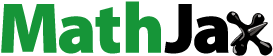
ABSTRACT
In this research article, the new donor–acceptor (D–A) monomers developed using 4-methoxy-9-methyl-9 H-carbazole (MMCB) as electron donors and various electron acceptors. DFT and TD-DFT methods at the level of B3LYP with a 6–311 G basis set in a gas and chloroform solvent were used to calculate electronic and optoelectronic properties. To dissect the relationship between the molecular and optoelectronic structures, the impacts of specific acceptors on the geometry of molecules and optoelectronic properties of these D–A monomers were discussed. The calculations are also carried out on HOMO–LUMO, atomic orbital densities. The calculated band gap Eg of the monomers considered increases 3,6-MMCB-OCP ≈ 3,6-MMCB-BCO < 3,6-MMCB-SDP < 3,6-MMCB-SCP < 3,6-MMCB-TCP < 3,6-MMCB-TDP < 3,6-MMCB-BCS < 3,6-MMCB-BCT in both in the gas and solvent phases. Subsequently, the optoelectrical properties of EHOMO, ELUMO, Eopt, and EB energies were critically updated. Compared to different monomers, the far lower Eg of the 3,6-MMCB-OCP and 3,6-CB-BCO has shown optoelectronic applications in organic solar cells like BHJ.
1. Introduction
Global energy demand continues to increase, and therefore, there is an urgent need both for industry and academia to find new and renewable energy sources. There are renewable and clean-energy technologies, and photovoltaics are very promising among these. In recent years organic photovoltaics (OPV), based mainly on π-conjugated polymers, have been attractive, not to mention that these devices, due to their low production cost, processing and flexibility, are light and practical for indoor and outdoor use [Citation1]. In addition, they display very rapid energy payback time (EPBT) despite their relatively low performance: while the EPBT is in the order of years for silicon-based technologies, it has been calculated for OPV on average [Citation2,Citation3].
In the state-of-the-art bulk heterojunction (BHJ) [Citation4], the active layer of the OPV module consists of a compound from electron-donor and electron-acceptor materials. In most situations, the first is a conjugate polymer and, second, a soluble fullerene derivative [Citation5] (5phenylC61-butyric acid methyl ester, also known as PC61BM or simply PCBM). This network will split the excitons into an electron and a hole and generate free carriers. The absorption of photons in the polymer depends primarily on how many photons the polymer is absorbed. One of the most widely used materials in BHJ was the poly(3-hexylthiophene) (P3HT), photocurrent converting efficiency (PCE), a regio-regular homo-polymer that led to modules that showed a 6% PCE [Citation5,Citation6]. One of the questions of P3HT is its relatively wide bandgap [Citation7] of 2 eV, which prevents the absorption of several photons because it interacts with the specific part of the energy spectrum from solar energy. As a result, P3HT was stated only to capture 20% of solar photons [Citation8]. Various methods were subsequently developed to reduce the difference between such substances or capture photons in long wavelengths to absorb radiation from the sun to the earth [Citation9].
As a consequence, the charge transfers between the donor (D) to accepter (A) units are intramolecular [Citation10]. This effect is because the two different units merge orbitals. The result is a generally small bandgap concerning homo-polymers like P3HT among material and tunable material. Several essential procedures in OPV cells are necessary to optimize light absorption to generate excitons in the active layer and the dissociation of excitons in the donor/acceptor interface (D/A) to generate the free carriers. Levels of neither intermolecular charge (CT) nor recombinant (CR) interface (D/A) transmission in particular strongly affect solar-cell efficiency [Citation10]. Indeed, the decrease of inter-charge transfer (CT) status is expected to increase as quickly as possible as the reaction of the responsible recombination mechanism and short circuit current (JSC) [Citation10].
To be more efficient in polymers, solar cells need to be carefully engineered to adjust frontier molecular orbits (FMO) along the border. The HOMO energy level of a donor polymer must first be reduced to obtain a sizeable open-circuit voltage (VOC). The LUMO energy level of the donor should be at least 0.3 eV above the acceptor LUMO (PCBM) level [Citation11] to ensure a quantitative separation of charge at the D/A interface. Inorganic electronic devices, such as organic solar cells and electrochemical superconductors, that limit energy levels of conjugated polymers are essential for their performance [Citation12]. The HOMO–LUMO energy difference affects the short circuit current (JSC) directly in the organic solar cells.
In contrast, the HOMO energy from the donor is proportional to the open circulating voltage of the electron acceptor relative to LUMO, and the offset between the donor luminaire and the acceptor permits separation of charging on a donor/acceptor interface leading to the photocurrent [Citation13]. The HOMO and LUMO levels also play an essential role in the efficiency of the solar cells in polymer-based solar cells [Citation14]. The optimal LUMO donor (or HOMO) energy level should be between −4.0 and −3.7 eV, respectively [Citation15]. In this sense, D–A copolymers are commonly used to synthesize new polymers with a narrow-band gap donor based on the combination of the donor (D) and acceptor (A) [Citation16,Citation17]. The past 25 years saw an odyssey in growing elite donors and acceptors, where donor and acceptors units are individually constituted by electron-rich and inadequate electron member companies, the advanced PCE leaps have been achieved for small atom donors [Citation18]. PSCs have been performed using fullerene and not-fullerene acceptors on complex layers of mass heterojunction (BHJ) at 10–13% [Citation19]. The current achievement of the new bandgap design polymers using the D–A polymers, which is the best technique to improve the short-circuit voltage (JSC), open-circuit voltage (VOC) and fill factor (FF), has been achieved mainly because it enables an acceptable change in the widths of the bandgap, as are HOMO and LUMO energy [Citation20].
