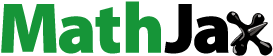
ABSTRACT
Due to the unique properties such as nontoxicity, biodegradability, availability from renewable resources, and cost-effectiveness, polysaccharides play a very important part in the science and technology field. The various chemically modified derivatives of these offer a wide range of high value-added in both food and non-food industries. Among the chemical modification, etherified polysaccharide is one of the most widespread derivatives by introducing an ether group which is commonly stable in both acidic and alkaline conditions. Hydroxyalkylation, alkylation, carboxymethylation, cationization, and cyanoethylation are some of the modifications commonly employed to prepare polysaccharides ethers derivatives. There also has been a growing tendency for creating new types of modification by combining the different means of chemical techniques. The correct determination of degree of substitution (DS)/molar substitution (MS) is crucially important. The objective of this article is to summarize developments in synthetic etherified polysaccharides, involving analytical methods for determination of MS/DS, measurement processes, and the associated mechanisms.
1. Introduction
In recent years, the requirement for polysaccharide derivatives for various uses has increased dramatically. There are a great many factors contributing to these increases include: (1) There are inexpensive and readily available sources of polysaccharides all over the world, especially for starch, cellulose, and glycogen; (2) polysaccharides have good compatibility with numerous other substances during production, especially hydrocolloid in foods; and (3) polysaccharides are renewable, eco-friendly, and biodegradable. Indeed, on a global scale, intensive efforts are concentrated to produce different kinds of polysaccharide derivatives for different applications that apply to different industries.
Due to the disadvantages of native polysaccharides that limit their use in both food and non-food applications, the modification by chemical or physical techniques is necessary [Citation1]. The various modification technologies, particularly chemical and physical modifications, can alter the properties of different polysaccharide derivatives and their pastes and gels in different ways. Thereinto, the functional properties of native polysaccharides can be improved by chemical modifications, including but not limited to esterification [Citation2], etherification [Citation3–5], acylation [Citation6], crosslinking [Citation7], oxidization [Citation8], and depolymerization by acid [Citation9] or enzymatic hydrolysis [Citation10].
Modifying polysaccharide by etherification significantly changes the physicochemical properties, for example, hydroxypropylation can effectively inhibit the ordered structure of starch paste, retard the retrogradation, enhance the fluidity, and improve the clarity [Citation11]; quaternization acquires higher solubility, better heavy anion-exchange capacity and metal ions sorption [Citation12], alkylation stimulates the benzo[a]pyrene (BaP) aqueous solubilization, and presents high surfactant properties [Citation13]. Furthermore, the specific characteristics of the polysaccharides and the levels of chemical modification are closely related. [Citation14–16] Therefore, it is necessary to determine the extent of etherification as well as the distribution of substituents. Moreover, with the increasing industrial importance of modified polysaccharides, the interest in methods for its analysis is growing.
Normally, there are two parameters which are used to represent the amount of derivatization [Citation14–15]. Simplistically, for now, we will take starch for example. One is degree of substitution (DS), i.e., the average number of hydroxyl groups per repeating unit that were replaced by a given substituent, which is defined by the amount of hydroxyl groups in the repeating unit that can be chemically modified. Hence, the maximum DS varies with the structures of polysaccharides, which is restricted by the total amount of hydroxyl groups that are available within the repeating unit. The maximum DS for starch, cellulose, and glycogen is 3, however, the maximum DS for agarose and xylan is 4 and 2. Some substituents, like hydroxy alkyl groups, feature a hydroxyl group themselves that is also accessible for chemical modification. In these cases, an additional descriptor is required, i.e., molar substitution (MS). The MS value describes the average number of substituents per repeating unit that were introduced. This value can exceed the total amount of hydroxyl groups per repeating unit. The parameter of MS depends on the synthesis conditions of etherified polysaccharide and can vary over a wide range. MS is associated with the degree of side chain formed; the size of the MS value can be theoretically infinite. Among the hydroxyalkylation, hydroxyethyl starch (HES), for example, the hydroxyl group of each hydroxyethyl group can be hydroxyethylated, even multiple etherifications. Thus, the MS is often used to define the formed chains of substituent groups and is used hereafter. So, the MS is the correct term to use for the formed chains of substituent groups and is used hereafter. Meanwhile, the convenient and practical methods are essential to the determination of DS/MS.
Along with the rocketing development of carbohydrate chemistry in the past several decades, a variety of modification strategies and techniques have been discovered and successfully applied and the determination of DS/MS has become a problem that cannot be ignored. A number of review articles concentrated entirely on preparation, characterization of physicochemical properties and application of etherified polysaccharide [Citation16–22], and there is almost no comprehensive review of this topic in recent years. An earlier extensive review by Morgan in 1946 [Citation23] was directed toward the development and improvements of previous methods for the determination of ethylene glycol ethers. In so doing, his research helped pave the way for many valuable explorations in the study of glycol ethers, including hydroxyethyl cellulose. Numerous examples of the use and modified of Zeisel, spectrophotometric, and other methods for the determination of the alkoxyl substituent in polysaccharide were summarized by Cobbler and Samsel in 1962 [Citation24]. Mini-reviews of determination of the hydroxypropyl (HP) level in modified cellulose and starch were surveyed by Ho and Seib, respectively [Citation25,Citation26]. More recently, the methods of determination of amounts of hydroxypropylation were summarized concisely by Fu in 2019 [Citation27], and the determination of the DS of starch esters was outlined by Shi in the ‘DS determination’ section of Part three of the article [Citation1].
Due to insufficient attention paid by the published literature reviews of the comprehensive determination methods of the extent of etherification, we decided to summarize the analytical methods for determination of MS/DS of etherified polysaccharide, including the alkyl ethers, hydroxyalkyl ethers, carboxymethyl ethers, cyanoethyl ethers, cationic ethers, and mixed ethers. Throughout the paper, a systematically discussion of measurement processes and associated mechanisms is also presented. The objective is to enlighten the researchers to identify challenges and opportunities related to this field.
2. Hydroxyalkyl ether
The hydroxyalkylation of polysaccharide involves one or more hydroxyl groups on an anhydroglucose unit (AGU) reacting with epoxides in alkaline conditions. Hydroxyalkyl ethers have () been prepared earlier using ethylene oxide (EO), 1,2-propylene oxide (PO), 1,2-butylene oxide (BO) [Citation28,Citation29], and other long-chain 1,2-epoxyalkanes [Citation30]. Starch hydroxyls attack at the least sterically hindered site on the epoxide via a bimolecular nucleophilic (SN2) mechanism. Several analytical methods for the determination of the MS of hydroxyalkyl ethers have been developed over the years and is generally mature enough.
2.1. Hydroxyethylation
Hydroxyethylation of polysaccharides was most commonly found in hydroxyethyl starch (HES) and hydroxyethyl cellulose (HEC). For example, HES, as a medically plasma volume expander, the MS and DS are the key determining factors of the duration of pharmacologic action, solubility, and stability in water [Citation31]. As we noted earlier, because of multiple substitutions, there is some difference between DS and MS [Citation32].
