Abstract
In this study, to find the best location to cultivate the trees for river bank protection, some experimental tests were performed. One row of trees were cultivated at five different distances from the main channel/floodplain interface in a rectangular compound open channel. In experimental model for simulating the trees, some cylindrical wood rods were attached to the bed of flood plain. The three-dimensional flow velocity of flow in a cross-section between the trees were measured in 149 nodal points using Micro-acoustic Doppler velocimeter (ADV) velocity meter. At each nodal point, 6000 velocity data for each direction were stored in a computer. From the analysis of the experimental data, it was concluded that upon cultivating trees at 10% distance of flood plain width from the edge of main channel, the minimum streamwise velocity occurred near main channel/floodplain interface, while the maximum streamwise velocity occurred far from the edge of floodplain. Therefore, the best location for cultivating the trees was found to be at 10% of floodplain width and it is suggested for river bank protection in practical applications.
1 Introduction
Many rivers and artificial channels consist of a deep main channel with one or two floodplains at the sides (Myers Citation1978). Despite many previous studies in compound channel, there are still some different issues to investigate in compound open channels. The studies such as Myers and Elsawy Citation(1975), Myers Citation(1978), Knight and Demetriou Citation(1983) Knight and Hamed Citation(1984) and Christodoulou Citation(1992) have been performed to propose a formula for determining apparent shear stress on the vertical interface between main channel and floodplain. Myers Citation(1978) found that conventional techniques for determining discharge using an imaginary wall at main channel/floodplain interface could be justified only at considerable floodplain depth. Ozbek et al. Citation(2004) performed some experiments in a symmetrical compound channel and assumed three different imaginary interface planes (horizontal, vertical and diagonal) between main channel and floodplain for computing apparent shear stress and discharge. Yang et al. Citation(2007b) performed some experiments in rough main channel and floodplain and found that diagonal, vertical and horizontal division methods have high amount of errors in computing the composite roughness in compound channel.
Sellin Citation(1964), Zheleznyakov Citation(1971), Rajaratnam and Ahmadi Citation(1981), Zarrati et al. Citation(2008), Shiono and Knight Citation(1991), Huthoff et al. Citation(2008) and Hua et al. Citation(2007) have investigated vortices and momentum transfer in main channel and floodplain interface and found that due to formation of vortices at the interface momentum, transfer occurs at the interface and between two sections. Longitudinal and horizontal eddies that generate at the edge of the floodplain are introduced by Huthoff et al. Citation(2008). Knight and Demetriou Citation(1983) derived equations for momentum transfer in lateral and vertical directions within the cross-section using experimental data and they found that for compound channels with low relative depths and high flood plain widths, apparent shear force increases on floodplain/main channel boundary wall. Myers and Brennan Citation(1990) showed that momentum transfer mechanism increases main channel resistance coefficient and decrease this parameter in floodplain. Christodoulou Citation(1992) proposed a formula for determining apparent shear stress on the vertical interface between main channel and floodplain. Bousmar and Zech Citation(1999) presented a model for compound channel considering momentum transfer between main channel and floodplains and found a good agreement between the experimental data and the model.
Numerical modelling of flow structure in vegetated compound channel is another important issue in compound open channel. To simulate mean flow and turbulence structure in compound open channel with vegetation on floodplain, many investigators (Tsujimoto et al. Citation1991, Citation1992, Shimizu and Tsujimoto Citation1994, Fischer-Antze et al. Citation2001, Helmio Citation2002, Kang and Choi Citation2005, Wilson et al. Citation2006, Joung and Choi, Citation2008) developed some numerical model to compute velocity distributions in channels with submerged vegetation. The results were in agreement with measured velocity profiles.
To investigate resistance characteristics of in-bank and over-bank flows, more recently Yang et al. Citation(2007a) examined the influence of different types of vegetation on flow structure especially at the edge of the floodplain and showed that characteristics of flow such as secondary currents and lateral velocity distribution are very complex at the edge of the floodplain in vegetated compound open channel.
Therefore, the main purpose of this study is to find how far the trees must be cultivated from the edge of flood plain to protect river banks and flood plain edges from scouring. In addition, at the cultivating point, trees must be having enough stability. For more importance of the problem, see . Therefore to find the best location for the tree cultivation, a series of experimental study was carried out to investigate the effect of cultivating trees at different distances from the main channel/floodplain boundary and the results are presented herein.