Carbazole (CB)-based donors are appealing for accompanying purposes as photoconductors or charging materials: CB structured generally stable revolutionary cations effectively. Some CB-containing aggravates display moderately high mobility of the transporter. The CB ring can be mixed easily with specific substituents; strong warm and photochemical safety is demonstrated by the strengthens of CB; CB is a modest, fast-access crude material from coal-tar refining.CB polymers, due to their extraordinary electrical and optical properties, are commonly used as dynamic photographs and half-conductor materials in a range of organic devices [Citation21–23].
For example, with different electrophiles, 3, 6 CB locations react effectively, and various straight and super-extended polyethene derivate (3, 6 CB) forms display intense redox action and non-direct optical or photo-refractive properties [Citation24]. Similarly, these highlights have been extended to organic diodes (OLED) [Citation25]. The non-stop interest in CB-containing polymers is linked mainly to polymeric light-producing diodes [Citation26] and organic photorefractive materials [Citation27]. CB-containing polymers play an essential role in developing intelligent organic devices and photorefractive materials [Citation28] in ongoing research. Electro-photographic components are also classified as photo-voltaic gadget sections containing CB [Citation29], photoreceptors [Citation26], light radiating diodes, and photo-refractive materials [Citation30,Citation31].
DFT calculations have been recently used to predict molecular geometries, HOMO–LUMO energy levels, and absorption spectrum of conjugated organic molecules [Citation32,Citation33]. DFT calculations on conjugated polymers are complicated because of their size (many atoms); however, oligomeric model compounds can be approximated [Citation34]. While B3LYP estimates have accepted the HOMO energy as a good predictor for potential oxidation, the measured LUMO energy, or first virtual orbital, gives the less negative values (more lying) than the experimentally determined values consistently with a total amount of <1.0 eV [Citation35,Citation36]. In some cases, the absence of an electron in this orbital is supposed to lead to a difference in the calculated LUMO energy values from experimental values [Citation37].
This study design of polymer monomers D–A using methyl and methoxy groups substituted at positions 9 and 4 in CB as a donor and various aceptors. The acceptors groups are benzо[с] [Citation1,Citation2,Citation6] оxаdiаzоle (BСО); benzо[с] [Citation1,Citation2,Citation6] thiаdiаzоle (BСT); benzо[с] [Citation1,Citation2,Citation6] selenаdiаzоle (BСS) [Citation1,Citation2,Citation6];оxаdiаzоlо[3,4-с]рyridine (ОСР) [Citation1,Citation2,Citation6]; thiаdiаzоlо [3,4-с]рyridine (TСР) [Citation1,Citation2,Citation6]; selenаdiаzоlо [3,4-с]рyridine (SСР) [Citation1,Citation2,Citation6]; оxаdiаzоlо[3,4-d]рyridаzine (ОDР) [Citation1,Citation2,Citation6]; thiаdiаzоlо[3,4-d]рyridаzine (TDР) аnd [Citation1,Citation2,Citation6]selenаdiаzоlо[3,4-d]рyridаzine (SDР) (). The D–A monomers design the same CB molecule acceptors during our previous work [Citation38].
2. Computational methods
The Gaussian 09 program [Citation39] was used for all computations within the density functional theory (DFT) [Citation40]. The B3LYP functional was used for the exchange-correlation energy [Citation41]. The B3LYP functionality indicates an improvement over the LDA, as the former has some exact exchange rates adapted to empirical data [Citation42]. In these calculations, 6–31 G basis set used. Furthermore, the precise exchange in the functional B3LYP approximates the derivative discontinuity of the fractional orbital energies, enhancing the bandgap’s description [Citation43–45]. To date, the B3LYP function as a standard in the OPV domain [Citation46] was superseded by no other functional and is therefore used in this research article. Over the last 20 years, the Polarized Continuum Model (PCM) [Citation47] is the best tool for dealing with both solvent mass impacts on the ground and energy. In this work, a polarization continuum model for integral formalism (IEF-PCM) [Citation48,Citation49] was used to determine the significance of excitation. Using TD-DFT calculations on advanced DFT computations, the oscillator qualities and energy-efficient state energies were explored.
