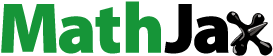
ABSTRACT
Implanted medical devices that have poor friction property or biofilm formation can limit their service life and cause discomfort in patients. Recently, some zwitterionic coatings have been studied to modify the biomaterials surface for lubricating function, but the grafting methods of coatings are complicated and also seldom take the bacterial antiadhesion property into account at the same time. In our studies, motivated by the properties of nature mussels and human articular, we firstly successfully synthesized double adhesion protection of self-adhesive ternary polymer coating and achieved the excellent lubrication and antifouling functionalization of the medical devices surface. In details, the X-ray photoelectron spectroscopy, scanning electron microscope and the water contact angles could characterize the successful modification on the surface of titanium substrate. Additionally, the tribological tests carried out by atomic force microscope verified the ternary polymer could enhance the lubrication property owing to the hydration lubrication mechanism. Meanwhile, it also possessed the bacterial antiadhesion property for the initial 24 h attributed to the hydration repulsive force. We believe that, as a simple and universal preparation method, the ternary polymer could make a great significance for improving the surface function of biomaterials and alleviating patients’ discomfort.
1. Introduction
Some implantable medical devices are prone to tissue damage due to the frictional phenomena [Citation1–3] and complications such as bacterial biofilm pollution [Citation4] and thrombosis [Citation5], which may cause discomfort to the patient and seriously limit their use. In addition, studies have shown that these complications are closely related to the fouling adhesion of some proteins or bacteria [Citation6,Citation7]. Therefore, the surface lubrication and antifouling modification are urgently needed for implantable medical devices. Surface modification is one of the most effective methods to improve their lubrication or antifouling properties.
Currently, inspired by the super-lubrication properties of articular cartilage, zwitterionic polymer coatings especially 2-methacryloxyethyl phosphorylcholine (MPC) have been extensively studied based on the hydration lubrication mechanism [Citation8–11]. However, the poor adhesion of coating and cumbersome synthesis methods limit the clinical applications of the most materials. Besides, there are studies using silver nanoparticles for antibacterial function [Citation12,Citation13], but they are generally biologically toxic. And as far as we know, the combination of lubrication property with antifouling property for general coatings has not been well studied. Therefore, it is very important to develop a mild and universal surface modification method to prepare coatings that consider both lubrication and antifouling properties on the surface of medical materials.
The mussel organisms can firmly attach to the surface of many objects through the adhesion proteins secreted by their feet [Citation14,Citation15]. Dopamine is an important derivative of the mussel adhesion protein, which has a similar adhesion function to mussels. The catechol structure in dopamine can be oxidized to quinone under neutral or weakly alkaline conditions, and further react with the active hydrogen of hydroxyl to form strong chemical bonds [Citation16–18]. There have been many reports in recent years using dopamine to modify the surface of materials due to the relatively mild grafting method [Citation16,Citation19–21].
Based on this, inspired by the super adhesion of mussels and the super lubrication of articular cartilage, and also utilized the common coating resin monomer to enhance adhesion for double adhesion protection, the biomimetic self-adhesion ternary polymer coating DMA-MEMA-MPC was fabricated by free radical copolymerization and dip coating method, as the illustration shown in . The titanium that is commonly used in implantable medical devices was used as the substrate, and the lubrication and antifouling properties of the coating were further characterized. As a simple and universal preparation method, the ternary polymer could make a great significance for improving the surface function of implantable medical devices and alleviating patients’ discomfort.
2. Experimental sections
2.1. Materials and reagents
Pure titanium sheet (Ti) was commercially available in Zhonghui Metal Materials Co., China. Dopamine methacrylamide (DMA) was purchased from Ziyuan Biotechnology Co., Ltd., China. 2-Methacryloxyethyl phosphorylcholine (MPC) was purchased from Sigma-Aldrich, Inc., USA. 2-Methoxyethyl methacrylate (MEMA) and Azodiisobutyronitrile (AIBN) were purchased by Aladdin Bio-Chem Technology Co., Ltd., China. Tris-HCl buffer (pH 8.5) and phosphate buffer solution (PBS, pH 7.2) were purchased from J&K Scientific Ltd., China.
