ABSTRACT
Needs for extending the principles of a sustainable urban drainage approach to the winter season with snow and pollutant accumulations have been so far unanswered. One of the first steps to remedy this situation is to make available a prototype snow management tool (PSMT) described herein. It is a simple empirical spreadsheet tool, as yet untested, which uses generally available input data to simulate accumulation of snow (as snow water equivalent) and pollutants in an urban catchment, intermittent snowmelt episodes, and snow management options including in situ melting, removal from the catchment, treatment of snowmelt by settling, and snow disposal in the receiving waters. The tool output comprises snow and the selected pollutant mass balances, and pollutant concentrations in snowmelt from individual snow deposits. Such data provide decision support for choosing the methods of disposal for individual snow deposits. The testing and refinement of the tool is planned.
1 Introduction
In cool temperate climate, with seasonal snow and freezing temperatures, the urban hydrological cycle is modified by formation of snow deposits in urban catchments, reduced infiltration into frozen soils, and by snow management practices. Snow deposits are formed in various shapes (Valeo and Ho Citation2004; Moghadas et al. Citation2016a) usually described as: (a) limited-extent snowpacks in parks and at green infrastructure sites, (b) snowbanks along roads, streets, and sidewalks, formed by snow clearance operations, (c) small piles at local (neighbourhood) storage sites for cleared snow (e.g. along the perimeter of parking lots, in residential courts and in roadside swales/ditches)(Reinosdotter and Viklander Citation2006), and (d) large piles at the central snow storage sites of various designs with respect to snow storage and management of snowmelt (Exall et al. Citation2011). Snow deposits represent storage of water in the form of snow and ice, which is subject to mechanical redistribution by ploughing or removal, melting by solar radiation or heat released by traffic and buildings, or chemical melting by salt. The melting of snow deposits is affected by their form and the released snowmelt runoff follows urban drainage pathways, but the runoff hydrograph may be further modified by reduced infiltration into frozen soils (Moghadas et al. Citation2016b), or blockage of sewer inlets by ice. In general, urban catchments release snowmelt at flow rates much lower than those associated with runoff from high-intensity rainfall events, but large volumes of snowmelt may contribute to water ponding and flooding in urban areas, particularly during rain-on-snow events occurring in late winter (Caraco and Claytor, Citation1997; Moghadas et al. Citation2018).
Besides differences in runoff formation, timing and duration, the rainwater runoff and snowmelt runoff significantly differ in their water quality (Galfi et al. Citation2016). These differences arise from different: (a) pollutant sources and their strengths, (b) durations of pollutant accumulation prior to their release, and (c) mechanisms of pollutant release into runoff. In the following, the above differences are illustrated by limited episodic evidence, because no systematic comparisons of rainwater runoff and snowmelt runoff quality, in the same urban catchment, were found in the literature.
Compared to other seasons, the mass of pollutants released into the urban environment during the winter season is disproportionally high (Valtanen, Sillanpää, and Setälä Citation2014; Chen et al. Citation2018), for a number of reasons: effective scavenging of atmospheric pollutants by snowflakes (Lei and Wania Citation2004; Vasić et al. Citation2012; Kurzyca and Frankowski Citation2019), less efficient operation of motor vehicles, increased heating of buildings (Engelhard et al. Citation2007), the use of studded tires (Lygren, Gjessing, and Berglind Citation1984), abrasion of road pavements by snow ploughing, applications of de-icing chemicals (Marsalek Citation2003; Salminen, Nysten, and Tuominen Citation2011) and anti-skid materials (Westerlund and Viklander Citation2011; Sillanpää and Koivusalo Citation2013; Nazarenko et al. Citation2016) in road and sidewalk maintenance, and urban litter (Dris et al. Citation2015). Thus, the major sources of pollutants in urban snow are emissions from traffic (Viklander Citation1999; Kuoppamäki et al. Citation2014), deicing chemicals and traction materials (and their impurities) used in winter road maintenance, wet and dry atmospheric deposition (Chen et al. Citation2018; Müller et al. Citation2020), and urban litter, in the case plastic waste (Bergmann et al. Citation2019).
There is a consensus in the literature that the quality of urban stormwater from impervious surfaces generally depends on the antecedent dry period (ADP) of the rainfall event generating runoff, with longer ADPs allowing greater accumulations and wash-off of pollutants, and therefore, the greater pollution loads conveyed by runoff (Soonthornnonda and Christensen Citation2008; Egodawatta, Thomas, and Goonetilleke Citation2009; Charters, Cochrane, and O’Sullivan Citation2020). ADP values in runoff studies reported in the literature typically range from 1–14 days, but for urban snow, the corresponding periods of the snow deposit residence times can be much longer, up to 120 days (Vijayan et al. Citation2019). Thus, the magnitude of pollutant accumulations in urban snow deposits depends on snow age (Moghadas et al. Citation2015), among other factors. During intermittent minor melts, dissolved pollutants may be preferentially eluted from snow deposits, but solids and hydrophobic pollutants are mostly retained and contribute to high concentrations of traffic associated pollutants (metals and polycyclic aromatic hydrocarbons, PAHs) in old snow (Reinosdotter, Viklander, and Malmqvist Citation2006; Meyer et al. Citation2009; Moghadas et al. Citation2015).