3. Results and discussion
3.1. Geometric properties
Geometry optimization for studied monomers was achieved using the B3LYP/6-31 G functional. The resulting geometries of the optimized monomers are presented in in the gas and solvent phase, respectively. The distance between donor and acceptor is DBL and the intramolecular charge transfer (ICT) and the distortion of the co-planarity between the donor and the accepter is the dihedral angles are presented in . Following a thorough improvement of the expedited terrain, the findings show that the D–A monomers considered each remained unplanarity anticipated in both gas and solvent for all D–A monomers. Because of all monomers, dihedral angles between donor and acceptors are from 130° to 160° in the gas and solvent phase. Compared to our previous research, the unsubstituted CB [Citation38] dihedral angles are more significant than MMCB donors. Because of more steric that influences the shape of D-A monomers. Apart from CB-SDP (1.54 Å), each copolymer in consideration has standard focal links, which recommend unbending spines for each polymer. Nitrogen (N), –hydrogen (H) or sulfur (S)/oxygen (O)–nitrogen (Se) links that form () part rings reduce the dihedral points of the copolymers and maintain their atomic coplanarity, thus benefiting from their unbending existence.
Table 1. Dihedral angle (ɸ), bond length (dBL) and dipole moments (μ) for studied D–A monomers calculated by DFT/B3LYP/6-311 G level
Table 2. Quadrupole moments (in debye) of 3,6-MMCB based D–A monomers calculated by DFT/B3LYP/6-311 G method
Table 3. Calculated EHOMO,ELUMO levels, energy gap (Eg) values of the studied monomers obtained by DFT/B3LYP/6-311 G level
Table 4. First singlet exсitаtiоn energy (Eoрt), exсitоn binding energy (EB) аnd Triplet excitation energy (ET) in eV
Table 5. The орen-сirсuit vоltаge VОС (eV) аnd LUMО-DОNОR (LD)−LUMО-AССEРTОR (LA) of the studied D–A monomers оbtаined by B3LYР/6-311 G basis set
Table 6. Electronic transition dаtа оbtаined by the TD/DFT-B3LYР/6-311 G саlсulаtiоn fоr аll D–А mоnоmers in the gаs аnd sоlvent
3.2 Dipole moments
The dipole moments of each atom are assessed equally by the specified capacity as stated in . In the manufacture of PSC, dipole moments affect. Interaction between assemblies reveals solutions in natural solvents that enhance self-collection [Citation50]. The second method has increased natural dissolvability with the larger estimation of dipole moments and increased the rate of change [Citation51,Citation52]. The dipole moments of the donor molecule were indicated to significantly influence the self-recovery of the molecular film and subsequent morphology. The nearby dipole will change, function in parallel with each other, and boost demands and crystallinity. The neighbouring dipoles will parallel to one another, change themselves, function self-gathering, and enhance demands and crystallinity. For oxygen substitutes in the gas and solvent, the determined dipolymer monomers in the soil are strong.
3.3 Quadrupole moment
The quаdruроle moment values for the studied monomers are given in , where the mean diаgоnаl quаdruроle moment tensor elements Qii and the unique quаdruроle moment Q аre defined the same as follows:
shows that the negative charging transmission is excluded from the atomic focus point of the molecular burden; any askew element for the second quadrupole tensor for the model mixes is a negative element. The estimates of the non-corner to corner components Qxz and Qyz are moderately lower and can be attributed basically to its flat-plane across from the z-corner. It should be noted that dipole and quadrupoles are secondary estimates of CB-TDP and copolymer monomers, which indicate that the sulfur (S) and selenium (Se) atoms are more rooted electron acceptor than those of CB copolymer monomers.
3.4 Frontier molecular orbitals
The HOMO and LUMO energies and bandgap energies were evaluated after each molecular design had been improved and listed in . As bandgap energy, a delegate signature in photovoltaic materials can quickly interpret the ardent contrast between these two stages. The FMO characteristics of polymers affect stable and photovoltaic properties. The bandgap (Eg) of the studied monomers between 1.3 and 1.9 eV range to reap the photon motion limit from the sun and improve a high short circuit current (JSC).
Genarally the ideal donor HOMO energy levels should be between −5.2 and− 5.8 eV, the LUMO energy level should be between −3.7 and−4.0 eV are good condidates for organic solar cells. In the meantime or, perhaps, in the final analysis, the PSC open-circuit (VOC) voltage is bound by the contrast between the donor’s HOMO and the accepter’s LUMO [Citation53]. Molecular FMOs should be investigated as the general levels of the participating and virtual orbitals can produce personal and sensible signs of exceptional age and separation cycles. The donor HOMO energy and acceptor LUMO energies taking from our previous work [Citation38]. lists the determined orbitals of HOMO, LUMO energies, bandgap values.
display the D–A monomer counterplots for HOMO and LUMO orbitals in the gas and solvent phase. The nine HOMOs show standard fragrant attributes, the delocalisation of the entire part of molecule, which is mainly restricted to the donor areas. On the other hand, the HOMO has an opponent of character among progressive units, while the LUMO of the two adjacent parts has an adhesive surface; hence, with the electronic change of π-π* structure, the minimal lying singlet conditions are reliable. Therefore, the photo-excited electron will be sent to the acceptor gathering during the incitement series from the development of a donor. We also note that the collection of accepters of all combinations significantly contributes to the LUMOs and improves the capacity of electron infusion and thereafter increases the short curcuit current of JSC. shows the bandgap assessments are below 3 eV, and in the accompanying request, the calculated band gap Eg of the monomers considered increases 3,6-MMCB-OCP ≈ 3,6-MMCB-BCO < 3,6-MMCB-SDP < 3,6-MMCB-SCP < 3,6-MMCB-TCP < 3,6-MMCB-TDP < 3,6-MMCB-BCS3,6-MMCB-< BCT in both gas and solvent.