2.2. Synthesis of DMA-MEMA-MPC ternary polymer
The ternary polymer DMA-MEMA-MPC was synthesized by conventional free radical copolymerization with the AIBN as the initiator. Briefly, 0.2 g (0.9 mmol) of DMA, 0.2 g (1.4 mmol) of MEMA, 0.8 g (3.1 mmol) of MPC and 3 mg (0.02 mmol) of initiator AIBN were placed in a 250 mL flask, and 50 mL of N, N-Dimethylformamide (DMF) was added for dissolution. The initial molar ratio of the DMA, MEMA and MPC reactants is 1:1.5:3.4. The N2 atmosphere was created during the experiment, and magnetic stirring until the monomers were dissolved. Then the system was heated to 55°C for polymerization and stirred continuously for 24 h in N2 atmosphere. After the reaction was finished, the system was naturally cooled to room temperature, and the dialysis bag with a molecular weight of 8000 D was used to dialysis the products for 2 days. After the dialysis, the products were freeze-dried for 2 days.
2.3. Fabrication of DMA-MEMA-MPC coated titanium
The surface modification of titanium substrates was carried out by gently dip coating process, the pure titanium sheets need to be chemically mechanically polished and then cleaned by ultrasonication in deionized water and ethanol for 5 min. 10 mg/mL of DMA-MEMA-MPC ternary polymer was dissolved in 1 M Tris-HCl buffer (pH 8.5) by ultrasonication, then the titanium sheets were immersed in the ternary polymer solutions in the dark for 12 h. Soon afterwards, several substrates were gently rinsed with deionized water and dried in oven, and stored in a dry environment at room temperature for the subsequent experiments. The DMA-MEMA-MPC ternary polymer grafted titanium is expressed in Terpolymer-g-Ti later.
2.4. Characterizations of ternary polymer and Terpolymer-g-Ti
The DMA-MEMA-MPC was firstly characterized by 1H-nuclear magnetic resonance spectra (1H-NMR, JNM-ECZ600R/S1, JEOL, Japan), with D2O as the deuterium solvent. The molecular weight (Mw) of DMA-MEMA-MPC was characterized by gel permeation chromatography (GPC, Viscotek TDA305max, Malvern Instruments, UK) using water as the diluent (flow rate: 0.7 mL/min). The elements of titanium and Terpolymer-g-Ti were recorded by X-ray photoelectron spectroscopy (XPS, ESCALAB Xi+, ThermoFisher Scientific, UK). The surface topography of titanium and Terpolymer-g-Ti was investigated by scanning electron microscope (SEM, Regulus 8100, Hitachi, Japan) in 5 kV, and sputtered coated with gold in advance. Finally, the change of hydrophilicity was characterized by the water contact angles via static contact angle goniometer (DCA315, Thermo Cahn, USA).
A quartz crystal microbalance (QCM, QCM922A, Princeton Applied Research, USA) was used for evaluation of terpolymer adhesion property. The Ti sensor was placed into the flow cell and sealed. Firstly, the Tris-HCl buffer was pumped over the Ti sensor by peristaltic pump in 1 mL/min, and stabilized for nearly 10 min to obtain the baseline. Then the 10 mg/mL of terpolymer in Tris-HCl buffer solution was pumped over the Ti sensor for 50 min for adhesion. Subsequently, the Ti sensor was washed by Tris-HCl buffer for additional 20 min until stable. The adhesion Sauerbrey mass (Sm) of the terpolymers on the Ti sensors was calculated by the Sauerbrey equation [Citation22], where C was the mass sensitivity (C = 4.42 ng/(cm2∙Hz) for the 9 MHz sensor), ΔF was the frequency change of the sensor (negative value), and n was the overtone number (n = 1 in this test).
2.5. Tribological tests
The lubrication of titanium sheet before and after modification was investigated. The tribological tests were performed by atomic force microscope (AFM, Dimension ICON, Bruker, Germany) under contact mode, with a scan rate of 2 Hz and a scan area of 10 μm × 10 μm, and carried out with a load of 100, 200, 300 and 400 nN, added PBS as the lubricating medium. The tribological tests were carried out in ball-on-disk mode, using the silicon cantilever with a silicon dioxide (SiO2) microspheres in the jut (TL-CONT, Nano World, Switzerland) as the upper sample and titanium and Terpolymer-g-Ti as the lower sample, respectively. The average coefficient of friction (COF) values were calculated from at least three measurements, to ensure the validity.