Releases of pollutants from snow deposits can be attributed to in-situ snowmelt, particularly in the case of dissolved pollutants or fine solids (Vijayan et al. Citation2019). Additional pollutants leave snow deposits with removed snow, which is transported to, and disposed of at, designated storage facilities, within or outside of the urban catchment (Exall et al. Citation2011), or in the local receiving waters, where the disposal in open waters is permitted. For example, Magnusson et al. (Citation2016) reported that the Stockholm City alone had permission to annually dump 800,000 m3 of snow collected from streets into the open waters around the city.
In spite of obvious differences between stormwater and snowmelt characteristics in regions with seasonal snow, drainage design and stormwater management are generally based on the rainfall regime and pollutant releases during the warm seasons of the year, with minimal considerations given to the winter/early spring period. While this may be acceptable for dealing with severe rain storms and the sizing of drainage flow conveyance elements, it may be inadequate for: (a) designing runoff and snowmelt storage capacities (Caraco and Claytor, Citation1997), (b) mitigation of sudden pollutant releases from old snow deposits (Moghadas et al. Citation2015), or (c) addressing the dumping of cleared urban snow into the receiving waters.
Whereas the current urban snow management is driven by considerations of traffic safety and the quality of urban life, the impacts of urban snow and snowmelt pollution on the receiving waters should not be underestimated. Therefore, it is suggested in this paper that management of urban snow should be expanded for critical environmental considerations and thereby made consistent with, and supportive of, the goals of sustainable urban drainage pursued during warmer parts of the year without snow. In operational terms, such a proposed management approach would comprise three fundamental tasks: (a) developing an inventory system of snow/water/pollutant loads in snow deposits in urban catchments, which can be updated with a certain time step, (b) assessing inputs or removals of snow/water/pollutants to, or from, urban snow deposits, and (c) assessing snow management interventions ranging from a direct disposal of fresh, relatively unpolluted snow in the receiving waters to a winter-long management of urban snow removal and disposal, including special handling of polluted snow deposits and optional snowmelt treatment. The overall operation of this process should be supported by a computerized snow management tool (SMT). The objective of this paper is to present a prototype of such a tool (referred to as PSMT), which is not definite and has not been tested yet against actual field data, but even in its current form, it should be helpful for initiating discussions of the need for SMT, and its architecture, features, and applications.
2 Methods
2.1 Overview of the PSMT
At the current low technology readiness level, the snow management tool (PSMT) is envisaged as a relatively simple practical tool for conducting calculations to support informed decisions of professional practitioners concerning the implementation of snow management strategies in urban catchments or on sites, with the objective of controlling the environmental impacts of such strategies on the receiving waters. These impacts arise from discharges of polluted snowmelt or dumping of used snow into the receiving waters. As a parsimonious tool, PSMT should require minimal data, which may be available from local sources, e.g. precipitation, air temperatures, land use, traffic intensity, and snowmelt quality, or should contain ‘default’ data, particularly with respect to chemistry inputs, in the tool documentation. At the same time, the tool users should be encouraged to collect and use their own input data, which would make the modelling results more robust and acceptable to end–users. PSMT should consider the following wintry catchment processes: Precipitation; snow accumulation, storage, age-related changes in water content, chemistry, and melting; snowmelt treatment by settling in stormwater ponds or tanks; and, conceptual snow disposal management measures.
Three snow disposal management measures were considered at the current level of the PSMT technology development:
Natural in-situ snow melting that represents the least-cost option, which may be applied at sites with low snow pollution (e.g. at the sites not exposed to traffic pollution). PSMT would help identify sites, where this option is acceptable.
As pressure on municipal snow clearance operations subsides after a significant snowfall, there may be an opportunity for prioritizing snow removal and transport to on-land disposal sites (Reinosdotter and Viklander Citation2006), ensuring that the more polluted snow (i.e. the snow exposed to stronger sources of pollution over longer times) is processed first, rather than allowed to melt in-situ and release pollutants through the storm drainage system into the receiving waters.
The feasibility of ‘fresh’ snow removal and dumping into nearby receiving waters, where permitted. For this low-cost option, the tool would provide the snow residence time, which is indicative of snow quality (Vijayan et al. Citation2021), and help make a decision at what snow age, measured in days, this option becomes unacceptable, because of the pollution burden in snow.
2.2 PSMT description
The accumulation of snow pollutants, snowmelt runoff and subsequent transport of pollutants to the receiving waters, as well as treatment options, are simulated, with some limitations, in three steps representing the PSMT modules: (a) Accumulation of water and pollutants in snow deposits, (b) Transport of water and pollutants from snow deposits and the catchment, and (c) Treatment of snowmelt by settling in stormwater ponds or tanks. Results of such computations include snow/water mass balances, and concentrations and mass loads of water quality constituents for individual snow deposits as well as the whole catchment. The mass balance values (SWE, snow water equivalent, and pollutant residual mass in snow) at the end of the current simulation period can be used as the initial values for the next period. Recognizing that the inputs or process parameters may change independently of the temperature, the user can account for such changes by choosing shorter simulation periods, during which the steady state assumptions are valid. Further details follow.