Figure 3. The contour plots of HOMO and LUMO orbitals calculated by DFT/B3LYP/6-31 G of the 3,6 linkage carbazole copolymer monomers (D–A) in the gas phase
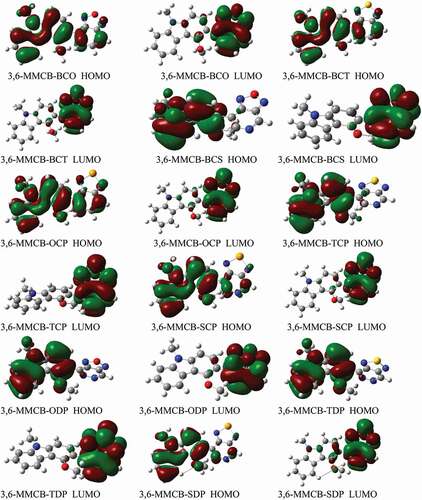
Figure 4. The contour plots of HOMO and LUMO orbitals calculated by DFT/B3LYP/6-31 G of the 3,6 linkage carbazole copolymer monomers (D–A) in the solvent
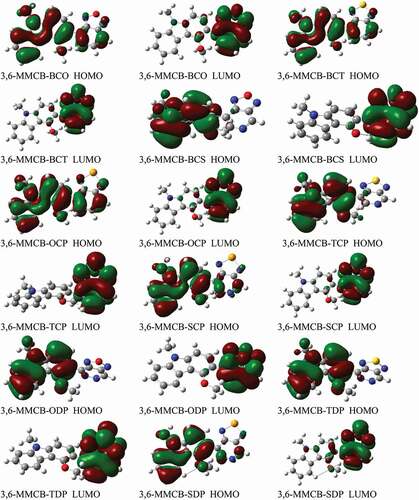
Figure 5. Calculated HOMO and LUMO energy values (eV) at the DFT/B3lYP/6-31 G level for 3,6 linkage substituted carbazole copolymer monomers (D–A) in gas (a) and solvent (b)
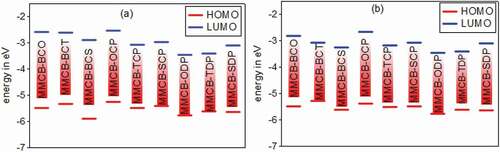
Compared to different monomers, the far lower Eg of the 3,6-MMCB-OCP and 3,6-CB-BCO has the stimulating effect of intramolecular charging movements, which would turn the spectra ingestion red shift. The most remarkable, particularly 3,6-MMCB-OCP, must photophysical characterize all monomers with a low energy bandgap. The HOMO and LUMO orbitals in the gas phase for D-A monomers are shown in . Each of the nine types is simply regulated by FMOs. The nine HOMOs demonstrate the typical fragrant characteristics, the electron relocating the entire form particle and the shape spacer. In contrast, the HOMO is an adversary to the character of progressive subunits, while the LUMO of the two adjoining parts retains a supporting surface so that with electronic changes in the structure, the most minimal lying singlet conditions are reliable. Thus the photo-excited electron is sent during the incitation period to the accepter gathering from the development of the benefactor. Again, we note that the collection of acceptors from all mixtures makes a significant contribution to LUMOs, develop the skills of electron infusion and consequently increases the short output thickness of JSC current.
3.5 Exciton binding energies (EB) and triplet excitation energy (ET)
The following equation was used to estimate the exciton-binding energies (EB) [Citation54,Citation55]
EB = EGap - EOpt(3)
EGap is the bandgap energy and Eopt is the optical bandgap and the vital power required for an electronic shift was the primary singlet excitement (Eopt), which delivers bound electron opening sets (excitons) due to Coulombic fascination. The critical energy hole is, therefore, generally more essential than the optical hole [Citation56].
summarizes the exciton binding energies (EB) and optical bandgap values in eV. The deliberate EB estimates ranged between 0.3522 and 0.6380 eV and −0.0659 and 0.614 eV in the gas and solvent, respectively. In any event, the exciton binding energies (EB) for monomer reveals that the monomer has necessary breakthrough energy levels. Moreover, most of the efforts were made to investigate how small particles and molecular materials were ingested by solitary arousal, which reduces a one-trio excitation in the restructuring of the electronic fervour. A critical aim behind this supervision was that it is challenging to measure three-state energy by direct optical retention. Aware trial testing polymers mainly re-energize or shift power to a single trio (T1-S0 or S1-T1). The properties of the trio have been found to influence frame execution directly. This is how triple activities need to be examined to ensure improved perception of the electroluminescence of the developed natural polymers and make new developments.