The previously described method for the analysis of ethoxy group was determined by chemical titration. The measurement of Werner and Mitchell [Citation33] is generally upon the chromic acid oxidation of the ether and titration of the excess dichromate, which was suitable for monomethyl ethers of ethylene glycol. The classical Zeisel method used constant-boiling hydroiodic acid to cleavage the hydroxyethyl ethers into their corresponding alkyl iodides. [Citation34,Citation35] Due to the lack of adequate awareness of another product, i.e., ethylene, it failed to give quantitative and reproducible results in a very long period of time. Until 1946, Morgan [Citation23] devised a modified alkoxyl method and apparatus which was suitable for the determination of hydroxyethyl cellulose via the titration of ethyl iodide and ethylene with silver nitrate and bromine, respectively. In consideration of the feasibility of low substituted hydroxyethyl starch and the amount of excess hydriodic acid, Lortz [Citation36] modified and strengthened the Morgan’s alkoxyl apparatus, adjusted the sample size and corresponding hydriodic acid to regulate the small amounts of ether substitution within the scope of 0.005 to 0.20 hydroxyethyl and hydroxypropyl group per anhydroglucose unit. However, the method is not ideal for alkyl groups of longer chain length than propyl, for instance, hydroxybutyl ether. The absorption-titration method has been used for a long time both here and abroad. In combination with gas chromatographic (GC) technique, several modified Zeisel methods for creating increasingly precise determination for alkyl cellulose ethers, [Citation24,Citation37] hydroxyethyl starch and derivatives [Citation38,Citation39–42] have been published. The analyses of a series of ethyIcelIulose specimens [Citation24], HES 130/0.4 [Citation43,Citation44] and HES 150/0.5 [Citation45] by the gas chromatographic method and the method of chemical titration were compared, the technique of Zeisel gas chromatography provides a simple, rapid and reproducible quantitative analysis method. The proposed mechanism for the hydriodic acid decomposition procedure of HES and HEC is illustrated in Scheme 1. As it is mentioned in the mechanism, there are two routes. One is acid catalyzed and the direct conversion to iodoethane. Another is that the final iodoethane was synthesized via ethylene intermediate. Two routes can lead to the production of iodoethane.
The MS ratio calculation of the HES/HEC is theoretically based on the ethylene oxide unit (C2H4O) by the following equation 1. [18] The parameters E and m in formula 1 equal the weight of iodoethane and sample; WE is the weight % of EO in HES; the values 155.97, 162.14, and 44.05 in equation equal the molecular weight of iodoethane, AGU, and C2H4O.
Furthermore, in consideration of the systematic errors of the destructive analysis of HES, such as incomplete cleavage or chemical side reactions during acid hydrolysis, etc., a non-destructive, fast and relatively accurate method to estimate MS with minimal and simple sample preparation is crucially important. As we all know, the NMR spectroscopic method is a powerful tool for analyzing polysaccharide structures. In 2015, Moiseev and co-workers [Citation46] reported the modified Proton Magnetic Resonance (PMR) spectroscopy technique which was used for determining the MS in HES. The results suggested that the PMR spectroscopy is a sensitive and accurate technique with some advantages by correlating the integrated intensities of resonances for terminal anomeric protons, substituted branching chains and unsubstituted AGU residues in HES. In allusion to the identification and quantitative analysis of impurities, it also has certain advantages.
On the other side of the coin, for the most natural polysaccharides mainly of starch and cellulose, the site where the chemical modification occurs, would at its C-2, C-3, and C-6 hydroxyl groups of the AGU to generate the esters, ethers, carbonates or carbamates, etc. The determination of DS and regioselectivity of these which are vitally important for the characterization of original and technologically advanced materials can often be a complex task [Citation47]. For hydroxyethylation, take the HES for example again, the DS of HES has been to shown to be an important measure for determining the efficacy of different starches [Citation32–50]. For the modification of glucose unit, different substitution degree ratios on O-2, O-3, and O-6 have been published [Citation51–54]. The substituent distribution of hydroxyethyl starch has been determined by capillary GC [Citation52] or GLC [Citation55] or GC-MS [Citation41–54] of the silylated hydrolysate with the exception of the early joint use of paper and thin-layer chromatography and spectrophotometry [Citation51].
2.2. Hydroxypropylation
Hydroxypropylation is a widely used and accepted means to modify the structure and properties and promote the functionality of polysaccharides such as chitosan [Citation56–58], starch [Citation59,Citation60], cellulose [Citation61,Citation62], and others [Citation63,Citation64]. It was effective in improving shelf life, freeze-thaw stability, cold water swelling, and reconstituting characteristics. Take Hydroxypropyl starch (HPS), for example. By reacting with PO, the hydroxypropyl groups were introduced into the glucose unit of alkali-activated starch. As a result, the retrogradation has been prevented, the paste clarity has been improved, and the shelf-life, freeze-thaw stability, and cold storage stability to starch-based food products have been extended. The extent of substitution is a key element which influenced the changed physicochemical properties. Several methods used to determine DS/MS have been proposed. In this section, the classical HPS () and a new-type of HPS () will be discussed separately ().
2.2.1. Classical HPS
There are significant numbers of previous researches on hydroxypropylation of starch with PO. In other words, the hydroxyl group on C-2 of hydroxypropyl rather than the terminal position, namely, β-HPS (). Due to the stability of ether groups in acid and alkali circumstance, β-HPS is a class of modified starch which is widely used in food industry. Meanwhile, the FDA stipulates that all of the hydroxypropylated starches fit the maximum permissible level limitation in food applications, that is to say, the MS should not be more than 0.2 [Citation65]. And for β-HPS, the spectrophotometric (colorimetric) method of Johnson [Citation66,Citation67] is used to determine the MS of hydroxypropyl group and is also an official standard method of the Joint FAO/WHO Expert Committee on Food Additives.
The colorimetric method is based on reaction of ninhydrin with propanal which is liberated from the HPS during an acid digest, that is to say, involving the dehydration of 1,2-propanediol which is generated from the hydrolysis of the 2-hydroxypropyl group, and then to propionaldehyde and the enolic tautomer (or form) of propanal, which is an isomer of allyl alcohol. As early as 1957, Jones and Riddick [Citation68] had been reported that the 1,2-propanediol was dehydrated to a mixture of allyl alcohol (2-propen-1-0 l) and the enolic form of propionaldehyde by treatment of concentrated sulfuric acid (Scheme 2), and the mixture of allyl alcohol and propionaldehyde can be measured spectrophotometrically at 595 nm by reacting with ninhydrin to generate a violet-colored complex [Citation69].
A standard curve was made using 1,2-propanediol, and native starch was used as a control [Citation66–70]. By using the following formula 2, the MS was calculated through the spectrophotometer by a conversion constant of 0.7763. The parameters P and m in formula 2 equals the weight of the measured propylene glycol and sample, and F is dilution factor; the values 162.14 and 58.08 in equation equal the molecular weight of AGU and C3H6O;
Another method is derived from Zeisel determination. In the early stages, the determination of MS of polysaccharide derivatives was mostly using the Morgan method [Citation23] which had been identified as a standard method for testing hydroxyethyl cellulose (HEC) by ASTM [Citation71] in 1976 with some modification by Lortz [Citation36]. The apparatus, operating conditions, and calculation methods had been improved for purpose of making it suitable for the determination of hydroxypropyl ethers. Wang [Citation72] and Xiang [Citation73] modified the classical Morgan method and improved the reproducibility (RSD<1%). As gas chromatography develops, the new improvement in separation and determination of the alkyl halides become possible. In 1962, Cobler and Samsel [Citation24] investigated the 3-isopropoxy-n-propylamine and hydroxypropyl cellulose (HPC) ethers by the modified Zeisel method with gas chromatographic analysis which affords a 50% time saving. In 1979, an improved Zeisel gas chromatographic technique had been reported for the determination of MS in HPC by Hodges [Citation37]. The experimental and calibration of HPC were determined and a proposed mechanism for the HI-decomposition procedure of HPC was illustrated in .