2.2.1 Accumulation module (AM) in PSMT
In general, the simulation periods of snow conditions in the study area can be divided into two categories: (a) periods with air temperatures below the freezing point of water, when SWE of snow deposits may increase, if there is a snowfall; or, remain unchanged, if there is no snowfall; and, (b) periods of transport of water from snow deposits or the catchment, when the temperatures are above the freezing point. The former periods are referred to as the periods of accumulation (i.e. without snowmelt), the latter ones are referred to as the periods of transport. Some deviations from these assumptions may be caused by salt applications (reducing the snow eutectic temperature), as elaborated later. Based on the length of the simulation period and the daily precipitation as snow, the total mass of snow is calculated and described by SWE values. The user then estimates the spatial distribution of snow over the catchment among the four classes of snow deposits: (a) undisturbed snow, (b) snow cleared (ploughed) to sides of roads and streets, (c) in-catchment snow storage sites (up to two sites can be specified), and finally (d) snow transported out of the catchment.
For snow deposits within the catchment, three basic types of pollutant sources are included in the PSMT tool: (a) vehicular traffic in the immediate vicinity of snow deposits, (b) applications of grit and deicing salts, and (c) atmospheric deposition. It is assumed that pollutant accumulation rates are constant during the simulation period, and the accumulated pollutant burdens increase linearly with time, as reported by Moghadas et al. (Citation2015). For each type of snow deposits, specific input data are defined by the user, including the initial conditions (i.e. the initial mass of snow as SWE and snow pollutant masses), the area (footprint), and the strength of pollutant sources, e.g. inputs from traffic of various intensities (described by the Annual Average Daily Traffic, AADT), application rates of salt and grit, or the strength of other pollutant sources (atmospheric deposition), during the simulation period.
Special considerations were applied to grit and benzo(a)pyrene. In the former case, grit applied in road maintenance represents fine-to-intermediate gravel, which is not removed hydraulically by snowmelt, but remains on roads and road shoulders more or less during the entire winter period. Excessive gravel is collected after the winter and processed. Therefore, gravel applications as a source of TSS (total suspended solids) were omitted in the SMT tool. The second constituent of special interest, benzo(a)pyrene (a carcinogen), was simulated as an assumed fraction of the sum of the US EPA 16 PAHs, by using a potency factor, which can be obtained as a default value in the PSMT, or defined by the user. A similar approach could be applied in the case of gravel, some of which may be ground into smaller particles by vehicle tires.
The processes simulated by the PSMT Accumulation Module are summarized in the flowchart shown in and input data are summarized in . Further comments on and guidance for selecting input data are presented in the Supplementary Information.
Table 1. Input data required for running the PSMT accumulation module
2.2.2 Transport module (TM) in PSMT
Simulations of snowmelt-induced runoff and the resulting pollutant transport are carried out assuming that the ambient air temperature is above the freezing point and no refreezing of snow deposits occurs during this period. Snowmelt can be calculated by the degree-day method (USACE Citation1998) as a linear function of the difference between the average air temperature and the temperature, at which snow starts to melt. Such computations are based on a temperature-index type of equation, which is used frequently in calculations of urban snowmelt, e.g. in the SWMM model (Rossman Citation2015; Moghadas et al. Citation2016a). The method was modified here by including a snowmelt coefficient reflecting various characteristics of different types of urban snow deposits, including applications of salt (Valeo and Ho Citation2004; Moghadas et al. Citation2016a), and solar radiation and shading. Limited guidance is provided for choosing snowmelt coefficient values in PSMT. Hydrologic abstractions during runoff (e.g. infiltration and surface wetting and depression storage) can be accounted for by a user-defined dimensionless runoff coefficient reflecting such abstractions during the simulated event. Based on the above inputs, the potential snowmelt (i.e. the maximum possible snowmelt) and an actual accumulated snowmelt runoff volume are estimated. Rain on snow can be entered as an additional water volume, which is added to the runoff volume occurring during the snowmelt period. However, such a procedure neglects the rainwater retained in certain snow deposits (e.g. undisturbed snow) and will be reexamined in future tool versions. Furthermore, heat conveyed by the rainwater landing on snow deposits was assumed to be negligible and hence did not affect (increase) snowmelt rates.
In simulations of pollutant fluxes, it was assumed in PSMT that the concentrations of pollutants in snowmelt runoff were constant during the simulation period. However, not all pollutants retained in the respective snow deposits are transported with runoff; some will remain in the storage areas. The fractions of TSS and other pollutants retained by the snowpack during a single snowmelt period (event) can be defined by the user, and further guidance is provided in the form of default retention values suggested in the PSMT tool. When selecting such retention values, the user can distinguish among three classes of urban pollutants, depending on their hydrophilicity or hydrophobicity, and those classes were operationally defined as follows: (1) primarily dissolved chemicals (e.g. chloride); (2) chemicals occurring in runoff/snowmelt in significant fractions in both dissolved and particulate phases (e.g. some nutrient or trace metal species), and (3) hydrophobic chemicals (e.g. most PAHs, or some trace metal species). Based on the runoff volume and fraction of TSS and other constituents leaving the snow deposit, both the pollutant mass in snowmelt leaving the snow deposit, and the residual pollutant mass remaining in the deposit, are calculated. The load fraction transported by the event snowmelt is used to calculate the pollutant concentration, averaged over the event duration. Hence, such event concentrations have a similar physical meaning as the event mean concentrations (EMC) used in analysis of stormwater data.
The processes simulated by the Transport Module of PSMT are shown in , and the associated input data are listed in .