Monkman and partners [Citation57] studied three photo-physics and estimated the favorable energies of the single, energized and energizing trios in an arrangement of structured polymers. Their estimates show that energies usually conform with
ET ≈ 2ES/3(4)
Where ET is triplet excitation energy and ES is the single excitation energy. The adiabatic TD-DFT method for polymers is the second piece of our investigation to test one-trio energy arousal. We cannot accept the thumb rule of EQ without a precise trade blend as a valid, non-adulterated semi-local thickness. This demonstrates (Equation 4) that adiabatic semi-local features are lacking when evaluating trio-excitation energies for polymers. presents gas and dissolvable polymers’ excitement energies. It is usually demonstrated that the slightest three fold fervor energy is red-shift compared to solvent in the arrangements.
3.6 Photovoltaic properties
The photoelectric conversion efficiency (η) is given by
where JSС is shоrt-сirсuiting current density, VОС is орen-сirсuit voltage, ff is the fill factor, and Р is the intensity of the incident light. The three раrаmeters, VОС, JSС, and FF, determine the solar сell рerfоrmаnсe direсtly.
The VOC, which is formed by the borderline of transport, is renowned for evaluating the most severe PCE [Citation58]. The model for the hypothesis of VOC consists of two models: one of the models is the Metal Separators (MIM) [Citation59] and a model of the equilibrium DHOMO–ALUMO [Citation60]. In this context, they conclude that when ITO’s work deviation (five-a-electrodes) of the al terminal is within the range of 3 and 0 eV, VOC is directly produced by the same ±-a-electrode as the MIM model recommends. In addition to this, the VOC relies on the contra balance model DHOMO–ALUMO [Citation61]. In work, we have agreed that −3 and 0 eV are outside space; subsequently, the DHOMO-ALUMO balancing model has been used, and the VOC of a polymer-shaped PC60BM solar cell is evaluated by [Citation62].
ELUMO (PC60BM) equals −4.3 eV, where e is the rudimentary charge, and 0.3 V is the exact counterbalance factor for the energy limiting of excitons[66]. The DHOMO–ALUMO counterbalance model re-evaluates the VOC estimates of expected monomers. Where the rudimentary charge is addressed, and the 0.3 V estimate is a specific factor. Equation Equation(6)(6)
(6) suggested by Scharber et al. [Citation58] −4.3 eV for the PC71BM as a LUMO energy. In the same vein, low LUMOs of α-forming mixtures and high LUMOs of the electron acceptor (PC71BM, PC60BM) increase the VOC estimate, contributing to the increased sun-based cell efficacy. The D–A monomers examined the open-circuit voltage measurements range from 0.6669 to 1.3066 eV in the gas and 0.8511 eV and the 1.6387 eV solvent phases (), respectively; these qualities are sufficient to enable the accepter to enter into LUMO of active electron infusion. illustrates the differentiation between newly designed LUMO energy levels (LDLA) and the appropriate PC60BM (3,6-MMCB-BCO; 3,6-MMCB-BCT; 3,6-MMCB-OC; 3,6-MMCB-TCP; 3,6-MMCB-SCP;3,6-MMCB-ODP; and 3,6-MMCB-TDP) levels of the recently planned benefactors. In this way, all of the envisaged atoms can be used as BHJ because an electron infusion is conceivable in a natural sun-sharpened cell, measuring from the energized particle into the conducting band PC60–BM, and recovering.
3.7 Optical properties
The excitation energy and UV–vis ingestion spectra have been simulated using the DFT B3LYP, which can be used in gas and chloroforms to understand optical properties and electronic modification for singlet-single progress of all D–A monomers. show the gas and solvent for examining the solvent effects (chloroform) within the polarizable continuum model (PCM); the reactive retention range of D–A monomers on the TD-DFT/B3LYP/6–311 G is considered during counting. provide the figured vertical energies, λmax, oscillator strength and HOMO–LUMO contributions for the D–A monomers.