FTIR spectroscopy was used to detect hydroxypropylation in modified starches. It can not only characterize the structure of the HPS, but also estimate the degree of substitution by using the derivative difference spectroscopy. In the past, IR spectroscopy had been used to estimate the degree of substitution of various modified starches (e.g., cyanoethyl starch, acetate starch, sulphate starch) [Citation74] and pectin [Citation75]. Due to limitations of the instrumentation, FTIR spectroscopy was used to detect hydroxypropylation in HPS successfully until 1992 [Citation76]. The hydroxypropyl substitution can be detected in a modified starch by IR. As is shown in , compared with the other functional groups, the methyl group is the only distinctive characteristic which observed as a peak of asymmetric methyl C-H stretching centred at 2974 cm−1. The magnitude of the peak varies according to the contents of HP substitution in the starch, and the peak is clearly defined. The method provides a rapid means of detecting hydroxypropylation by using the second derivative difference spectrum of the HP group. The area under the peak centered at 2974 cm−1 is calculated, and the content of HP group is measured by utilizing a calibration curve. The studies demonstrate a correlation between the content of HP substitution and the spectral property in the mid infra-red region. It would be a means of rapid quantitation once there were characteristic peaks and a reliable set of secondary standards.
Last but not least to mention is that the proton nuclear magnetic resonance [Citation1H-NMR] spectroscopy can effectively measure the relative contents of HP groups and glucose units [Citation77–79]. As with FTIR spectroscopy, it is also utilizing the ratio of the respective proton integrals between the chemical shifts of the methyl group protons of the HP group and the protons of the polysaccharides. The [Citation1]H-NMR method is a two-step method, comprising of depolymerization and deuteration. The purpose of the former process is to cut down the molecular weight of the polysaccharides with the treatment of acid-catalyzed hydrolysis [Citation77–80] or α-amylase-catalyzed hydrolysis [Citation26–83]. After the former hydrolysis process, all hydroxyl group protons were exchanged with deuterium by treatment with a right amount of D2O added into the NMR tube ().
There are two general approaches in which the HP contents of HPS could be calculated. One approach uses the known concentrations of CH3COOH (or CH3COONa) as an external standard, so, the integrated intensities of protons on the HP methyl group (IHP) and acetyl (IAc) could be utilized to determine the MS directly with the following Equationequation 3(3)
(3) , where m and I, respectively, represent the weight and comprehensive peak resonance integrated areas of the resonances assigned to the methyl groups of HP and acetic acid.
The other approach utilizes the comprehensive area of the six protons on C-2, C-3, C-4, C-5, and C6 of a glucosyl unit other than hydroxyl group protons [Citation80,Citation81] or the anomeric proton [Citation78] to calculate MS. With the former, the MS can be figured up by using the proportion of the integrated intensities of protons on HP substituents and oxygenated carbons (HCO) of the AGU, including the methylene (C-6) and methine (C-2, C-3, C-4, C-5) protons to the integrated intensity of the HP methyl signals without regard to the anomeric proton (EquationEquation 4(4)
(4) ). For the latter, the anomeric proton of the AGU of starch was used as an internal standard; the MS can be counted up directly from Equationequation 5
(5)
(5) . The symbol IHP in EquationEquations (4)
(4)
(4) and (Equation5
(5)
(5) ) is the integrated intensity of the methyl group (-CH3) on HP substituents, and the symbol IHAP is the integrated intensity of the anomeric (C-1) proton of the AGU, and IHCO is the integrated intensity of all the methylene (C-6) and methine (C-2, C-3, C-4, C-5) protons on the AGUs.
As for hydroxyalkyl etherified starch, the incidence of reaction is known to occur mainly at the hydroxy group on O-2 by reacting with EO and PO in base [Citation26–84]. The anomeric region of the AGU in starch and starch hydrolysis products have been distinguished previously by Gidley through the use of the [Citation1] H-NMR spectra [Citation85]. As for the distribution of HP groups on O-2, O-3, and O-6 of a glucosyl unit, Xu and Seib [Citation26] determined the distribution of HP groups of several starch ethers with DS values ranging from 0.05 to 0.23 by [Citation1] H-NMR, and concluded that the probability of HP substitution was 67%–78% on O-2, 15%–29% on O-3 and 2%–17% on O-6. With increasing the DS, the proportion (%) of O-6 leaned to rise slightly; for the O-3, however, tended to decrease slightly. Only the O-2 remained essentially unchanged. All of the conclusions are consistent with what has been found with cellulose and other starch ethers [Citation52–88].
2.2.2. New-type HPS
The HPS described above is β-HPS, i.e., the hydroxyl group on C2 of hydroxypropyl rather than the terminal carbon atom (). A new type of HPS, γ-hydroxypropyl starch (γ-HPS), is prepared by employing 3-chloropropanol as the etherifying reagent [Citation89]. The analogous-structured γ-HPS involving the hydroxyl group on the terminal C3 position of propyl group () was reported by our group. Two independent measurements for the determination of MS in γ-HPS were described.
One is colorimetric Method. In order to explore the feasibility, the classical spectrophotometric method of Johnson was measured. A calibration curve with 1,3-propanediol was prepared, and the wavelength optimum, reaction time and precision determination were investigated. Due to the result and the literature [Citation68], an improved colorimetric process was presented with a good repeatability (RSD = 0.37%) which was appropriate for determination of γ-HPS. The proposed mechanisms of two colorimetric processes were illustrated in . The MS ratio was calculated by the Equationequation (2)(2)
(2) .
Another is Zeisel-Gas Chromatography with a feasible mechanism for the degradation reaction through an assumed 1,3-diiodo intermediate. The process of the cleavage reaction could be partially understood by chromatographing the reaction products during the course of the experiment. The peak area ratio of 2-iodopropane (A)/ 1-iodopropane (B) is over 40:1, which illustrated that 2-iodopropane is the major hydrolysis product. The actual quantity of final hydrolysis product can be figured up by gathering A and B. Calculation of the MS of γ-HPS is theoretically based on the propyl oxide unit, C3H6O, by the following Equationequation 6(6)
(6) . Refer to the HES, the P and m in formula 5 equal the weight of iodopropane and the sample of γ-HPS; The value of number 58.08, 169.99, and 162.14 were represented the molar mass of C3H6O, iodopropane, and AGU.
2.3. Hydroxybutylation
Hydroxybutylation is also an important way to attain polysaccharides modification. Currently published literatures on hydroxybutyl-modified polysaccharides are more common in chemical modification of chitosan [Citation90–93]. For starch, the HES and HPS possess many advantages, thus great attention has been paid to their development and application by scholars both at home and abroad in recent years; but there is scarce study on the hydroxybutyl starch (HBS) which is part of nonionic starch ether [Citation94–96]. Good hydrophilicity and stability and favorable thermo-responsive property could be obtained by hydroxybutylation. Just like HPS, the classical HBS and a new-type of HBS will be stated and discussed separately in this section ().
2.3.1. Classical HBS
The classical HBS was synthesized by utilizing 1,2-epoxybutane as hydroxylalkylation reagent in aqueous NaOH (). The most frequently used method for the determination of the MS of HBS was ultraviolet spectrophotometry which was published by Harry-O’Kuru [Citation97]. This whole conversion process contains two parts illustrated below in . The first stage in conversion is hydrolysis involving the generation of 1,2-butanediol; the intermediate 1,2-butanediol will be turned into n-butyraldehyde and the enolic form of butenol by dehydration in the second step (). Meanwhile, both of the absorbance maximum and standard curves between absorbances and concentrations proved the feasibility of the method for the determination of the MS of HBS. All of the findings of the research provided basis for spectrophotometric procedure for estimating the MS of HBS. The method is simple, low cost, high accuracy, and can be used as a conventional method in industrial application.
2.3.2. New-type HBS
In recent years, there have been some researches about the synthesis and characterization of 2-hydroxybutyl starch, however, the new style of δ-hydroxybutyl starch (δ-HBS), i.e., the hydroxyl group in the terminal of butyl was rarely reported (). Owning to the difference in chemical structure and method feasibility [Citation68], the classical spectrophotometric method was not applicable to δ-HBS. Herein, an improved Zeisel gas chromatography for the estimation of the MS of δ-HBS was described [Citation98].