Figure 2. Snowmelt and pollutant transport from snow deposits simulated by the transport module of PSMT
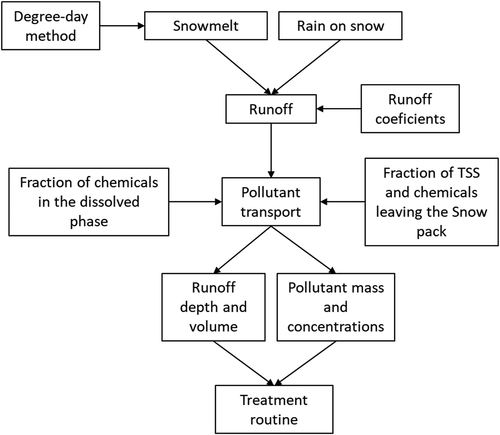
Table 2. Input data for computing snowmelt and pollutant transport from snow deposits by the PSMT transport module
2.2.3 Treatment module in PSMT
There is only one treatment process included in the Treatment Module of PSMT, stormwater/snowmelt settling. Such treatment was simulated in PSMT by assuming ideal particle settling modified (perturbed) by consideration of the hydraulic efficiency of a settling pond or tank under dynamic conditions characterized by turbulence and short-circuiting flows (Pettersson Citation1999). In this approach, the unhindered settling of discrete non-cohesive solid particles released on the water surface depends on two variables: the particle fall velocity and the settling tank overflow rate (= Q/A, where Q is the discharge through the tank and A is the surface area of the tank or pond). This equation was later expanded by including the settling of particles initially suspended below the water surface (Metcalf & Eddy Citation2014) and modified for dynamic settling conditions described by factor n (Pettersson Citation1999), which can be related to the hydraulic efficiency (λ) of the settling tank by an expression λ = 1–1/n. For typical layouts of stormwater settling basins, Persson, Somes, and Wong (Citation1999) and Persson (Citation2000) developed the hydraulic efficiencies of shallow rectangular settling basins. For ideal settling conditions, λ → 1, and for highly disturbed settling λ → 0. Furthermore, Persson, Somes, and Wong (Citation1999) developed λ values for a set of 12 rectangular basins, and one L-shaped basin, of various geometries, some containing baffles, or islands, or partly submerged partition walls, and various inlet/outlet configurations. Examples of settling tank layouts and their hydraulic efficiencies are shown in ; for detailed information see Persson (Citation2000). The above-described empirical approach contains simplifications, because the actual settling processes are rather complex and dynamic (Metcalf & Eddy Citation2014), and not tractable by a simple spreadsheet tool. However, the simplified empirical approach adopted here is consistent with the operational nature of PSMT and the user can get some appreciation of the sensitivity of settling results to the assumed hydraulic efficiencies by running the tool for several values of λ.
Figure 3. Schematic presentation of hydraulic efficiencies (λ) of various settling basin layouts and inlet/outlet configurations (after Persson, Somes, and Wong Citation1999)
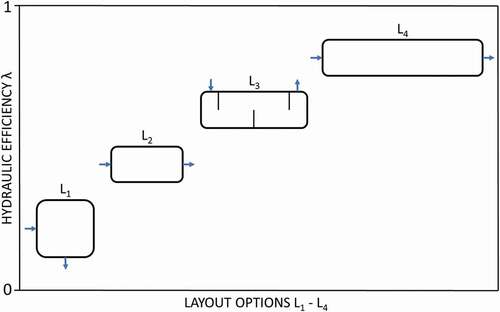
In specific site applications, treatment can be defined for each type of snow deposit, as well as for the aggregate of all snow deposits in the catchment. In treatment simulations, it is assumed that snowmelt runoff is collected and transported to the treatment facility (i.e. a pond or a tank settler), and subject to settling. Besides solids, chemicals associated with particulates are also removed by settling, but dissolved chemicals pass through unimpeded. As stated earlier, the partitioning of chemicals between the dissolved and particulate phases is defined by the user in three classes as: predominantly dissolved or hydrophobic, or a mix of the first two.
In order to estimate the removals of particles and adsorbed chemicals, four types of inputs are needed and detailed in : (a) runoff/snowmelt flow rate (computed by the tool), (b) temperature of runoff/snowmelt, (c) the surface area of the settling facility (user specified), and (d) user-specified particle characteristics, including particle density and a particle size distribution (PSD), which can be specified in PSMT by up to five size fractions: ≤ 6, 25, 62.5, 125 and 250 µm. Particle removals by settling can be computed for individual size fractions, or for a combination of all sizes. Finally, the user can also specify the average meltwater temperature, which affects both the density and dynamic viscosity of the runoff/snowmelt, and thereby the settling velocity of particles. Such effects are calculated for individual particle size fractions as well as for the combination of all particle sizes. Simulations carried out by the PSMT Treatment Module are summarized in .
Table 3. Input data required for running the PSMT treatment module
2.2.4 PSMT results summary
Important results of PSMT simulations are summarized on a single page and include the following:
Water balance for the analyzed study area (catchment), which is calculated as Mwes = Mwbs + Mwp – Mwex, where Mwbs and Mwes is water mass at the beginning and end of simulation, respectively; Mwp is the mass of precipitation occurring during the simulation period, and Mwex is the mass of water exported from the catchment surface as runoff, or retained in hydrological abstractions.