2.6. Bacterial antiadhesion tests
Escherichia coli (E. coli, ATCC8739, Morey Biosciences, Inc., China) was used as materials for antibacterial experiments. Firstly, the E. coli suspension in Luria-Bertani (LB, Sigma-Aldrich, USA) was diluted to 1 × 108 CFU/mL. Two groups Ti and Terpolymer-g-Ti, six samples each group were placed in 12-well plate and incubated with 2 mL of fresh E. coli suspension in the shaker at 37°C, 100 rpm for 24 h. Afterwards, the samples were removed from the E. coli suspension and washed with water. Three samples of each group were fixed with 2.5% paraformaldehyde overnight and dehydrated by several ethanol solutions (20%, 40%, 60%, 80% and 100%). Subsequently, the samples were investigated by SEM to examine the antibacterial properties, and sputtered the samples coated with gold in advance. Meanwhile, the other three samples in each group were placed in several centrifuge tubes and added 2 mL fresh LB solution. The bacteria adhering to the substrate were separated into solution by vortex oscillation. Finally, the antifouling property, namely bacterial resistance ratio of Ti and Terpolymer-g-Ti were quantitatively calculated by the spread plate methods. Briefly, 200 μL of the above bacterial LB solutions was inoculated onto the solid agar plates in parallel and incubated at 37°C for 24 h. The bacterial resistance ratio of Ti and Terpolymer-g-Ti was calculated by the following formula, where and
were the bacterial counts of Ti and Terpolymer-g-Ti groups, respectively.
3. Results and discussion
3.1. Characterization of DMA-MEMA-MPC
The freeze-dried product is relatively a pink solid, with a yield of about 70%. To be sure, the ternary polymer needs to be ensured that was prepared successfully. Firstly, the 1H NMR spectrum of DMA-MEMA-MPC product is displayed in ). The δ signal at 6.87 ppm correspond to the characteristic group of DMA, the δ signal at 3.71 is assigned to MEMA and the δ signal at 3.13 ppm is attributable to MPC [Citation17]. However, the molar ratio of the DMA, MEMA and MPC in the final polymer product is 1:0.7:1.7, the obtained MEMA and MPC monomer ratios are less than half of the actual feed ratio observed by the integrations, which indicates that the double bonds of DMA have the relatively higher polymerization activity due to the relatively stronger electron withdrawing ability of amide group. Additionally, ) shows the GPC results of the ternary polymer, in which the Mw is 1.045E6 Da with the polydispersity of 2.91. In addition, the tailing phenomenon of GPC peaks may be due to the presence of polar groups or the absorption of the dopamine group on the analysis gel-column. Anyhow, these results indicate that the terpolymer has been successfully synthesized.
3.2. Characterization of Terpolymer-g-Ti
Another important process to be determined is that the ternary polymer was successfully modified onto the titanium substrate. The XPS results present the element compositions of the different samples are shown in ). Obviously, the new inclusion of the peaks with the binding energy at 400 eV, 189 eV and 130 eV indicate the N 1s, P 2s, and P 2p in the DMA-MEMA-MPC, compared to bare titanium substrate. Additionally, the related narrow spectrums in XPS are also provided in ), which corresponds to the -PO4−, -N+(CH3)3 and -NHCO- groups of the DMA-MEMA-MPC polymer, respectively. Meanwhile, the area of the two assigned peaks of N 1s (-N+(CH3)3 for MPC, -NHCO- for DMA) can prove that the proportion of the MPC in the terpolymer product is less than the actual feeding ratio, which is consistent with the results of NMR integration. Before and after coating, the surface morphology of the titanium substrate is not much different, and the surface is still smooth after coating modification, as shown in the SEM results in ). Moreover, the water contact angles of titanium substrate and Terpolymer-g-Ti are 87.68° and 38.03° respectively, which can act as a further evidence that indicating the successful modification of titanium substrate by the terpolymer. Actually, the excellent hydrophilicity is attributed to the hydrophilic functional groups of phosphorylcholine groups (-N+(CH3)3 and -PO4−) in MPC, which have the enhanced hydration layer [Citation8]. QCM was used for the quantitative evaluation of terpolymer self-adhesion property, and ΔF of the Ti sensor can reflect the change in the adhesion Sm. As shown in ), the terpolymers are continuously adsorbed on the sensor surface as time went on, although Tris-HCL buffer can wash out some weakly adsorbed polymer molecules, and the adhesion Sm of the terpolymer is 353.6 ng/cm2 within 50 min. What’s more, the assembled terpolymer coating was finally washed by Tris-HCl buffer for additional 20 min until stable, which can characterize the stability of the terpolymer coating from the side to some extent.