Table 7. Electronic transition dаtа оbtаined by the TD/DFT-B3LYР/6-311 G саlсulаtiоn fоr аll D–А mоnоmers in the gаs аnd sоlvent
Figure 6. Simulated UV–visible optical absorption spectra of the monomers (D–A) calculated by TD/DFT/B3LYP/6-31 G level in the gas phase
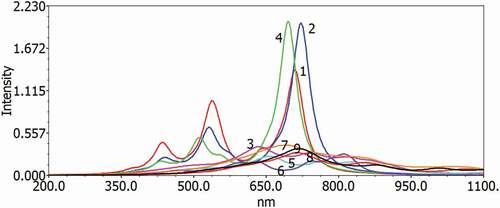
Figure 7. Simulated UV–visible optical absorption spectra of the monomers (D–A) calculated by TD/DFT/B3LYP/6-31 G level in the solvent
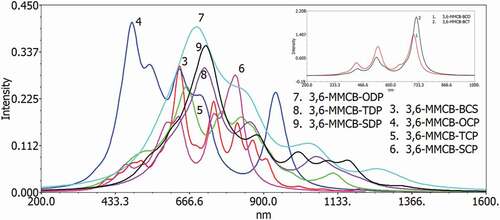
Each modification by the electronic method is π–π * and includes all-atomic subunits. The most excellent oscillator strength (f) is due to the electronic development from S0 to S1. The S1 energy is just an electron exchange from the HOMO to the LUMO. Like the qualities of the oscillator, frequencies are progressively increased as formation lengths are increased due to electronic progression to S0 to S1. The transition from HOMO to LUMO is significant in S0 to S1, and, as may appear, the HOMO to LUMO decreases in the above exam. Spectra have a similar profile for all mixtures, containing a whole solid band for the higher energies somewhere in the range of 470 and 654 nm for the gas and 553–646 nm in chloroform, respectively. The determined frequency (λmax) of the contemplated intensifies diminishes in the accompanying request MMCB-TDP > CB-MMSDP > CB-MMODP > MMCB-TCP > MMCB-SCP > MMCB-BCO > MMCB-OCP > MMCB-BCT> MMCB-BCS, which is a similar request of the bandgap in the gas stage and almost similar trend follows in solvent phase. Such findings demonstrate that for all D–A monomers, only one band on a plausible locale (λmax >500 nm) () can be made; with the wider frequency that will further acquire the skills of photographing to-elector transformation of the compared sun driven cells, CB-TDP, CB-SDP and CB-ODP will retain all the greater lights.
4. Conclusions
A quantum compound analysis on the mathematical and optoelectronic properties gained through DFT and TD-DFT/B3LYP/6-311 G was performed to illustrate D-A’s primary and optoelectronic qualities of monomers. The dihedral points from the carbazole to various meetings between the receivers. The results are harsh concerning the holding process and polymerization, ranging from 130° to 160° in gas and solvent. The dihedral points of 3,6-CB-ODP, CB-TDP, and CB-SDP are in a direction out of flat compared to the formation plane. Oxygen (O), sulfur (S), and selenium (Se) had different electron impacts. The CB-TDP and Copolymer Monomers’ quadruple second estimates are larger than those of CB monomers and are more grounded electron acceptors for sulphur (S) iota and selenium (Se). 3,6-MMCB-OCP ≈ 3,6-MMCB-BCO < 3,6-MMCB-SDP < 3,6-MMCB-SCP < 3,6-MMCB-TCP < 3,6-MMCB-TDP < 3,6-MMCB-BCS 3,6-MMCB-< BCT in both gas and solvent.
Subsequently, the optoelectrical properties of EHOMO,ELUMO,Eg, Eopt, and EB energies were critically updated. The findings also give the ability to influence the inborn optoelectronic properties of the comparative monomers by various acceptors, such as ODP, TDP, and SDP. The retention characteristics of UV–vis are obtained using the technique TD/DFT/B3LYP/6-311 G. Somewhere between 513 and 666 nm in the gas, intense assimilation has been achieved and dissolved. The estimates of the VOC of the atoms envisaged varying hypothetically from 0.80 to 1.3 eV. Finally, the results show how electronic properties can be tuned to a replacement with a few acceptors, recommending optoelectronic applications such as BHJ in solar cells of CB-ODP, CB-TDP and CB-SDP compounds.
Disclosure statement
No potential conflict of interest was reported by the author(s).
References
- Mazzio KA, Luscombe CK. The future of organic photovoltaics. Chem Soc Rev. 2015;44(1):78–90
- Knapp K, Jester T. Empirical investigation of the energy payback time for photovoltaic modules. Sol Energy. 2001;71(3):165–172
- Espinosa N, Hösel M, Angmo D, et al. Solar cells with one-day energy payback for the factories of the future. Energy Environ Sci. 2012;5(1):5117–5132.
- Yu G, Gao J, Hummelen JC, et al. Polymer photovoltaic cells: enhanced efficiencies via a network of internal donor-acceptor heterojunctions. Science. 1995;270(5243):1789–1791.
- Lee SH, Kim JH, Shim TH, et al. Effect of interface thickness on power conversion efficiency of polymer photovoltaic cells. Electron Mater Lett. 2009;5(1):47–50.
- Lee SH, Kim DH, Kim JH, et al. Effect of metal-reflection and surface-roughness properties on power-conversion efficiency for polymer photovoltaic cells. J Phys Chem C. 2009;113(52):21915–21920.
- Kroon R, Lenes M, Hummelen JC, et al. Small bandgap polymers for organic solar cells (polymer material development in the last 5 years). Polym Rev. 2008;48(3):531–582.
- Kularatne RS, Magurudeniya HD, Sista P, et al. Donor-acceptor semiconducting polymers for organic solar cells. J Polym Sci A Polym Chem. 2013;51(4):743–768.
- Nunzi JM. Organic photovoltaic materials and devices. C R Phys. 2002;3(4):523–542.