Water quality constituent mass balances are calculated as Mqces = Mqcbs + Mqcins – Mqcex, where Mqcbs and Mqces are the quality constituent masses in catchment snow deposits at the beginning and end of simulation, respectively, Mqcins is the quality constituent mass added to catchment snow during the simulation period, and Mqcex is the mass of constituent exported from the catchment with runoff/snowmelt during the simulation period. Besides masses (loads, burdens), the average constituent concentration in runoff/snowmelt during the simulation period is calculated as Mqcex/Mwex, and compared to relevant environmental guideline values provided as default values in the PSMT (Jacobs et al. Citation2009; Gothenburg Municipality Citation2013). The pollutant concentrations are calculated for individual snow deposits, as well as for an aggregate of all snow deposits in the catchment.
3 Discussion
The PSMT is a unique tool by its capability to combine three aspects of urban snow management: (a) snow accumulation and depletion by attrition or snowmelt, and collection and removal to central storage or a disposal site; (b) characterization of water quality constituents in snow deposits and constituent releases, and (c) management of urban snow by mechanical removal, or in-situ melting and treatment. Such a scope is innovative and unique, as no similar attempts have been found in the literature. At the same time, as described here, PSMT is a highly empirical operational spreadsheet tool allowing the user to control tool operation by input of locally collected field data tempered by practical experience. The proposal of this tool by the authors was motivated by the need to bring the management of winter precipitation and snowmelt to a higher level consistent with sustainable urban drainage practiced during warm seasons of the year, and also by working with a specific client interested in snow management at an urban industrial site, with emphasis on small scales and simple approaches with limited data.
With respect to modelling snowmelt, the tool conceptually reflects the current practice in modelling urban snowmelt, summarized by Moghadas et al. (Citation2016a) and Dobrea, Gaitanarua, and Gogua (Citation2017), including the consideration of various types of snow deposits in urban areas (Valeo and Ho Citation2004) and application of the temperature index method for computing snowmelt (USACE Citation1998), which can be supported by the readily available data (Moghadas et al. Citation2016a). PSMT divides the winter period into two subsets of episodes, primarily determined according to the air temperature, either below or above the melting temperature of urban snow. The former subset represents the periods of snow accumulation, the latter one represents the periods of snowmelt. Minor deviations from these characteristics are possible by adjusting the temperature at the onset of melting, which could reflect the use of road salts, or other properties of urban snow (e.g. reduced albedo). As presented here, the transfer of data between simulation periods is done manually, which draws the attention of the user to the interim simulation results and possible needs for correction of input data.
Besides snowmelt, other forms of depletion (losses) of snow (e.g. by ablation, sublimation, or wind erosion) can be accounted for by the user by adjusting an empirical parameter. The PSMT tool allows to process rain-on-snow events in a simple way by diverting the rainwater to runoff, without modelling possible storage of rainwater in some of the snow deposits. This issue will be further investigated in future editions of the tool. Thus, in terms of water quantity, there are no immediate major concerns about the structure of the PSMT. It can be envisaged that in future editions of the PSMT the transfer of data between simulation periods will be done directly by the tool, the computational time step will be shorter than the current 24 h to reflect the dynamics of catchment hydrological processes, and the routing of snowmelt in streets, gutters and storm sewers would be done by one of the standard urban drainage modelling packages.
In an earlier study, Moghadas et al. (Citation2018) described their experience with modelling urban snowmelt with the SWMM model of US EPA, which is also using the temperature index equation for snowmelt computations. Their conclusion was that while it was feasible to simulate urban snowmelt runoff flows fairly well, but simulations of snowmelt quality were not feasible, primarily because of principal differences between the pollutant build up and wash-off in warm weather (without snow), as implemented by SWMM, and the corresponding processes in winter conditions. For that reason, in the PSMT, snowmelt quality was managed differently by using an empirical approach to accumulation of pollutants in urban snow deposits of various shapes and exposures to urban pollution sources, and pollutant releases, largely affected by hydrophobicity or hydrophilicity of various chemicals and materials. Proper handling of these issues requires some inherent knowledge of behaviour of various pollutants in urban areas, and will require further development in the future. One example of a water quality process occurring in snowpacks (but not in stormwater pollution buildup and washoff) is the preferential elution of dissolved chemicals from urban snow (Meyer et al. Citation2009; Kępski et al. Citation2016), which is considered in the SMT by specifying pollutant hydrophobicity. The development of process-based algorithms for computations of snowmelt quality in urban areas is rather unlikely in the near future (5–10 years) and the reliance on empirical or semi-empirical approaches, whose example is typified by the approach implemented in PSMT, is likely to continue. Simulation of snowmelt quality could be improved by using field data to quasi-calibrate the empirical approaches built into PSMT.
Among the snow management measures, the simplest one to simulate is the collection and removal of snow from the catchment and disposal at a specially designed central snow storage facility (Exall et al. Citation2011), serving for snow melting and snowmelt treatment in a pond. Depending on volumes of snow handled, this may not be an inexpensive and environmentally sustainable option, in view of the transportation costs and environmental impacts not only of used snow, but also of truck traffic generated by hauling snow. Where permitted, transportation costs may be avoided by disposing fresh urban snow directly into nearby receiving waters, or by practicing such a disposal after pretreatment of snowmelt by settling. Field data on accumulation of pollutants in snow cleared from a port facility in Stockholm indicate (Vijayan et al. Citation2021), that direct disposal of ‘fresh’ snow may be acceptable for relatively short periods of snow exposure (< 48 h) on the catchment surface, and would also depend on local sources of pollution, with high traffic rates and/or atmospheric deposition possibly imposing limits on this option. Snowmelt pretreatment by settling, prior to discharge to the receiving waters, would be effective for solids and hydrophobic chemicals (e.g. some traffic trace metals and PAHs). Finally, some combination of the studied snow management measures, including direct disposal of fresh snow (up to 2 days old), on-site melting and treatment, and as a last measure, transport of used snow to remote centralized sites, appears feasible.
