identical indicating the high stability of monomer HMTAF in an acidic environment. In other words, HMTAF is a hydrolytic resistant monomer. In contrast, H1 NMR spectra of MDPB after acidic treatment showed extra peaks of methacrylic acid at 6.17 and 5.61 ppm () resulting from hydrolysis of ester bond of MDPB into methacrylic acid and pyridinium bromide salt 7 as shown in . It can be concluded from this experiment that MDPB underwent hydrolytic degradation faster than HMTAF in an acidic aqueous environment. Exposure of monomers in an acidic aqueous solution exaggerates aging condition via hydrolysis in the oral environment where resin adhesives are periodically exposed to acids from foods and cariogenic bacteria [Citation4,Citation22]. Instability of MDPB in a long-term water storage has been well studied by De Munck et al [Citation23].
Figure 3. Citation1H NMR spectra of MDPB (a) before and (b) after treatment with aqueous hydrochloric acid (0.1 M, pH = 1).
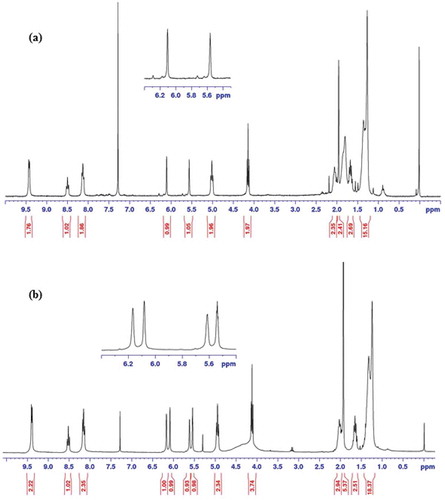
After blending HMTAF with photoinitiators: camphoquinone and ethyl 4-(dimethylamino)benzoate (EDMAB), and curing with a halogen lamp (blue light), conversion of monomer HMTAF into polymer was indicated by significant decrease of intensity of characteristic peak of carbon-carbon double bond at 1537 cm−1 in IR spectra as shown in [Citation24]. As a result, monomer HMTAF is able to polymerize under the blue light which is used in dental clinics. GPC data of HMTAF polymer showed Mw = 8468 g/mol, Mn = 3249 g/mol and PDI = 2.61. Polymers of HMTAF and MDPB were also subjected to hydrolysis in the same condition as above. After hydrolysis, GPC data showing Mw = 8744 g/mol, Mn = 3226 g/mol and PDI = 2.71 indicated that HMTAF polymer did not hydrolyzed in acidic solution within a week. MDPB polymer before hydrolysis gave Mw = 51,881 g/mol, Mn = 25,667 g/mol and PDI = 2.02, but it was partially degraded into oligomers under this condition, Mw = 37,973 g/mol, Mn = 22,171 g/mol, PDI = 1.71 (see all GPC traces in supplementary data).
Figure 4. FT-IR spectra of HMTAF (a) before and (b) after photopolymerization by curing with a halogen lamp.
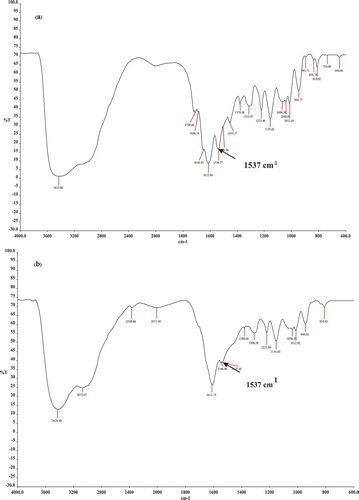
HMTAF was non-cytotoxic against Vero cells (non-cancerous African green monkey Cercopithecus aethiops kidney fibroblast cell lines). It did not show significant inhibitory effect on Vero growth even at high concentration of 200 µM, since 90% of Vero cells were viable as shown in , whereas the positive control, doxorubicin showed IC50 value at 1.5 µM (see supplementary data).
Conclusions
New resin adhesive monomer, HMTAF, was successfully synthesized in 30% overall yield. The methacrylamide HMTAF and its polymer were stable under an acidic solution whereas the commercial antibacterial methacrylate MDPB and its polymer were partially hydrolyzed under the same condition. HMTAF could be polymerized by curing with a halogen lamp. It was non-cytotoxic to Vero cell lines. Antibacterial properties of HMTAF against Streptococcus mutans and Enterococcus faecalis, associated with caries formation, will be further investigated. HMTAF is a potential monomer to be incorporated into resin adhesives for improving resin-dentin bond durability through hydrolytic resistance in the oral environment and antibacterial activity. The synthesis of HMTAF could be improved using a shorter process to enhance the overall yield. Moreover, the phosphate group maybe incorporated into the molecule for bonding to hydroxyapatite in dentin.