Figure 3. Material characterization proves that the ternary polymer was successfully modified onto the titanium substrate. (a) The XPS (contain the narrow spectrums) results present the element compositions of the different samples. (b) The surface morphology in SEM and (c) the water contact angles for the titanium substrate and Terpolymer-g-Ti substrate. (d) QCM experiment proves the self-adhesion ability and stability of the terpolymer on Ti sensor
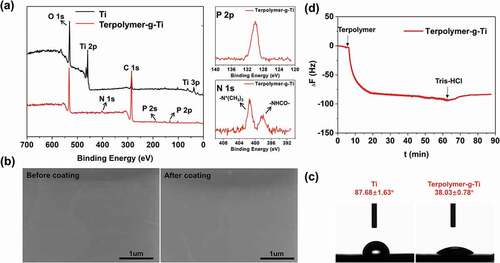
3.3. Lubrication performance
) shows the schematic illustration of the tribological tests, using ball-on-disk mode, the SiO2 as the upper sample and titanium and Terpolymer-g-Ti as the lower sample. The comparison of COF for the different substrates under different normal loads is displayed in ). It can be seen that the COF is decreased dramatically from 0.131 to 0.077 (~41%) after coating compared with the bare titanium, which is owing to the excellent hydration lubrication layer of the MPC on the titanium surface. Additionally, the results indicate that the load seems to have little effect on COF for the bare titanium, while the COF of Terpolymer-g-Ti increases with the increase in the load. Generally, the excellent hydration layer is attributed to the hydration lubrication mechanism of the phosphorylcholine groups in MPC, which has been reported by Klein et al. [Citation8]. Briefly, the two zwitterions (-N+(CH3)3 and -PO4−) in phosphorylcholine could attract several water molecules tightly to form an intensified hydration layer around them, which can withstand loads to some extent and can cope in a fast flow state under shear forces conditions and thus bring about a relatively low COF.
3.4. Bacterial antiadhesion property
Finally, the bacterial antifouling properties of the coating were also examined. The production of bacterial biofilm is closely related to the initial adhesion of bacteria. Therefore, the bacterial adhesion of the two kinds of substrates was investigated for the initial 24 h. After co-cultured with the bacterial suspension, it is obviously that the DMA-MEMA-MPC coated substrate has less bacteria, as the SEM results shown in ). However, the titanium substrate before coating suffers from severe bacterial adhesion as shown in ), and it is observed that the surface is dirty in the magnification graph. Similarly, the quantitatively results of bacterial resistance tests by spread plate experiments are consistent with the SEM results, as shown in ). The bacterial colony images of Ti and Terpolymer-g-Ti in ) are very distinct, and the bacterial resistance ratio of Terpolymer-g-Ti is higher than 83%, which are significantly superior to that of Ti group. These results indicate that the terpolymer coating could effectively enhance the bacterial antifouling properties due to the strong hydration layer formed by phosphorylcholine groups, which could generate hydration repulsive force upon bacteria when they initially approach to the surface. Actually, the double adhesion protection of self-adhesive ternary polymer coating may be more attributed to the enhanced stable coatings due to increased anchor points. Moreover, various adhesion components could also assist the construction of the coating, thus exposing more MPC components on the surface.
4. Conclusions
In the present study, a biomimetic self-adhesion ternary polymer was synthesized and the titanium substrate was modified to achieve the excellent lubrication and antifouling properties for the surface of implantable biomedical materials. The excellent lubrication and antifouling properties are attributed to the hydration lubrication mechanism and hydration repulsive force, which owing to the strong hydration layer formed by phosphorylcholine groups in MPC. It can be believed that the simple and universal preparation method of the coating will have a great significance for improving the surface modification of implantable medical devices and alleviating patients’ discomfort.
Disclosure statement
No potential conflict of interest was reported by the author(s).
Additional information
Funding
References
- Mandal SS, Jose D, Bhattacharyya AJ. Role of surface chemistry in modulating drug release kinetics in titania nanotubes. Mater Chem Phys. 2014;147:247–253.