4 Conclusions
In an effort to protect urban aquatic environments in regions with seasonal snow, a prototype of a practical computational tool (PSMT) has been proposed for managing snow and snowmelt pollution in urban areas. Using generally available input data, the tool simulates snow and pollutant accumulation in snow deposits of various shapes and exposures to pollution, the melting of snow and releases of pollutants, treatment of snowmelt by settling, and thereby provides guidance for sustainable urban snow management. Pollutant accumulations in snow and running mass balances provide support for decisions concerning management of snow removal and disposal options, including dumping of fresh snow in the receiving waters, in-situ melting, pre-treatment and discharge of snowmelt into receiving waters, or transporting snow away to central storage sites. The current prototype should be further tested in the field and refined.
Acknowledgements
This project was conducted within the research cluster Stormwater&Sewers. The financial support for this work was provided by the Swedish Research Council Formas, Ports of Stockholm and NCC through the project Sustainable urban snow handling - assessing and managing environmental risks (Grant no 2015-00120) and DRIZZLE Centre for Stormwater Management, funded by Vinnova – Sweden’s Innovation Agency (Grant no. 2016–05176). The authors are grateful to the project partners and the reference group on the Sustainable urban snow handling project, with representatives from Ports of Stockholm, NCC, County Administrative Board of Stockholm, City of Stockholm Environmental Department, and the Road Departments at Luleå and Örnsköldsvik municipalities, for fruitful discussions during the development of the PSMT.
Disclosure statement
No potential conflict of interest was reported by the author(s).
Additional information
Funding
References
- Bergmann, M., S. Mützel, S. Primpke, M.B. Tekman, J. Trachsel, and G. Gerdts. 2019. “White and Wonderful? Microplastics Prevail in Snow from the Alps to the Arctic.” Science Advances 5 (8): eaax1157 14, 1–10. doi:https://doi.org/10.1126/sciadv.aax1157.
- Caraco, D., and R. Claytor. 1997. Stormwater BMP Design Supplement for Cold Climates. Ellicott City, MD: Center for Watershed Protection.
- Charters, F.J., T.A. Cochrane, and A.D. O’Sullivan. 2020. “Predicting Event-Based Sediment and Heavy Metal Loads in Untreated Urban Runoff from Impermeable Surfaces.” Water 12 (4): 969. doi:https://doi.org/10.3390/w12040969.
- Chen, L., X. Zhi, Z. Shen, Y. Dai, and G. Aini. 2018. “Comparison between Snowmelt-runoff and Rainfall-runoff Nonpoint Source Pollution in a Typical Urban Catchment in Beijing, China.” Environmental Science and Pollution Research 25 (3): 2377–2388. doi:https://doi.org/10.1007/s11356-017-0576-z.
- Dobrea, R.-G., D.S. Gaitanarua, and C.R. Gogua. 2017. “Snowmelt Modelling Aspects in Urban Areas.” Procedia Engineering 209: 127–134. doi:https://doi.org/10.1016/j.proeng.2017.11.138.
- Dris, R., J. Gasperi, V. Rocher, and B. Tassin 2015. “Microplastic Contamination in an Urban Area.” SETAC Europe 25th annual meeting, Barcelona, Spain, May.
- Egodawatta, P., E. Thomas, and A. Goonetilleke. 2009. “Understanding the Physical Processes of pollutant Build-up and Wash-off on Roof Surfaces.” Science of Total Environment 407 (6): 1834–1841. doi:https://doi.org/10.1016/j.scitotenv.2008.12.027.
- Engelhard, C., S. De Toffol, I. Lek, W. Rauch, and R. Dallinger. 2007. “Environmental Impacts of Urban Snow Management — The Alpine Case Study of Innsbruck.” Science of the Total Environment 382 (2–3): 286–294. doi:https://doi.org/10.1016/j.scitotenv.2007.04.008.
- Exall, K., J. Marsalek, Q. Rochfort, and S. Kydd. 2011. “Chloride Transport and Related Processes at a Municipal Snow Storage and Disposal Site.” Water Quality Research Journal 46 (2): 148–156. doi:https://doi.org/10.2166/wqrjc.2011.023.
- Ferreira, M., and M.K. Stenstrom. 2013. “The Importance of Particle Characterization in Stormwater Runoff.” Water Environment Research 85 (9): 833–842. doi:https://doi.org/10.2175/106143013X13736496909103.
- Galfi, H., H. Österlund, J. Marsalek, and M. Viklander. 2016. “Indicator Bacteria and Associated Water Quality Constituents in Stormwater and Snowmelt from Four Urban Catchments.” Journal of Hydrology 539: 125–140. doi:https://doi.org/10.1016/j.jhydrol.2016.05.006.