Supplemental Material
Download PDF (1.2 MB)Disclosure statement
No potential conflict of interest was reported by the authors.
Supplementary material
Supplementary data for this article can be accessed here
Additional information
Funding
References
- Tyas MJ. Placement and replacement of restorations by selected practitioners. Aust Dent J. 2005;50:81–89.
- Rho YJ, Namgung C, Jin BH, et al. Longevity of direct restorations in stress-bearing posterior cavities: a retrospective study. Oper Dent. 2013;38:572–582.
- Peumans M, De Munck J, Van Landuyt KL, et al. A 13-year clinical evaluation of two three-step etch-and-rinse adhesives in non-carious class-V lesions. Clin Oral Investig. 2012;16:129–137.
- Bourbia M, Finer Y. Biochemical stability and interactions of dental resin composite and adhesives with host and bacteria in the oral cavity: a review. J Can Dent Assoc. 2018;84:1–7.
- Demarco FF, Correa MB, Cenci MS, et al. Longevity of posterior composite restoration. Dent Mater. 2012;93:87–101.
- Opdam NJ, van de Dande FH, Bronkhorst E, et al. Longevity of posterior restorations: a systematic review and meta-analysis. J Dent Res. 2014;93:943–949.
- Breschi L, Maravic T, Cunha SR, et al. Dentin bonding systems: from dentin collagen structure to bond preservation and clinical applications. Dent Mater. 2018;34:78–96.
- Pashley DH, Tay FR, Breschi L, et al. State of the art etch-and-rinse adhesives. Dent Mater. 2011;27:1–16.
- Salz U, Zimmerman J, Zeuner F, et al. Hydrolytic stability of self-etching adhesive systems. J Adhes Dent. 2005;7:107–116.
- Al Hiyasat AS, Darmani H, Elbetieha AM. Effects of bisphenol A on adult male mouse fertility. Eur J Oral Sci. 2002;110:163–167.
- Paranjpe A, Bordador LC, Wang MY, et al. Resin monomer 2-hydroxyethyl methacrylate (HEMA) is a potent inducer of apoptotic cell death in human and mouse cells. J Dent Res. 2005;84:172–177.
- Geurtsen W. Biocompatibility of resin-modified filling materials. Crit Rev Oral Biol Med. 2000;11:333–355.
- Antonucci JM, Zeiger DN, Tang K, et al. Synthesis and characterization of dimethacrylate containing quaternary ammonium functionalities for dental application. Dent Mater. 2012;28:219–228.
- Gerba CP. Quaternary ammonium biocides: efficacy in application. Appl Environ Microbiol. 2015;81:464–469.
- Imazato S, Russell RR, McCabe JF. Antibacterial activity of MDPB polymer incorporated in dental resin. J Dent. 1995;23:177–181.
- Pupo YM, Farago PV, Nadal JM, et al. Effect of a novel quaternary ammonium methacrylate polymer (QAMP) on adhesion and antibacterial properties of dental adhesives. Int J Mol Sci. 2014;15:8998–9015.
- Cocco AR, Rosa WL, Silva AF, et al. A systematic review about antibacterial monomers used in dental adhesive systems: current status and further prospects. Dent Mater. 2015;31:1345–1362.
- Nishiyama N, Suzuki K, Asakura T, et al. Adhesion of N-methacryloyl-ω-amino acid primers to collagen analyzed by 13C NMR. J Dent Res. 2001;80:855–859.
- Xu X, Wang Y, Liao S, et al. Synthesis and characterization of antibacterial dental monomers and composites. J Biomed Mater Res Part B. 2012;100:1151–1162.
- Panata I, Ekaruth S, Mathurose P, et al. Cytotoxic effects of Etlingera pavieana rhizome on various cancer cells and identification of a potential anti-tumor component. J Food Biochem. 2018;42:e12540.
- Perrault WR, Pearlman BA, Godrej DB, et al. The synthesis of N-aryl-5(S)-aminomethyl-2-oxazolidinone antibacterials and derivatives in one step from aryl carbamates. Org Proc Res Dev. 2003;7:533–546.
- Pinna R, Usai P, Filigheddu E, et al. The role of adhesive materials and oral biofilm in the failure of adhesive resin restorations. Am J Dent. 2017;30:285–292.
- De Munck J, Mine A, Vivan Cardoso M, et al. Effect of dentin location and long-term water storage on bonding effectiveness of dentin adhesives. Dent MaterJ. 2011;30:7–13.
- Nakano Y, Shinke K, Ueno K, et al. Solid polymer electrolytes prepared from poly(methacrylamide) derivative having tris(cyanoethoxymethyl) group as its side chain. Solid State Ion. 2016;286:1–6.