Different from the cleavage of HES/HEC or HPS/HPC [Citation38–42], the procedure of displacement reaction of δ-HBS was a little bit complicated. 1-Iodobutane and 2-iodobutane are the end products of HI-decomposition procedure. A few control reactions were conducted, and the latter is the major degradation product. As we stated before, the generated diiodide intermediate initially could be converted to the final iodobutane through two kinds of routes. Either of these routes can lead to the production of 2-iodobutane.
3. Alkyl ether
Etherification of native polysaccharides is generally obtained by reacting hydroxy or amino groups with alkyl halides, acrylonitrile, or epoxy alkanes in the presence of an alkaline catalyst. The various functional groups introduced with AGU substitution may be either hydrophobic or hydrophilic and they increase or decrease hydrophilicity of the modified polysaccharides. One of the typical hydrophobic functional groups is the alkyl or benzyl group, which is present in organic ethers of polysaccharides (cellulose [Citation99], starch [Citation100,Citation101], chitin [Citation102,Citation103], and pullulan [Citation104] under basic conditions. Increasing the substitution level with alkyl group markedly increases hydrophobicity of alkyl polysaccharide ethers.
According to different etherification substrates, several methods used to determine DS/MS have been proposed. For alkylation of chitosan, the DS per glucosamine unit was often determined by titration [Citation105,Citation106], C/N ratio of elemental analysis and NMR analysis [Citation103].
With the first approach, the extent of reaction and DS determination are derived by analyzing the change of bromide ions, that is to say, the generation rate of bromide ions is the conversion rate of etherification, and the DS of ethers can be calculated by the Equationequation 7(7)
(7) further [Citation106], where M is molar mass of the alkyl group; c is the concentration of silver nitrate standard solution (mol/L); V is the volume of consuming silver nitrate standard solution (mL); m is the weight of ethers.
The second method is specific to particular chitosan and its derivatives. The DS was calculated from the C/N ratio of elemental analysis, on account of a particular element nitrogen [Citation102–[Citation107–110]. And finally, the method of NMR analysis is suitable for nearly all the polysaccharides and derivatives with introduction of groups which have specific characteristic peak. For instance, the methyl and methylene of the propyl group from propyl-etherified amylose [Citation101], the methylene of the allyl group from allyl chitosan derivatives [Citation103], the methylene of the cyanoethyl group from cyanoethyl chitosan [Citation111]. DS was calculated from the peak area of the NMR spectrum by measuring and comparing the integral areas of proton signals in structural fragments. Reference is also made to the ‘2.2.1’ section in this article.
4. Other ether
Polysaccharides are typically the natural products. After a series of complicated chemical reactions, involving carbon-capture process, photosynthesis and more complex biosynthetic modifications, carbohydrates are formed. Therefore, different forms of modifications are applied to optimize the structural and functional properties of polysaccharides to achieve the targeted applications. So, different methods used to modify starch characteristics include enzymatic, physical, or chemical modification. Etherification is one of the most important means for the modifications of polysaccharides. Besides the above-mentioned ethers, we also emphasize other polysaccharide ethers in this section.
4.1. Carboxymethyl ether
Modification is commonly done to improve the structural composition, molecular weight, linkage pattern and ionic character of polysaccharides. Carboxymethylation, which can endow macromolecule with outstanding physical and chemical properties, is an important way to introduce carboxyl groups into biopolymers. For example, carboxycelluloses are important derivatives of natural cellulose polymers, and they have been widely used in many biomedical, agricultural, and wastewater treatment field applications [Citation112,Citation113]. It is well known that the properties (viscosity of solution, film forming, interaction with cations, and the formation of supramolecular aggregates, etc.) are mainly determined by the total DS, i.e., the average number of carboxymethyl groups in the polymer [Citation107].
There are many kinds of methods to measure the DS of carboxymethyl polymers in direct or indirect way, including the ashing method [Citation108,Citation109], acid washing method [Citation110–117], spectrophotometry [Citation118], complexometry [Citation119], NMR method [Citation120,Citation121], Zeisel-LC [Citation122], etc [Citation123–127]. In industry the DS is usually determined by titrimetric methods [Citation128]. All of first four methods belong to this. Over the years, aiming at the problem of time-consuming, poor stability and testing effectiveness in classical complexometry, many other improvements have been made by Huang [Citation129], including the replacement for murexide indicator with PAN indicator (1-(2-pyridinylazo)-2-naphthalenol) and adjusting the original pH (7.5–8.0) to slightly acidic (6.0–7.0) for inhibition of precipitation of Cu(OH)2. Like acid–base back titration, this is also one of most popular ways of determining the DS of carboxymethyl ethers. Moreover, the substitution degrees of carboxymethyl groups were further calculated and obtained from their respective [Citation1]H NMR spectra. Due to the protons of the methylene on the carboxymethyl group, a new proton signal appeared in the NMR spectra of all carboxymethyl ethers. Besides, the peak area of the aforementioned new appeared NMR signal turns larger and larger with increasing amount of monochloroacetic acid fed in the preparation process. The contents of carboxymethyl group were calculated on the basis of the integrated areas of the corresponding characteristic peaks in the [Citation1]H NMR spectra (Equationequation 8(8)
(8) ). Where A is peak areas; N is the number of protons; Signal is assigned to the methene (-CH2COOH); AGU is the proton of single hydrogen of an anhydroglucose unit. In addition, the extent of functionalization (degree of substitution) in chitosan derivatives was quantitatively assessed using the elemental analyses of burnable. The degrees of substitution (DS) of carboxymethyl chitosan derivatives were calculated on the basis of the percentages of carbon and nitrogen [Citation130].
4.2. Cyanoethyl ether
Cyanoethylation of biopolymers such as chitosan [Citation111–132], guaran [Citation133], starch [Citation134–136], bagasse [Citation137], cotton [Citation138], cellulose [Citation139,Citation140] and others [Citation141–143] can be performed by reacting starch with acrylonitrile using Michael addition. The introduction of cyano group into the polysaccharides has for long been used to improve properties of polymers. Take starch, for example, this treatment gives the starch a resistance to biodegradation, a good water solubility, a thick paste in water and adhesive properties [Citation144]. Similarly, the DS has an effect on physicochemical properties of cyanoethyl polysaccharides [Citation111–143]. The extent of cyanoethylation was determined by using the Kjeldahl method of nitrogen determination [Citation136]. The degree of substitution (DS) was calculated by C/N ratio of elemental analysis [Citation145–147]. Due to the methylene linked to nitrile group from cyanoethyl polysaccharides, [Citation1]H-NMR could be employed for further confirmation of the DS [Citation111–148].
4.3. Cationic ether
Cationic polysaccharides are generally synthesized by the reaction of polysaccharides and cationic reaction reagents such as tertiary amine compounds, quaternary amine compounds, and imine compounds. Take the starch for instance. Tertiary amine ether starch and quaternary ammonium ether starch are the main commodities starch. They are non-toxic and easily biodegradable. Introducing a cationic group to the starch gives good mineral binding properties; they are widely used in the paper industry, where they are mainly used as a flocculation, dispersion and ink fixing agent [Citation149]. Thus, the DS is an important parameter [Citation150]. The degree of substitution (DS) was calculated from the nitrogen content which was estimated by the micro Kjeldahl method after purification (equation 9) [Citation116–129,Citation132–151]. Where N% is the content of nitrogen measured by Kjeldahl; the values 14, 162.14 and M in equation equal the molecular weight of Nitrogen element, AGU and cationic ether. There are significant parallels with chitosan, cyanoethyl ether, and other nitrogenous compounds.