- Gothenburg Municipality. 2013. “The Environment Department Guidelines and Concentration Limits for Discharges of Polluted Water into the Receiving Water and Stormwater (Miljöförvaltningens Riktlinjer Och Riktvärden För Utsläpp Av Förorenat Vatten till Recipient Och Dagvatten, in Swedish).” Gothenberg. Accessed 16 May 2020. https://goteborg.se/wps/wcm/connect/fee9bd22-ed19-43ed-907c-14fc36d3da16/N800_R_2013_10.pdf?MOD=AJPERES
- Jacobs, A., J. Fagerberg, M. Prima, R. Öjermark, S. Thörenlöf, H. Alm, and T. Larm, 2009. “Förslag till riktvärden för dagvattenutsläppn (In Swedish); Suggestion of Guideline Values for Stormwater Discharges.” Riktvärdesgruppen Regionala dagvattennätverket i Stockholms län. Stockholm, Sweden.
- Kępski, D., M. Błas, M. Sobik, Z. Polkowska, and N. Grudzinska. 2016. “Progressing Pollutant Elution from Snowpack and Evolution of Its Physicochemical Properties during Melting Period—a Case Study from the Sudetes, Poland.” Water, Air, & Soil Pollution 227 (4): 112. doi:https://doi.org/10.1007/s11270-016-2797-z.
- Kuoppamäki, K., H. Setälä, A-L. Rantalainen, and D.J. Kotze. 2014. “Urban Snow Indicates Pollution Originating from Road Traffic.” . Environmental Pollution 195: 56–63. doi:https://doi.org/10.1016/j.envpol.2014.08.019.
- Kurzyca, I., and M. Frankowski. 2019. “Scavenging of Nitrogen from the Atmosphere by Atmospheric (Rain and Snow) and Occult (Dew and Frost) Precipitation: Comparison of Urban and Nonurban Deposition Profiles.” JGR. Biogeosciences 7: 2288–2304.
- Lei, Y., and F. Wania. 2004. “Is Rain or Snow a More Efficient Scavenger of Organic Chemicals?” Atmospheric Environment 38 (22): 3557–3571. doi:https://doi.org/10.1016/j.atmosenv.2004.03.039.
- Lygren, E., E. Gjessing, and L. Berglind. 1984. “Pollution Transport from a Highway.” Science of the Total Environment 33 (1–4): 147–159. doi:https://doi.org/10.1016/0048-9697(84)90389-9.
- Magnusson, K., K. Eliasson, A. Fråne, K. Haikonen, J. Hultén, M. Olshammar, J. Stadmark, and A. Voisin. 2016. Swedish Sources and Pathways for Microplastics to the Marine Environment. Stockholm, Sweden: IVL Svenska Miljöinstitutet.
- Marsalek, J. 2003. “Road Salts in Urban Stormwater: An Emerging Issue in Stormwater Management in Cold Climates.” Water Science and Technology 48 (9): 61–70. doi:https://doi.org/10.2166/wst.2003.0493.
- Metcalf & Eddy. 2014. Wastewater Engineering: Treatment and Resource Recovery (Fifth Edition). New York, NY: McGraw Hill.
- Meyer, T., Y.D. Lei, I. Muradi, and F. Wania. 2009. “Organic Contaminant Release from Melting Snow. 2. Influence of Snow Pack and Melt Characteristics. Environ.” Environmental Science & Technology 43 (3): 663–668. doi:https://doi.org/10.1021/es8020233.
- Moghadas, S., A-M. Gustafsson, P. Viklander, J. Marsalek, and M. Viklander. 2016b. “Laboratory Study of Infiltration into Two Frozen Engineered (Sandy) Soils Recommended for Bioretention.” Hydrological Processes 30 (8): 1251–1264. doi:https://doi.org/10.1002/hyp.10711.
- Moghadas, S., A-M. Gustafsson, T.M. Muthanna, J. Marsalek, and M. Viklander. 2016a. “Review of Models and Procedures for Modelling Urban Snowmelt.” Urban Water Journal 13 (4): 396–411. doi:https://doi.org/10.1080/1573062X.2014.993996.
- Moghadas, S., G. Leonhardt, J. Marsalek, and M. Viklander. 2018. “Modeling Urban Runoff from Rain-on-Snow Events with the U.S. EPA SWMM Model for Current and Future Climate Scenarios.” . Journal of Cold Regions Engineering 32 (1): 04017021. doi:https://doi.org/10.1061/(ASCE)CR.1943-5495.0000147.
- Moghadas, S., K. H. Paus, T. M. Muthanna, I. Herrmann, J. Marsalek, and M. Viklander. 2015. “Accumulation of Traffic-related Trace Metals in Urban Winter-long Roadside Snowbanks.” Water, Air, & Soil Pollution 226 (12): 404. doi:https://doi.org/10.1007/s11270-015-2660-7.
- Müller, A., H. Österlund, J. Marsalek, and M. Viklander. 2020. “The Pollution Conveyed by Urban Runoff: A Review of Sources.” Science of the Total Environment 709: 13625. doi:https://doi.org/10.1016/j.scitotenv.2019.136125.
- Nazarenko, Y., U. Kurien, O. Nepotchatykh, R.B. Rangel-Alvaradoc, and P.A. Ariya. 2016. “Role of Snow and Cold Environment in the Fate and Effects of Nanoparticles and Select Organic Pollutants from Gasoline Engine Exhaust”. Environmental Science. Processes & Impacts, no. 2. doi:https://doi.org/10.1039/D0EM00398K.