2 Materials and method
The experimental tests in this study were conducted in a laboratory flume with 15 m length, 0.7 m width and 0.6 m heigth which was built in hydraulic laboratory of water engineering department, Shiraz University, Shiraz, Iran. The compound open channel cross-section consists of a main channel with bed width equal 0.30 m and depth equal 0.15 m and two symmetrical flood plain of 0.20 m width (b). For simulating the trees in the experimental tests, the cylindrical wood rods with 12 cm height and 8 mm diameter were glued with 8 cm distance (TS) on the floodplain as shown in . To model the trees in the experimental tests, the cylindrical wooden rods with 12 cm height and 8 mm diameter were glued with 8 cm distance (TS) on the floodplain. The ratio of tree spacing to tree diameter was set TS/D = 80/8 = 10 in this experimental test, which is applicable in natural river. For large trees, normally 10 times of the grown tree diameter is recommended for tree cultivation.
The test reach was 2 m and it was selected 10 m distance from the entrance of the flume. For experimental test, the distance of trees from the edge of floodplain (d) was set 0, 10%, 20%, 30% and 40% of the flood plain width. Flow conditions in the experimental tests are described in . The flow was fully turbulent and subcritical. The bed of main channel is covered with 8 cm thickness of sediment particles. The d 50 size of sediment particles was 0.8 mm to provide the mobility in bed particles. The three-dimensional flow velocities were measured using acoustic Doppler velocity (ADV) meter with sampling rate of 50 Hz in 149 different nodal points. The flow velocity measurement in depth and in each cross-section of different cases is selected between 11th and 12th tree. shows the grid of data acquisition in cross-section of flow inside the compound channel and shows the cross-section of flow inside the assymetric compound channel.
Figure 3 (a) Grid of data acquisition in cross-section of flow inside the compound channel; (b) cross-section of assymetric compound open channel
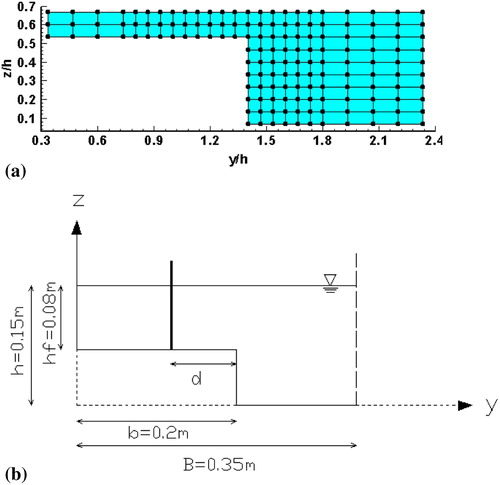
Table 1 Flow conditions in the experimental tests
Q is the flow rate, d is distance of trees on the floodplain from the main channel/floodplain boundary, TS is distance of trees from each other that its value is fixed and equal to 8 cm, t is duration of sampling at each point with ADV, h f is the flow depth on the floodplain, h is the total flow depth in the compound channel cross-section, Fr is Frode number and S b is the value of bed slope, y is lateral distance from the wall of floodplain, z is vertical distance from the bed of the flume, B is half width of compound channel and b is width of floodplain, respectively. Schematic descriptions of the above parameters are shown in .
3 Results and discussion
In this study, the distribution of streamwise velocity (velocity in flow direction), secondary current, turbulence intensity and turbulent kinetic energy were used as the parameters to identify and to recognize the best location of tree cultivation at the flood plain.
3.1 Distribution of streamwise velocity in cross-section of flow at different experiments
shows the contour lines of the streamwise velocity which is normalized by maximum streamwise velocity (u/u max) in different experimental tests.
From , it was found that at Run 1 of the experimental tests, which was performed in compound channel with no vegetation on the floodplain, the region with minimum value of streamwise velocity occurred at the wall of main channel but the region with high value of streamwise velocity occurred in the interface of main channel and flood plain. Also, the region with average value of streamwise velocity occurred on the floodplain ().