5. Mixed ethers
Over recent years, for the purpose of better performance and more widespread application, considerable attention has been focused upon the treatment and disposal of the modification of polysaccharides. However, it is difficult to meet the market demand by using a single modification process. There has been a trend to combine different kinds of chemical treatments to create new kinds of modifications. For the moment, more and more often, the polysaccharides modification processes are complex, i.e., combine two reagents or two methods. The physicochemical properties of chemically modified polysaccharides depend on various factors, primarily on the type of modifying agent, the conditions of reaction, and the kind of polysaccharides, as well as the value of DS/MS. To evaluate on the DS/MS, of course, calculate separately on each of the substituent groups. For example, acetylated oxidised starch is one obtained by dual chemical modification [Citation152]. Assessment of the effectiveness of oxidation and acetylation was based on the increase in the contents of carboxyl groups and carbonyl groups [Citation153] and acetyl groups [Citation154] in starch. Beyond that, there are several composite modified polysaccharides, such as acetylation in hydroxypropyl chitosan [Citation155], hydroxypropyl methylcellulose [Citation156–160].
6. Conclusions
Polysaccharides are generally undergoing the modification with the aim of satisfying their physicochemical properties to the requirements of the technological processes in which they will be utilized as additives only to ensure the appropriate structural performance and storage stability of the final product. The increasing industrial importance of polysaccharides ethers has aroused interest in methods for their analysis. The value of DS/MS markedly affects the properties of these compounds and the suitable methods for their determination are of great necessity. In conjunction with the research mentioned previously, the aim of this article is to summarize the chemical modification of polysaccharides with etherification, and the measurement processes of DS/MS and associated mechanisms are involved. We look forward to seeing that this review could give a summarization and prospect on analytical method of DS/MS for etherified polysaccharide derivatives and wishing to help encourage further research on new methods of analysis and modification.
Acknowledgments
This work was supported by the Industry-university-research projects (HX2020188, HX2020208), Post-doctoral Program of Anhui Province (2020B406), Anhui Provincial Natural Science Foundation (2108085QB53).
Disclosure statement
No potential conflict of interest was reported by the author(s).
Additional information
Funding
References
- Xu JT, Andrews T, Shi YC. Recent advances in the preparation and characterization of intermediately to highly esterified and etherified starches: a review. Starch. 2020;72(3–4):1900238.
- Gilet A, Quettier C, Wiatz V, et al. Unconventional media and technologies for starch etherification and esterification. Green Chem. 2018;20:1152–1168.
- Treib J, Baron J-F, Grauer MT, et al. An international view of hydroxyethyl starches. Intens Care Med. 1999;25:258–268.
- Spychaj T, Wilpiszewska K, Zdanowicz M. Medium and high substituted carboxymethyl starch: synthesis, characterization and application. Starch. 2013;65:22–33.
- Li JJ, Wu XY, Shi QF, et al. Effects of hydroxybutyl chitosan on improving immunocompetence and antibacterial activities. Mat Sci Eng C. 2019;105:110086.
- Golachowski A, Zięba T, Kapelko-Żeberska M, et al. Current research addressing starch acetylation. Food Chem. 2015;176:350–356.
- Azeredo H, Waldron KW. Crosslinking in polysaccharide and protein films and coatings for food contact-A review. Trends Food Sci. Tech. 2016;52:109–122.
- Vanier NL, Halal SLME, Dias ARG, et al. Molecular structure, functionality and applications of oxidized starches: A review. Food Chem. 2017;221:1546–1559.
- Palme A, Theliander H, Brelid H. Acid hydrolysis of cellulosic fibres: Comparison of bleached kraft pulp, dissolving pulps and cotton textile cellulose. Carbohyd. Polym. 2016;136:1281–1287.
- Poshina DN, Rail SV, Poshin AN, et al. Accessibility of chitin and chitosan in enzymatic hydrolysis: a review. Polym Degrad Stabil. 2018;156:269–278.
- Pal J, Singhal RS, Kulkarni PR. Physicochemical properties of hydroxypropyl derivative from corn and amaranth starch. Carbohyd Polym. 2002;48:49–53.
- Khalil MI, Farag S, Hashem A. Preparation and characterization of some cationic starches. Starch. 2010;45(6):226–231.
- Rosu AM, Rafin C, Surpateanu G, et al. Synthesis of alkylated potato starch derivatives and their potential in the aqueous solubilization of benzo[a]pyrene. Carbohyd Polym. 2013;93:184–190.
- Thyes C, Madjdpour C, Frascarolo P, et al. Effect of High- and Low-molecular-weight Low-substituted Hydroxyethyl Starch on Blood Coagulation during Acute Normovolemic Hemodilution in Pigs. Anesthesiology. 2006;105(6):1228–1237.
- Wang YW, Li AM, Yang H. Effects of substitution degree and molecular weight of carboxymethyl starch on its scale inhibition. Desalination. 2017;408:60–69.
- Singh J, Kaur L, McCarthy OJ. Factors influencing the physico-chemical, morphological, thermal and rheological properties of some chemically modified starches for food applications-a review. Food Hydrocolloid. 2007;21(1):1–22.
- Zhang GX, She Y, You YQ, et al. The application of an advanced visualized method in synthesis process optimization of carboxymethyl hydroxyethyl starch. Carbohyd Polym. 2010;79:673–676.
- Wang YF, Zhang L, Liu HS, et al. Relationship between morphologies and mechanical properties of hydroxypropyl methylcellulose/hydroxypropyl starch blends. Carbohyd Polym. 2016;153:329–335.
- B O. Wurzburg. In: Whistler RL, editor. Methods in Carbohydrate Chemistry. New York: Academic Press; 1964. p. 286–288.
- Wurzburg OB, Wurzburg OB, Ed. Modified starches: properties and uses. Boca Raton Fl: CRC Press; 1986. p. 41–53.
- Tharanathan RN. Starch — value addition by modification. Crit Rev Food Sci Nutr. 2005;45:371–384.
- Mischnick P, Momcilovic D. Chemical Structure Analysis of Starch and Cellulose Derivatives. Adv Carbohyd Chem Biochem. 2010;64:117–210.
- BeMiller JN. Anais brasileiros de dermatologia. Carbohyd. Polym. 2011;86:386–423.
- Zia-ud-din HGX, Fei P. Physical and chemical modification of starches: a review. Crit Rev Food Sci Nutr. 2017;57:2691–2705.
- Yang X, Li AQ, Li XX, et al. An overview of classifications, properties of food polysaccharides and their links to applications in improving food textures. Trends Food Sci Tech. 2020;102:1–15.
- Morgan PW. Determination of ethers and esters of ethylene glycol. a modified alkogyl analysis. Ind Eng Chem Anal Ed. 1946;18:500–504.
- Cobler JG, Samsel EP, Beaver GH. Yao Xue Xue Bao = Acta Pharmaceutica Sinica. Talanta. 1962;9(6):473–481.
- Ho FFL, Kohler RR, Ward GA. Ukrains’kyi biokhimichnyi zhurnal. Anal. Chem. 1972;44(1):178–181.
- Xu A, Seib PA. Determination of the level and position of substitution in hydroxypropylated starch by high-resolution1h-NMR spectroscopy of alpha-limit dextrins. J Cereal Sci. 1997;25:17–26.
- Fu Z, Zhang L, Ren MH, et al. Developments in hydroxypropylation of starch: a review. Starch. 2019;71:1800167.
- Koda S, Hori T, Nomura H. Hydrophobic hydration of hydroxyalkyl cellulose. Polymer. 1992;33(15):3234–3238.
- Cumpstey I. Chemical modification of polysaccharides. ISRN Org Chem. 2013;417672.
- Funke U, Lindhauer MG. Effect of Reaction Conditions and Alkyl Chain Lengths on the Properties of Hydroxyalkyl Starch Ethers. Starch. 2001;53:547–554.
- Glover PA, Rudloff E, Kirby R. Hydroxyethyl starch: a review of pharmacokinetics, pharmacodynamics, current products, and potential clinical risks, benefits, and use. J Vet Emerg Crit Care. 2015;24:642–661.