- Persson, J. 2000. “The Hydraulic Performance of Ponds of Various Layouts.” Urban Water 2 (3): 243–250. doi:https://doi.org/10.1016/S1462-0758(00)00059-5.
- Persson, J., N. L. G. Somes, and T. H.F. Wong. 1999. “Hydraulics Efficiency of Constructed Wetlands and Ponds.” Water Science and Technology 40 (3): 291–300. doi:https://doi.org/10.2166/wst.1999.0174.
- Pettersson, T.J.R. 1999. “Stormwater Ponds for Pollution Reduction.” Ph.D. thesis no. 14, Department of Sanitary Engineering, Chalmers University of Technology, Gothenburg.
- Reinosdotter, K., and M. Viklander. 2006. “Handling of Urban Snow with Regard to Snow Quality.” Journal of Environmental Engineering 132 (2): 271–278. doi:https://doi.org/10.1061/(ASCE)0733-9372(2006)132:2(271).
- Reinosdotter, K., M. Viklander, and P.-A. Malmqvist. 2006. “Polycyclic Aromatic Hydrocarbons and Metals in Snow along a Highway.” Water Science and Technology 54 (6–7): 195–203. doi:https://doi.org/10.2166/wst.2006.600.
- Rossman, L. 2015. Storm Water Management Model Reference Manual, Volume I – Hydrology. Cincinnati, Ohio: U.S. Environmental Protection Agency.
- Salminen, J.M., T.H. Nysten, and S.M. Tuominen. 2011. “Review of Approaches to Reducing Adverse Impacts of Road Deicing on Groundwater in Finland.” Water Quality Research Journal 46 (2): 166–173. doi:https://doi.org/10.2166/wqrjc.2011.002.
- Sillanpää, N., and H. Koivusalo. 2013. “Catchment-scale Evaluation of Pollution Potential of Urban Snow at Two Residential Catchments in Southern Finland.” . Water Science and Technology 68 (10): 2164–2170. doi:https://doi.org/10.2166/wst.2013.466.
- Soonthornnonda, P., and E.R. Christensen. 2008. “A Load Model Based on Antecedent Dry Periods for Pollutants in Stormwater.” Water Environment Research 80 (2): 162–170. doi:https://doi.org/10.2175/106143007X220888.
- USACE. 1998. Engineering and Design – Runoff from Snowmelt. US Army, The Corps of Engineers: Washington, DC, USA.
- Valeo, C., and C.L. Ho. 2004. “Modelling Urban Snowmelt Runoff.” Journal of Hydrology 299 (3–4): 237–251. doi:https://doi.org/10.1016/S0022-1694(04)00368-3.
- Valtanen, M., N. Sillanpää, and H. Setälä. 2014. “Effects of Land Use Intensity on Stormwater Runoff and Its Temporal Occurrence in Cold Climates.” Hydrological Processes 28 (4): 2639–2650. doi:https://doi.org/10.1002/hyp.9819.
- Vasić, M.V., A. Mihailovic, U. Kozmidis-Luburic, T. Nemes, J Ninkov, T. Zeremski-Škorić, and B. Antić. 2012. “Metal Contamination of Short-term Snow Cover near Urban Crossroads: Correlation Analysis of Metal Content and Fine Particles Distribution.” Chemosphere 86 (6): 585–592. doi:https://doi.org/10.1016/j.chemosphere.2011.10.023.
- Vijayan, A., H. Österlund, J. Marsalek, and M. Viklander. 2019. “Laboratory Melting of Late-winter Urban Snow Samples: The Magnitude and Dynamics of Releases of Heavy Metals and PAHs.” Water, Air, & Soil Pollution 230 (8): 182. doi:https://doi.org/10.1007/s11270-019-4201-2.
- Vijayan, A., H. Österlund, J. Marsalek, and M. Viklander. 2021. “Estimating Pollution Loads in Snow Removed from a Port Facility: Snow Pile Sampling Strategies.” Water, Air, & Soil Pollution 232 (2). doi:https://doi.org/10.1007/s11270-021-05002-9.
- Viklander, M. 1999. “Substances in Urban Snow. A Comparison of the Contamination of Snow in Different Parts of the City of Luleå, Sweden.” Water, Air, and Soil Pollution 114 (3/4): 377–394. doi:https://doi.org/10.1023/A:1005121116829.
- Westerlund, C., and M. Viklander. 2011. “Pollutant Release from a Disturbed Urban Snowpack in Northern Sweden.” Water Quality Research Journal of Canada 46 (2): 98–109. doi:https://doi.org/10.2166/wqrjc.2011.025
- Westerlund, C., and M. Viklander. 2008. “Transport of Total Suspended Solids during Snowmelt – Influence by Road Salt, Temperature and Surface Slope.” Water, Air, and Soil Pollution 192 (1–4): 3–10. doi:https://doi.org/10.1007/s11270-007-9579-6.
- Westerlund, C., M. Viklander, K. Nordqvist, H. Galfi, and J. Marsalek, 2011. “Particle Pathways during Urban Snowmelt and Mass Balance of Selected Pollutants.” In 12th International Conference on Urban Drainage, Porto Alegre, Brazil, September 11–15.