At Run 2, in which trees are cultivated just at the edge of floodplain, region with minimum value of streamwise velocity occurred in the back of the trees and far from the location of cultivating the tree and at the edge of the floodplain. The region with high value of streamwise velocity in main channel interacts with the region with minimum value of streamwise velocity in the location of cultivating the trees at the edge of floodplain. However, the region with high value of streamwise velocity in main channel is stronger than the region with minimum value of streamwise velocity in the main channel. Therefore the region with minimum value of streamwise velocity occurred far from the edge of the floodplain on the floodplain. The region with average value of streamwise velocity occurred at the edge of the floodplain (). In fact, at the location of cultivating the tree at the edge of the floodplain, there is severe disturbance of flow, but by getting far from this location, the region with low velocity is apparent. In natural rivers, trees which are cultivated near the edge of floodplain on the floodplain are not stable ().
At Run 3, region with low value of streamwise velocity occurred at the edge of floodplain and continued to the back of tree (). and clearly indicates that as the trees are cultivated far from the edge of the floodplain, the region with minimum value of streamwise velocity moves towards the wall of the floodplain. As a result, at Run 6, the trees are cultivated at a distance equal to 0.4 times the floodplain's width from the edge, therefore the region with minimum values of streamwise velocity moved between the location of cultivated trees and the wall of the floodplain ().
3.2 Analysis of secondary currents at different experiments
Yang et al. Citation(2007a) compared the secondary currents in rigid bed compound open channel in four conditions of no vegetation, vegetation on floodplain by grass, shrub and tree and found that for relative depths (D r = h f/h) of about 0.7, the secondary cells on the floodplain after vegetation were weak. In this study, relative depths (D r = h f/h) were 0.53, and the results of flow streamlines are presented for different experiments in . There are two vortex in main channel and floodplain in no vegetation test () which they are reported by some researches such as Shiono and Knight Citation(1991). By cultivating trees on the floodplain, the strength of vortices on the floodplain are decreased; especially at Run 6, in a region between tree and wall of floodplain, some minor secondary current cells are generated by the effect of tree and wall, in which these results are consistent with the result of Yang et al. (Citation2007a).
3.3 The location of minimum value of streamwise velocity in floodplain for different experiments
shows that after cultivating trees on the floodplain, the location of minimum value of streamwise velocity in floodplain moves towards the edge of floodplain and by approaching the location of cultivating the trees to the edge of floodplain, the location of minimum value of streamwise velocity in floodplain moves towards the edge of floodplain except at Run 2 (d = 0 cm). At Run 2, the region with high value of streamwise velocity in main channel interacts with the region of low value of streamwise velocity on the floodplain. The location of minimum value of streamwise velocity in floodplain runs away from the edge of floodplain. As a result of this section, the location of minimum value of streamwise velocity in floodplain at Run 3 (d = 2 cm) has the closest distance from the edge of floodplain in comparison with other experiments and this is a good result for protecting the edge of floodplain. In fact, cultivating trees at y = 10% of width of floodplain in these experiments is an optimum distance for decreasing the streamwise velocity at the edge of floodplain and for protecting this point.
3.4 The location of maximum value of streamwise velocity in floodplain for different experiments
The location of maximum value of streamwise velocity in floodplain for different experiments is shown in . The results indicated that the location of maximum streamwise velocity in floodplain moved towards the wall of floodplain after cultivating trees on the floodplain except in Run 6. At Run 6, the flow on the floodplain can be divided into two regions: region 1 is confined between tree and wall of floodplain in which, by the effect of these two obstructions, the region with minimum value of streamwise velocity occurred; region 2 is located between the edge of floodplain and tree. In this region, the streamwise velocity values are greater than those of region 1 and maximum streamwise velocity occurred in this region.
3.5 Turbulent intensities at the edge of floodplain near the bed
Turbulent intensities were computed at the edge of floodplain near the bed in streamwise direction (δ u), lateral direction (δ v) and vertical direction (δ w) for different experiments using the following equation:
3.6 Turbulent kinetic energy at the edge of floodplain near the bed
In this section, turbulence kinetic energy is determined for different experiments at the edge of floodplain and near the bed according to the following equation:
As shown in , it was found that streamwise turbulent kinetic energy has the highest value rather than lateral and vertical directions and its value is approximately equal to lateral direction. At Run 2, turbulent kinetic energy in streamwise direction and lateral direction has highest values in comparison with all other experiments.