- Roten IV, Madjdpour C, Frascarolo P, et al. Molar substitution and C2/C6 ratio of hydroxyethyl starch: influence on blood coagulation. Brit J Anaesth. 2006;96:455–463.
- Werner HW, Mitchell JL. Determination of Monoalkyl Ethers of Ethylene Glycol. Ind Eng Chem Anal Ed. 1943;15:375–376.
- Zeisel S. Uber ein Verfahren zum quantitativen Nachweis von Methoxyl. Monatsh Chem. 1885;6:989–996.
- Mathew S, Abraham TE. The Journal of the Kentucky Medical Association. Food Chem. 2007;105:579–589.
- Samsel E, McHard J. Ind. Eng Chem Anal Ed. 1942;14:750–754.
- Lortz HJ. Determination of Hydroxyalkyl Groups in Low-Substituted Starch Ethers. Anal. Chem. 1956;28:892–895.
- Hodges KL, Kester WE, Wiederrich DL, et al. Determination of alkoxyl substitution in cellulose ethers by Zeisel gas chromatography. Anal. Chem. 1979;51:2172–2176.
- Tai H, Powers RM, Protzman TF. Determination of Hydroxyethyl Group in Hydroxyethyl Starch by Pyrolysis-Gas Chromatographic Technique. Anal Chem. 1964;36:108–110.
- Fishbein L Jr., Zielinski WL. Gas chromatography of hydroxyethyl derivatives. J Chromatogr A. 1967;28:418–421.
- Hodges JW, Merkus HG, Galan LD. Application of alkali ions in chemical ionization mass spectrometry. Anal Chem. 1976;48:1557–1562.
- Lee YC, Baaske DM, Carter JE. British Journal of Urology. Anal. Chem. 1983;55:334–338.
- Shang C, Hua YQ, Zhang L. Determination of molar substitution of hydroxyethyl starch 130 injection with GC and chemical method. J Shenyang Pharm Univ. 2009;26:559–563.
- Du ZH, Ren CJ, Yu P,et al. Study on the determination of molar substitution ratio of hydroxyethyl starch by GC. Qilu Pharm Affair. 2012;31:92–94.
- Wang J, Pan XY. Improved Method for Substitution Determination of Hydroxyethyl Starch 200/0.5 and Sodium Chloride Injections. China Pharm. 2013;16:1836–1838.
- Kuzmina NE, Moiseev SV, Krylov VI, et al. Modification of PMR spectroscopy technique for determination of the molar substitution in hydroxyethyl starch. Pharm Chem J. 2015;49:559–563.
- King AWT, Jalomäki J, Granström M, et al. A new method for rapid degree of substitution and purity determination of chloroform-soluble cellulose esters, using 31P NMR. Anal Methods. 2010;2:1499–1505.
- Treib J, Haass A, Pindur G, et al. All medium starches are not the same: influence of the degree of hydroxyethyl substitution of hydroxyethyl starch on plasma volume, hemorrheologic conditions, and coagulation. Transfusion. 1996;36:450–455.
- Hitosugi T, Saito T, Suzuki S, et al. Anesthesia and Analgesia. Anesth Analg. 2007;105:724–728.
- Westphal M, James MFM, Kozek-Langenecker S, et al. Hydroxyethyl starches. Anesthesiology. 2009;111:187–202.
- Ozaki T, Tada M, Irikura T. A study on the distribution of hydroxyethyl groups in hydroxyethyl-starch. Yakugaku Zasshi-J Pharm Soc Japan. 1972;92:1500–1506.
- Merkus HG, Mourits JW, Galan LD, et al. Substituent distribution in hydroxyethyl starch. Starch. 1977;29:406–409.
- Arisz PW, Thai HTT, Boon JJ. Changes in substituent distribution patterns during the conversion of cellulose toO-(2-hydroxyethyl) celluloses. Cellulose. 1996;3:45–61.
- Zhong S, Zhang SF, Ma W, et al. Hydroxyethyl starch with high surface activity via ethylene carbonate functionalization. J Appl Polym Sci. 2019;137:48807.
- Belder AND, Norrman B. The substitution patterns of O-(2-hydroxyethyl) starch and O-(2-hydroxyethyl) dextran. Carbohyd. Res. 1969;10:391–394.
- Peng YF, Han BQ, Liu WS, et al. Preparation and antimicrobial activity of hydroxypropyl chitosan. Carbohydr Res. 2005;340:1846–1851.
- Cheng ZH, Huang J, Hu S, et al. Effects of hydroxypropyl degree on physiochemical activities of chitosan from squid pens. Int J Biol Macromol. 2014;65:246–251.
- Fan LH, Zou SQ, Ge HY, et al. Preparation and characterization of hydroxypropyl chitosan modified with collagen peptide. Int J Biol Macromol. 2016;93:636–643.
- Lee HL, Yoo B. Effect of hydroxypropylation on physical and rheological properties of sweet potato starch. LWT - Food Sci. Technol. 2011;44:765–770.
- Senanayake S, Gunaratne A, Ranaweera KKDS, et al. Effect of Hydroxypropylation on Functional Properties of Different Cultivars of Sweet Potato Starch in Sri Lanka. Int J Food Sci. 2014:148982.
- Wan T, Liu LY, Zeng W, et al. Studies on surface hydroxypropylation and swellability of bacterial cellulose. Adv Mater Res. 2013;601:12–15.
- Chen CT, Huang Y, Zhu CL, et al. Synthesis and characterization of hydroxypropyl cellulose from bacterial cellulose. Chinese J Polym Sci. 2014;32:439–448.
- Liu W, Xie ZH, Zhang BC, et al. Effects of hydroxypropylation on the functional properties of psyllium. J Agr Food Chem. 2010;58:1615–1621.
- Liu W, Xu J, Jing P, et al. Preparation of a hydroxypropyl ganoderma lucidum polysaccharide and its physicochemical properties. Food Chem. 2010;122:965–971.
- Dias FF, Tekchandani HK, Mehta D. Modified starches and their use by food industries. Food Chem. 1997;64:361–375.
- Johnson DP. Bulletin of the World Health Organization. Anal. Chem. 1969;41:859–860.
- Chun EH, Oh SM, Kim HY, et al. Effect of high hydrostatic pressure treatment on conventional hydroxypropylation of maize starch. Carbohydr Polym. 2016;146:328–336.
- Jones LR, Riddick JA. Colorimetric determination of 1,2-propanediol and related compounds. Anal Chem. 1957;29:1214–1216.
- Jones LR, Riddick JA. Colorimetric determination of propionaldehyde. Anal Chem. 1954;26:1035–1038.
- Zhao K, Liu LY, Liu JT. Spectrophotometric Determination of Degree of Substitution of Hydroxypropyl Starch. Food Sci. 2011;32:201–203.
- ASTM. Annual book of ASTM standard. standard methods of testing hydroxyethyl cellulose. West Conshohocken PA: ASTM International; 1976. p. 276–283.
- Wang M. Determination of low substitution degree of hydroxypropyl starch and hydroxypropyl crosslinking. Chinese J Anal Chem. 1982;10:432–434.
- Xiang KH, Yu L. Determination on Substitution Degree of Hydroxypropyl Starch Ether. China Surfact Det Cosmet. 1990;1:31–33.
- Van Der Bij VJR, Vogel WF. Infrarotspektroskopie von einigen Stärkederivaten. Starch. 1962;14:113–118.
- Gnanasambandam R, Proctor A. Determination of pectin degree of esterification by diffuse reflectance Fourier transforms infrared spectroscopy. Food Chem. 2000;68:327–332.
- Forrest B, Cove L. Identification and quantitation of hydroxypropylation of starch by FTIR. Starch. 1992;44:179–183.