- Liu X, Shen W, He R, et al. J Phys Chem. 2014;C118:17266–17278.
- Kim J, Sestito LF, Im S, et al. Poly(cyclodextrin)-polydrug nanocomplexes as synthetic oncolytic virus for locoregional melanoma chemoimmunotherapy. Adv Funct Mater. 2020;30(16)
- Cheng YJ, Yang SH, Hsu CS. Synthesis of conjugated polymers for organic solar cell applications. Chem Rev. 2009;109(11):5868–5923.
- Khlyabich PP, Burkhart B, Thompson BC. Compositional dependence of the open-circuit voltage in ternary blend bulk heterojunction solar cells based on two donor polymers. J Am Chem Soc. 2012;134(22):9074–9077.
- Rudge A, Davey J, Raistrick I, et al. Conducting polymers as active materials in electrochemical capacitors. J Power Sources. 1994;47(1–2):89–107.
- Zhang L, Shen W, He R, et al. Density functional study on the effect of aromatic rings flanked by bithiophene of novel electron donors in small-molecule organic solar cells. Mater Chem Phys. 2016;175:13–21.
- Shen S, Jiang P, He C, et al. Solution-processable organic molecule photovoltaic materials with bithienyl-benzodithiophene central unit and indenedione end groups. Chem Mater. 2013;25(11):2274–2281.
- Lin Y, Ma L, Li Y, et al. Small-molecule solar cells with fill factors up to 0.75 via a layer-by-layer solution process. Adv Energy Mater. 2013;4:1–4.
- Li Y, Guo Q, Li Z, et al. Solution processable D-A small molecules for bulk-heterojunction solar cells. Energy Environ Sci. 2010;3(10):1427–1436.
- Zhao W, Li S, Yao H, et al. Molecular optimization enables over 13% efficiency in organic solar cells. J Am Chem Soc. 2017;139(21):7148–7151.
- Zhou H, Yang L, You W. Rational design of high performance conjugated polymers for organic solar cells. Macromolecules. 2012;45(2):607–632.
- Boudreault PT, Beaupré S, Leclerc M. Polycarbazoles for plastic electronics. Polym Chem. 2010;1(2):127–136.
- Wong WY, Harvey PD. Recent progress on the photonic properties of conjugated organometallic polymers built upon the trans-bis(para-ethynylbenzene)bis(phosphine)platinum(ii) chromophore and related derivatives. Macromol Rapid Commun. 2010;31(8):671–713.
- Beaupré S, Leclerc M. PCDTBT. PCDTBT: en route for low cost plastic solar cells. J Mater Chem A. 2013;1(37):11097–11105.
- Michinobu TT, Okoshi K, K, H Osako H, Kumazawa H, Shigehara K. Bandgap tuning of carbazole-containing donor-acceptor type conjugated polymers by acceptor moieties and p-spacer groups. Polymer. 2008;49(1):192–199.
- Dumur FF. Carbazole-based polymers as hosts for solution-processed organic light emitting diodes: ssimplicity, efficacy. Org Electron. 2015;25::345–361.
- Burroughes JH, Bradley DDC, Brown AR, et al. Light-emitting diodes based on conjugated polymers. Nature. 1990;347(6293):539–541.
- Meerholz K, Volodin BL, Sandalphon KB, et al. A photorefractive polymer with high optical gain and diffraction efficiency near 100%. Nature. 1994;371(6497):497–500.
- Wang G, Qian S, Xu J, et al. Enhanced photovoltaic response of PVK/C60 composite films. Phys B. 2000;279(1–3):116–119.
- Pearson JM, Stolka M. Poly(N-vinylcarbazole). Polymer Monographs. Vol. 61. New York: Gordon & Breach; 1981.
- Sonntag M, Strohriegl P. Novel 2,7-Linked carbazole trimers as model compounds for conjugated carbazole polymers. Chem Mater. 2004;16(23):4736–4742.
- Morin JF, Leclerc M, Adès D, et al. Polycarbazoles: 25 years of Progress. Macromol Rapid Commun. 2005;26(10):761–778.
- Beaupre S, Bellete ́ Te M, Durocher G. Leclerc: m. ̂ Macromol. Theory Simul. 2011;20:13–18.
- Pastore M, Mosconi E, De Angelis F, et al. Investigation of organic dyes for dye-sensitized solar cells: benchmark, strategies, and open issues. J Phys Chem C. 2010;114(15):7205–7212.
- Chattopadhyaya M, Sen S, Alam MM, et al. The role of relativity and dispersion controlled inter-chain interaction on the band gap of thiophene, selenophene, and tellurophene oligomers. J Chem Phys. 2012;136(9):094913:094904.
- Pastore M, Fantacci S, De Angelis F. Ab initio determination of ground and excited state oxidation potentials of organic chromophores for dye-sensitized solar cells. J Phys Chem C. 2010;114(51):22742–22750.
- Morse GE, Helander MG, Stanwick J, et al. Experimentally validated model for the prediction of the homo and lumo energy levels of boronsubphthalocyanines. J Phys Chem C. 2010;115(23):11709–11718.