3.7 Vertical distribution of turbulence intensity for streamwise, lateral and vertical directions at y/h = 1.33 in the floodplain and at y/h = 1.4 in the main channel
Vertical distribution of turbulence intensity for streamwise, lateral and vertical directions at y/h = 1.33 in the floodplain and at y/h = 1.4 in the main channel are shown in and . In these figures, grass, no vegetation, shrub and tree refer to the results of Yang's paper and Runs 1, 2, 3, 4, 5 and 6 refer to the results of the present study. As described by Yang et al. Citation(2007a), H r is defined for the floodplain and main channel, separately using following equations:
Figure 9 Vertical distribution of turbulence intensity at y/h = 1.33 and in the floodplain for (a) streamwise, (b) lateral and (c) vertical directions
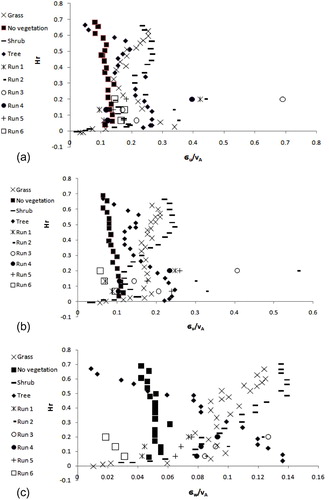
Figure 10 Vertical distribution of turbulence intensity at y/h = 1.4 in the main channel for (a) streamwise, (b) lateral and (c) vertical directions
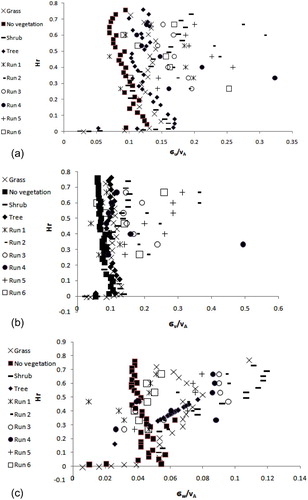
It can be seen that the streamwise and lateral intensities are approximately equal and vertical turbulence intensities have minimum values. These are consistent with the results of Yang et al. Citation(2007a). There are some differences between the experimental condition of this study and Yang et al. Citation(2007a), such as density of cultivating, kinds of vegetation, side slope of main channel, relative depths (D r = h f/h), which may affect the result.
4 Conclusions
The main purpose of this study is to find how far the trees must be cultivated from the edge of flood plain. To answer the above question, an experimental study was carried out to investigate the effect of cultivating trees at different distances from the main channel/floodplain boundary. Three-dimensional velocities were measured by acoustic Doppler velocity meter. The results indicated that cultivating trees just at the edge of floodplain does not affect decreasing streamwise velocity at the edge of floodplain. Furthermore, it was found that cultivation of tree at 10% of flood plain width produces minimum streamwise velocity at the edge of floodplain and hence is suitable for river bank protection. Therefore, for generating the region with minimum values of streamwise velocity at the edge of floodplain and for preventing instability of trees at the cultivating location, trees must be cultivated at a distance of 10% of floodplain width and it is suggested for practical application.
Additional information
Notes on contributors
Ali Reza Keshavarzi
Present address: School of Civil & Environmental Engineering, F.E.I.T., UTS, Broadway, NSW, 2007, Australia. Tel: +612 95142286, Fax: +61 2 9514 7920.Notes
References
- Bousmar , D. and Zech , Y. 1999 . Momentum transfer for practical flow computation in compound channels . Journal of Hydraulic Engineering, ASCE , 125 ( 7 ) : 696 – 706 .
- Christodoulou , G. C. 1992 . Apparent shear stress in smooth compound channels . Water Resources Management , 6 ( 3 ) : 235 – 247 .
- Fischer-Antze , T. 2001 . 3D numerical modelling of open-channel flow with submerged vegetation . Journal of Hydraulic Research , 39 ( 3 ) : 303 – 310 .
- Helmio , T. 2002 . Unsteady 1D flow model of compound channel with vegetated floodplains . Journal of Hydrology , 269 ( 1 ) : 89 – 99 .