- Stahl H, McNaught RP. A rapid nuclear magnetic resonance method for determining hydroxypropyl group in modified starch. Cereal Chem. 1970;47:345–350.
- Graaf RAD, Lammers G, Janssen LPBM, et al. Quantitative analysis of chemically modified starches by 1H-NMR spectroscopy. Starch. 1995;47(12):469–475.
- Yang XJ, Zhi ZH, Lu LD. Determination of the Molar Substitution of Hydroxypropyl Celluloses by an NMR Method. Spectrosc. Lett. 1998;31:1279–1285.
- Villwock VK, BeMiller JN. Effects of salts on the reaction of normal corn starch with propylene oxide. Starch. 2005;57:281–290.
- Sc B, Östergård K, Björck II, et al. A study of native and chemically modified potato starch. part i: analysis and enzymic availability in vitro. Starch. 1988;40:58–66.
- Han JA, BeMiller JN. Influence of reaction conditions on MS values and physical properties of waxy maize starch derivatized by reaction with propylene oxide. Carbohyd. Polym. 2006;64:158–162.
- Lawal OS. Starch hydroxyalkylation: Physicochemical properties and enzymatic digestibility of native and hydroxypropylated finger millet (Eleusine coracana) starch. Food Hydrocolloid. 2009;23:415–425.
- Leegwater DC, Marsman JW, A. Analysis of an acid hydrolysate of a low-substituted hydroxypropyl starch. Starch. 1973;25:142–145.
- Gidley MJ. American Journal of Diseases of Children (1960). Carbohyd. Res. 1985;139:85–93.
- Richardson S, Nilsson GS, Bergquist KE, et al. Characterisation of the substituent distribution in hydroxypropylated potato amylopectin starch. Carbohydr Res. 2000;328:365–373.
- Zhao JW, Chen ZH, Jin ZY, et al. Level and position of substituents in cross-linked and hydroxypropylated sweet potato starches using nuclear magnetic resonance spectroscopy. Carbohyd Polym. 2015;131:424–431.
- Belder A. N. D., Norrman, B. The substitution patterns of O-(2-hydroxyethyl) starch and O-(2-hydroxyethyl) dextran. Carbohyd. Res. 1969;10:391–394.
- Liu XL, Zhang K, Hu Y, et al. Determination of molar substitution of γ-hydroxypropyl starch. Starch - Stärke. 2020;73:2000013.
- Zhang KH, Qian YF, Wang HS, et al. Electrospun silk fibroin–hydroxybutyl chitosan nanofibrous scaffolds to biomimic extracellular matrix. J Biomat Sci. 2011;22:1069–1082.
- Wang QQ, Kong M, An Y, et al. Hydroxybutyl chitosan thermo-sensitive hydrogel: a potential drug delivery system. J Biomat Sci. 2013;48:5614–5623.
- Li JJ, Wu XY, Shi QS, et al. Effects of hydroxybutyl chitosan on improving immunocompetence and antibacterial activities. Mat Sci Eng C. 2019;105:110086.
- Sun MJ, Wang T, Pang JH, et al. Hydroxybutyl chitosan centered biocomposites for potential curative applications: a critical review. Biomacromolecules. 2020;21:1351–1367.
- Wokadala OC, Emmambux NM, Ray SS. Inducing PLA/starch compatibility through butyl-etherification of waxy and high amylose starch. Carbohyd Polym. 2014;112:216–224.
- Dong ST, Li S, Hao YC, et al. Hydroxybutyl starch-based thermosensitive hydrogel for protein separation. Int J Biol Macromol. 2019;134:165–171.
- Chen Y, Hao YC, Li S, et al. Preparation of hydroxybutyl starch with a high degree of substitution and its application in temperature-sensitive hydrogels. Food Chem. 2021;355:129472.
- Harry-O’Kuru RE, Moser KB, Gordon SH. Colorimetric determination of the molar substitution of 2-hydroxybutyl and related starch ethers. Carbohydr Polym. 1992;17:313–318.
- Man XL, Peng WK, Chen J, et al. Analysis of molar substitution of hydroxybutyl group by zeisel reaction in starch ethers. Molecules. 2021;26:5509.
- Takaragi A, Minoda M, Miyamoto T, et al. Reaction characteristics of cellulose in the LiCl/1,3‐dimethyl‐2‐imidazolidinone solvent system. Cellulose. 1999;6:93–102.
- Bemiller JN, Wing RE. Methyl terminal-4-O-methylmalto-oligosaccharides. Carbohyd. Res. 1968;6:197–206.
- Teramoto N, Motoyama T, Yosomiya R, et al. Synthesis and properties of thermoplastic propyl-etherified amylose. Eur Polym J. 2002;38:1365–1369.
- Umemura T, Hirakawa M, Yoshida Y, et al. Quantitative protection of chitin by one-step tritylation and benzylation to synthesize precursors for chemical modifications. Polym Bull. 2012;69:303–312.
- Akopova TA, Demina TS, Cherkaev GV, et al. Solvent-free synthesis and characterization of allyl chitosan derivatives. RSC Adv. 2019;9:20968–20975.
- Shibata M, Nozawa R, Teramoto N, et al. Synthesis and properties of etherified pullulans. Eur Polym J. 2002;38:497–501.
- Cheronis ND, Ma TS. Organic functional group analysis by micro and semimicro methods. New York: Wiley; 1964.
- Yin ZH, Zhang YQ, Xie XL. Synthesis and properties of hexadecyl starch ether. Textile Auxiliaries. 2013;30:9–12.
- Kurita K, Ishii S, Tomita K, et al. Reactivity characteristics of squid β-chitin as compared with those of shrimp chitin: high potentials of squid chitin as a starting material for facile chemical modifications. J Polym Sci Part A: Polym Chem. 1994;32:1027–1032.
- Dong YM, Yuan Q, Wu YS, et al. Studies on the effect of substitution degree on the liquid crystalline behavior of cyanoethyl chitosan. J Appl Polym Sci. 2015;76:2057–2061.
- Sharma PR, Varma AJ. Functional nanoparticles obtained from cellulose: engineering the shape and size of 6-carboxy cellulose. Chem Commun. 2013;49:8818.
- Sharma PR, Joshi R, Sharma SK, et al. A simple approach to prepare carboxy cellulose nanofibers from untreated biomass. Biomacromolecules. 2017;18:2333–2342.
- Heinze T, Liebert T, Heinze U, et al. Starch derivatives of high degree of functionalization 9: carboxymethyl starches. Cellulose. 2004;11:239–245.
- Aggeryd I, K. Olin. Determination of the degree of substitution of sodium carboxymethylcellulose by potentiometric titration and use of the extended Henderson-hasselbalch equation and the simplex method for the evaluation. Talanta. 1985;32:645–649.
- Zhong XX. Improvement of the Ashing Method to Determine the Degree of Carboxymethyl Substitution of Carboxymethyl Chitosan. J Shaoyang Univ. (Sci. Technol.). 2006;3:88–90.
- Daul GC, Reinhardt RM, Reid JD. Preparation of soluble yarns by the carboxymethylation of cotton. Text Res J. 1953;23:719–726.
- El-Sheikh MA. International Journal of Radiation Oncology, Biology, Physics. Carbohyd. Polym. 2010;79:875–881.
- Li SF, Mujyambere JMV, Liu M. Synthesis of Carboxymethyl Starch with High Degree of Substitution by a Modified Dry Process. Adv Mat Res. 2011;233-235:306–310.
- Balsamo V, López-Carrasquero F, Laredo E, et al. Preparation and thermal stability of carboxymethyl starch/quaternary ammonium salts complexes. Carbohyd Polym. 2011;83:1680–1689.
- Zhang H, Wang JK, Liu WJ, et al. Microwave-assisted synthesis, characterization, and textile sizing property of carboxymethyl corn starch. Fiber Polym. 2015;16:2308–2317.