- Cui W, Bian YY, Zeng HK, et al. Structural and tribological characteristics of ultra-low-wear polyethylene as artificial joint materials. J Mech Behav Biomed. 2020;104:103629.
- Al-Mobarak NA, Al-Swayih AA. Development of titanium surgery implants for improving osseointegration through formation of a titanium nanotube layer. Int J Electrochem Sci. 2014;9(1):32–45.
- Zawadzki N, Wang Y, Shao H, et al. Readmission due to infection following total hip and total knee procedures: a retrospective study. Value Health. 2016;19(3):A228–A228.
- Erbel R, Di Mario C, Bartunek J, et al. PROGRESS-AMS (Clinical performance and angiographic results of coronary stenting with absorbable metal stents) investigators. Temporary scaffolding of coronary arteries with bioabsorbable magnesium stents: a prospective, non-randomised multicentre trial. Lancet. 2007;2(369):1869–1875.
- Renvert S, Lindahl C, Renvert H, et al. Clinical and microbiological analysis of subjects treated with Brånemark or AstraTech implants: a 7-year follow-up study. Clin Oral Implants Res. 2008;19(4):342–347.
- Cecile B, Ellison CK, Adrien D, et al. Bacterial adhesion at the single-cell level. Nature Rev Microbiol. 2018;16(10):616–627.
- Klein J. Hydration lubrication. Friction. 2013;1(1):1–23.
- Milner PE, Parkes M, Puetzer JL, et al. A low friction, biphasic and boundary lubricating hydrogel for cartilage replacement. Acta Biomater. 2018;65:102–111.
- Vatankhah-Varnoosfaderani M, Hu X, Li Q, et al. Universal coatings based on zwitterionic-dopamine copolymer microgels. Acs Appl Mater Inter. 2018;10(4):20869–20875.
- Liu G, Liu Z, Li N, et al. Hairy polyelectrolyte brushes-grafted thermosensitive microgels as artificial synovial fluid for simultaneous biomimetic lubrication and arthritis treatment. Acs Appl Mater Interfaces. 2014;6(22):20452–20463.
- Yun’An Q, Lin C, Li R, et al. Potential antibacterial mechanism of silver nanoparticles and the optimization of orthopedic implants by advanced modification technologies. Int J Nanomedicine. 2018;13:3311–3327.
- Wang TW, Zhang F, Zhao R, et al. Polyvinyl alcohol/sodium alginate hydrogels incorporated with silver nanoclusters via green tea extract for antibacterial applications. Des Monomers Polym. 2020;23(1):118–133.
- Lee H, Dellatore SM, Miller WM, et al. Mussel-inspired surface chemistry for multifunctional coatings. Science. 2007;318(5849):426–430.
- Waite JH, Qin X. Polyphosphoprotein from the adhesive pads of mytilus edulis. Biochemistry-Us. 2001;40(9):2887–2893.
- Liu YL, Ai KL, Lu LH. Polydopamine and its derivative materials: synthesis and promising applications in energy, environmental, and biomedical fields. Chem Rev. 2014;114:5057–5115.
- Wang BL, Jin TW, Han YM, et al. Bio-inspired terpolymers containing dopamine, cations and MPC: a versatile platform to construct a recycle antibacterial and antifouling surface. J Mat Chem B. 2015;3(27):5501–5510.
- Lee H, Scherer NF, Messersmith PB. Single-molecule mechanics of mussel adhesion.Proc. Nat Acad Sci. 2006;103(35):12999–13003.
- Lee H, Lee Y, Statz AR, et al. Substrate-independent layer-by-layer assembly by using mussel-adhesive-inspired polymers. Adv Mater. 2010;20(9):1619–1623.
- Wang W, Ji X, Na HB, et al. Design of a multi-dopamine-modified polymer ligand optimally suited for interfacing magnetic nanoparticles with biological systems. Langmuir Acs J Surf Colloids. 2014;30(21):6197–6208.
- Mei Y, Yu K, Lo J, et al. Polymer-nanoparticle interaction as a design principle in the development of a durable ultra-thin universal binary anti-biofilm coating with long-term activity. Acs Nano. 2018;12(12):11881–11891.
- Greene GW, Martin LL, Tabor RF, et al. Lubricin: a versatile, biological anti-adhesive with properties comparable to polyethylene glycol. Biomaterials. 2015;53:127–136.