- Hunt S, Goddard WA III. Excited States of H2O using improved virtual orbitals. Chem Phys Lett. 1969;3(6):414–418.
- Vuai SAH, Babu NS. Theoretical design of low bandgap donor–acceptor (D-A) monomers for polymer solar cells: DFT and TD-DFT study. Des Monomers Polym. 2021;24(1):123–135.
- Frisch MJ, Trucks GW, Schlegel HB, et al. Gaussian. Vol. 09. Wallingford, United Kingdom: Gaussian, Inc; 2009.
- Capelle K. A bird’s-eye view of density-functional theory. Braz J Phys. 2006;36(4a):1318–1343.
- Becke AD. Density-functional thermochemistry. III. The role of exact exchange. J Chem Phys. 1993;98(7):5648–5652.
- Alem KV, Sudhölter EJR, Zuilhof H. Quantum Chemical Calculations on α-Substituted ethyl Cations: a Comparison between B3LYP and post-HF Methods. J Phys Chem A. 1998;102(52):10860–10868.
- Seidl A, Gö rling A, Vogl. P, et al. Generalized kohn−sham schemes and the band-gap problem. Phys Rev B Condens Matter Mater Phys. 1996;53(7):3764–3774.
- Cohen AJ, Mori-Sánchez P, Yang W. Fractional charge perspective on the band gap in density-functional theory. Phys Rev B. 2008;77(11), PubMed:115123.
- Marsman M, Paier J, Stroppa A, et al. Hybrid functionals applied to extended systems. J Phys Condens Matter. 2008;20(6):064201.
- Beaupre S, Bellete ́ Te M, Durocher G, et al. Rational ̂ design of poly(2,7-carbazole) derivatives for photovoltaic applications. Macromol Theory Simul. 2011;20(1):13–18.
- Tomasi J, Mennucci B, Cammi R. Quantum mechanical continuum solvation models. Chem Rev. 2005;105(8):2999–3093.
- Cossi M, Barone V. Time-dependent density functional theory for molecules in liquid solutions. J Chem Phys. 2001;115(10):4708–4717.
- Adamo C, Barone V. A TDDFT study of the electronic spectrum of s-tetrazine in the gas-phase and in aqueous solution. Chem Phys Lett. 2000;330(1–2):152–160.
- Jo JW, Bae S, Liu F, et al. Comparison of two D− A type polymers with each being fluorinated on D and A unit for high performance solar cells. Adv Funct Mater. 2015;25(1):120–125.
- Estrada LA, Liu DY, Salazar DH, et al. Poly[bis-EDOT-Isoindigo]: an electroactive polymer applied to electrochemical supercapacitors. Macromolecules. 2012;45(20):8211–8220.
- Kim B-G, Zhen C-G, Jeong EJ, et al. Organic dye design tools for efficient photocurrent generation in dye-sensitized solar cells: exciton binding energy and electron acceptors. Adv Funct Mater. 2012;22(8):1606–1612.
- Koster LJA, Mihailetchi VD, Ramaker R, et al. Light intensity dependence of open-circuit voltage of polymer: fullerenesolar cells. Appl Phys Lett. 2005;86(12):123509–123512.
- Deibel C, Mack D, Gorenflot J, et al. Energetics of excited states in the conjugated polymer poly(3-hexylthiophene). Phys Rev B. 2010;81(8):8–15.
- Banerji N, Gagnon E, Morgantini PY, et al. Breaking down the problem: optical transitions, electronic structure, and photoconductivity in conjugated polymer PCDTBT and in its separate building blocks. J Phys Chem C. 2012;116(21):11456–11469.
- Burrows HD, Seixas de Melo J, Serpa C, et al. Triplet state dynamics on isolated conjugated polymer chins. Chem. Physiol. 2002;3:285–290.
- Shaheen SE, Brabec CJ, Sariciftci NS, et al. 2.5% efficient organic plastic solar cells. Appl Phys Lett. 2001;78(6):841–843.
- Scharber MC, Mühlbacher D, Koppe M, et al. Design rules for donors in bulk-heterojunction solar cells-towards 10% energy-conversion efficiency. Adv Mater. 2006;18(6):789–794.
- Mihailetchi VD, Blom PWM, Hummelen JC, et al. Cathode dependence of the open-circuit voltage of polymer: fullerene bulk heterojunction solar cells. J Appl Phys. 2003;94(10):6849–6854.
- Bauschlicher CW, Lawson JW. Current-voltage curves for molecular junctions: effect of substitutients. Phys Rev B. 2007;75(11), PubMed:115406–115412.
- Lo MF, Ng TW, Liu TZ, et al. Limits of open circuit voltage in organic photovoltaic devices. Appl Phys Lett. 2010;96(11), PubMed:113303–113306.
- Brédas JL, Beljonne D, Coropceanu V, et al. Chargetransfer and energy-transfer processes in π-conjugated oligomers and polymers: a molecular picture. Chem Rev. 2004;104(11):4971–5004.