- Xu R, Wang CY, Gu HF, et al. Determination of Sodium CarboxymethylCellulosein Papers by Spectrophotometric. China Pulp & Paper. 2011;30:39–42.
- Sharma PR, Chattopadhyay A, Sharma SK, et al. Effcient removal of UO22+from water using carboxycellulose nanofibers prepared by the Nitro-Oxidation method. Ind Eng Chem Res. 2017;56:13885–13893.
- Li HJ, Cai T, Yuan B, et al. Flocculation of both kaolin and hematite suspensions using the starch-based flocculants and their floc properties. Ind Eng Chem Res. 2015;54:59–67.
- Wang YW, Li AM, Yang H. Effects of substitution degree and molecular weight of carboxymethyl starch on its scale inhibition. Desalination. 2017;408:60–69.
- Miller TG, Hronek RJ. Determination of Carboxymethyl Substitution in Cellulose Ethers by Zeisel Reaction and Liquid Chromatography. Anal. Chem. 1985;57:2091–2093.
- Dong YM, Mei XF, Yuan Q, et al. Determination of Degree of Substitution for Acylated Chitosan and Cyanoethyl Chitosan Using FTIR. J XiaMen Univ (Nat Sci). 2002;41:44–49.
- Muzzarelli RAA, Tanfani F, Emanuelli M, et al. N-(carboxymethylidene)chitosans and N-(carboxymethyl)chitosans: Novel chelating polyampholytes obtained from chitosan glyoxylate. Carbohyd. Res. 1982;107:199–214.
- Chen HF, Pan SR, Hu Y, et al. Determination of Substitution Degree of Carboxymethyl Chitosan by Colloid Titration. J Inst Anal. 2003;22:70–73.
- Liu SP, Chen S, Liu ZF, et al. Resonance Rayleigh scattering spectra of interaction of sodium carboxymethylcellulose with cationic acridine dyes and their analytical applications. Anal Chim Acta. 2005;535:169–175.
- Liu J, Chen J, Dong N, et al. Food Chem. The Journal of the Acoustical Society of America. 2012;132:2224–2230.
- Tōei K, Sawada M. A turbidimetric method for colloid titrations. Anal Chim Acta. 1977;89:383–389.
- Huang J, Pan LJ, Zheng Z, et al. A rapid and accurate method of determinating substitution degree of carboxymethyl starch. Sci. Technol. Food Ind. 2003;24:82–84.
- Sun XQ, Zhang JJ, Chen Y, et al. Synthesis, Characterization, and the Antioxidant Activity of Carboxymethyl Chitosan Derivatives Containing Thiourea Salts. Polymers. 2019;11:1810.
- Rathinam S, Ólafsdóttir S, Jónsdóttir S, et al. Selective synthesis of N,N,N-trimethylated chitosan derivatives at different degree of substitution and investigation of structure-activity relationship for activity against P. aeruginosa and MRSA. Int J Biol Macromol. 2020;160:548–557.
- Abou-Zeid NY, Waly AI, Kandile NG, et al. Preparation, characterization and antibacterial properties of cyanoethylchitosan/cellulose acetate polymer blended films. Carbohyd Polym. 2011;84:223–230.
- Ragheb A, Abd El-thalouth I, Abou Amer M, et al. Chemical Modification of Guaran Gum. Part 2: cyanoethylation. Starch. 1993;45:244–249.
- Hebeish A, Khalil MI. Characterization of the Reaction Products for Starch and Acrylonitrile. Starch. 1988;40:104–107.
- Nwokocha L. Adhesive Properties of Cyanoethyl Starch. J Adhes Sci Technol. 2011;25:893–902.
- Zhu ZF, Chen XY, Wang M, et al. Toughened cyanoethyl starch by blending with poly (methyl acrylate-co-acrylic acid-co-acrylonitrile) latex for warp sizing. Indian J Fibre Text Res. 2017;42:100–106.
- Hassan ML, El-Wakil NA, Sefain MZ. Thermoplasticization of bagasse by cyanoethylation. J Appl Polym Sci. 2001;79:1965–1978.
- Daul GC, Reinhardt RM, Reid JD. The preparation of partially cyanoethylated cotton with acrylonitrile. Textile Res. J. 1955;25:246–253.
- Khalil EMA, El-Wakil NA. Infrared absorption spectra of cyanoethylated cellulose fibres. Cell Chem Technol. 2000;34:473–479.
- Zhou J, Li Q, Song Y, et al. A facile method for the homogeneous synthesis of cyanoethyl cellulose in NaOH/urea aqueous solutions. Polym Chem. 2010;1:1662–1668.
- Verraest DL, da Silva LP, Peters JA, et al. Synthesis of Cyanoethyl Inulin, Aminopropyl Inulin and Carboxyethyl Inulin. Starch. 1996;48:191–195.
- Sharma BR, Kumar V, Soni PL. Cyanoethylation ofCassia tora Gum. Starch. 2003;55:38–42.
- Fiege K, Luensdorf H, Atarijabarzadeh S, et al. Cyanoethylation of the glucans dextran and pullulan: substitution pattern and formation of nanostructures and entrapment of magnetic nanoparticles. Beilstein J Org Chem. 2012;8:551–566.
- Guarás MP, Ludueña LN, Alvarez VA. Starch-based materials in food packaging. Development of Biodegradable Products from Modified Starches. 2017:77–124.
- Morooka T, Norimoto M, Yamada T. Cyanoethylated cellulose prepared by homogeneous reaction in paraformaldehyde-DMSO system. J Appl Polym Sci. 1986;32:3575–3587.
- Okalebo JR, Gathua KW, Woomer PI. Laboratory methods of soil and plant analysis: a working manual, 2nd Edition. Nairobi Kenya: Tropical Soil Fertility and Programme. 2002.
- Joshi G, Naithani S, Varshney VK, et al. Potential use of waste paper for the synthesis of cyanoethyl cellulose: a cleaner production approach towards sustainable environment management. J Clean Prod. 2017;142:3759–3768.
- Cao XF, Sun SN, Peng XW, et al. Synthesis and characterization of cyanoethyl hemicelluloses and their hydrated products. Cellulose. 2013;20:291–301.
- Sharma M, Aguado R, Murtinho D, et al. A review on cationic starch and nanocellulose as paper coating components. Int J Biol Macromol. 2020;162:578–598.
- Liu QX, Li JL, Xu WC. Preparation and Properties of Cationic Starch with High Degree of Substitution. Mat Sci Forum. 2011;663-665:1264–1267.
- Su YT, Du HY, Huo YQ, et al. Characterization of cationic starch flocculants synthesized by dry process with ball milling activating method. Int J Biol Macromol. 2016;87:34–40.
- Pietrzyk S, Juszczak L, Fortuna T, et al. Effect of the oxidation level of corn starch on its acetylation and physicochemical and rheological properties. J Food Eng. 2014;120:50–56.
- Wang YJ, Wang LF. Physicochemical properties of common and waxy corn starches oxidized by different levels of sodium hypochlorite. Carbohyd Polym. 2003;52:207–217.
- Zhao K, Li B, Xu MJ, et al. Microwave pretreated esterification improved the substitution degree, structural and physicochemical properties of potato starch esters. LWT-Food Sci Technol. 2018;90:116–123.
- Wang PB, Lv XS, Zhang BQ, et al. Simultaneous determination of molar degree of substitution and its distribution fraction, degree of acetylation in hydroxypropyl chitosan by 1H NMR spectroscopy. Carbohydrate Polymers. 2021;263:117950.
- Zhang L, Wang YF, Yu L, et al. Rheological and gel properties of hydroxypropyl methylcellulose/hydroxypropyl starch blends. Colloid Polym Sci. 2015;293:229–237.