- Huthoff , F. 2008 . Interacting divided channel method for compound channel flow . Journal of Hydraulic Engineering, ASCE , 134 ( 8 ) : 1158 – 1165 .
- Hua , W. 2007 . Computation of momentum transfer coefficient and conveyance capacity in compound channels . Journal of Hydrodynamics , 19 ( 2 ) : 225 – 229 .
- Joung , Y. and Choi , S. U. 2008 . Investigation of twin vortices near the interface in turbulent compound open-channel flows using DNS data . Journal of Hydraulic Engineering, ASCE , 134 ( 12 ) : 1744 – 1756 .
- Kang , H. and Choi , S. U. 2005 . 3D numerical simulation of compound open-channel flow with vegetated floodplains by Reynolds stress model . Journal of Civil Engineering, ASCE , 9 ( 1 ) : 7 – 11 .
- Knight , D. A. and Demetriou , J. D. 1983 . Floodplain and main channel flow interaction . Journal of Hydraulic Engineering, ASCE , 109 ( 8 ) : 1073 – 1092 .
- Knight , D. W. and Hamed , M. E. 1984 . Boundary shear stress in symmetrical compound channels . Journal of Hydraulic Engineering, ASCE , 110 ( 10 ) : 1412 – 1430 .
- Myers , W. R.C. 1978 . Momentum transfer in a compound channel . Journal of Hydraulic Research , 16 ( 2 ) : 139 – 150 .
- Myers , W. R.C. and Brennan , E. K. 1990 . Flow resistance in compound channels . Journal of Hydraulic Research , 28 ( 2 ) : 141 – 155 .
- Myers , W. R.C. and Elsawy , E. M. 1975 . Boundary shear in channel with floodplain . Journal of Hydraulics Division, ASCE , 101 ( 7 ) : 933 – 946 .
- Ozbek , T. 2004 . Comparison of methods for predicting discharge in straight compound channels using the apparent shear stress concept . Turkish Journal of Engineering Environmental Science , 28 : 101 – 109 .
- Rajaratnam , N. and Ahmadi , R. 1981 . Hydraulics of channels with flood-plains . Journal of Hydraulic Research , 19 ( 1 ) : 43 – 60 .
- Sellin , R. H.J. 1964 . A laboratory investigation into the interaction between the flow in the channel of a river and that over its floodplain . La Houille Blanche , 110 : 689 – 789 .
- Shimizu , Y. and Tsujimoto , T. 1994 . Numerical analysis of turbulent open channel flow over a vegetation layer using a k–e turbulence model . Journal of Hydroscience and Hydraulic Engineering , 11 ( 2 ) : 57 – 67 .
- Shiono , K. and Knight , D. W. 1991 . Turbulent open channel flow with variable depth across the channel . Journal of Fluid Mechanics Digital Archive , 222 : 617 – 646 .
- Tsujimoto , T. , Shimizu , T. and Okada , T. 1991 . Turbulent structure of flow over rigid vegetation-covered bed in open channels , Japan : Hydraulic Laboratory, Kanazawa University . KHL Progressive Report 1
- Tsujimoto , T. 1992 . Turbulent open-channel flow over bed covered by rigid vegetation . Journal of Hydroscience and Hydraulic Engineering , 10 ( 2 ) : 13 – 25 .
- Wilson , C. A.M.E. 2006 . 3D numerical modelling of a willow vegetated river/floodplain system . Journal of Hydrology , 327 ( 1–2 ) : 13 – 21 .
- Yang , K. , Cao , S. and Knight , D. W. 2007a . Flow patterns in compound channels with vegetated floodplains . Journal of Hydraulic Engineering, ASCE , 133 ( 2 ) : 148 – 159 .
- Yang , K. , Cao , S. and Liu , X. 2007b . Flow resistance and its prediction methods in compound channels . Acta Mechanica Sinica , 23 : 23 – 31 .
- Zarrati , A. R. , Jin , Y. C. and Karimpour , S. 2008 . Semianalytical model for shear stress distribution in simple and compound open channels . Journal of Hydraulic Engineering, ASCE , 134 ( 2 ) : 205 – 215 .
- Zheleznyakov , G. V. Interaction of channel and floodplain flows . Proceedings of the 14th international congress of IAHR . Paris. pp. 144 – 148 .