Abstract
A new electron source and an electron collection source were found for the closure of the internal electron circuit inside fluorescent lamp (FL) tubes. The sources are formed in front of the polarized electric-insulator particles on the metal electrodes at both ends of the FL tube. The appearance and disappearance of both sources are controlled by the electric voltages applied to the metal electrodes. The electrons and Ar+ for the sources are generated by the ionization of the Ar atoms, and they return to the Ar atoms at the electron collection source. Thus, the Ar atoms are preserved in the FL tubes, ensuring a long operation life for such tubes.
1. Introduction
The studies of the lighting gases have a long history. More then 200 years have passed since discovery of lighting gases, and more than 90 years have passed since the invention of the practical fluorescent lamp (FL) tube Citation1. The structure of the FL tube is very simple. FL tubes are produced with only five parts; (i) vacuum-sealed glass tube, (ii) a pair of electrodes (cathode and anode), (iii) Ar gas around 5 Torr, (iv) Hg droplets, and (v) phosphor screen. They are visible by the eyes (i.e., the concrete materials). Furthermore, the annual production volumes of FL tubes in the world is in the billions Citation2. Everyone believes the production technologies of the FL tubes are mature technologies. We have carefully and critically analyzed the established technologies of the FL tubes in the publications. We have found that the production technologies of the FL tubes are not mature technology scientifically. In operation, the FL tubes essentially require (a) electron sources (cathode) and (b) electron collecting source (anode). The electron circuit inside an FL tube (i.e., internal electron circuit) should be closed by electron flow between cathode and anode. They are invisible by the eyes (i.e., the abstraction). We have found the vagueness of the electron source and electron collecting source to close the internal electron circuit. This report will describe the formation of the new electron source as a part of our clarifications.
2. Experiments
External-electrode FL (EEFL) tubes were used for the experiments. The EEFL tube samples were prepared as follows: the electrodes for the operation of the EEFL tube were installed on the outer wall at both ends of the FL glass tube by winding the plastic-covered lead wires a few turns. The wound lead wires were strongly pressed against the outer wall of the EEFL tube through the thermal shrinkage of the plastic tubes (transparent). The wound electrodes were separated from the Ar gas space inside the EEFL tube by the glass thickness (0.5 mm) and the plastic layer (∼0.5 mm) of the lead wires. No metal electrode was exposed in the Ar gas space in the EEFL tube.
The results were then applied to the ordinal FL tubes, in which, the metal electrodes at both sides were covered with layers of the electric-insulator particles. The examined FL tubes were operated with the external driving device (ballast) at the peak voltage (2 kV) and with 30 kHz frequencies.
3. Results and discussion
3.1. Internal electron circuit in the FL tube operation
The electrons from the external electrodes (EEs) of the EEFL tubes do not penetrate the glass walls of the FL tubes; as such, the EEs do not inject the electrons into the Ar gas space inside the FL tubes. The internal DC electron circuit in the EEFL tubes separates from the external AC driving circuit in the electron flow. The electron source (i.e., cathode) and electron collection source (i.e., anode) are necessary for the formation of an electric circuit inside the FL tubes. Then, the electron flow in the circuit closes between the cathode and anode inside the FL tube. The direction of the electron movement is from cathode to anode Citation2, opposite the direction in solids.
3.2. Electron sources in the FL tubes
The formation of electron sources is an important subject in the operation of FL tubes. It is believed that the commercial FL tubes use the thermoelectrons from (a) the heated BaO layers on the tungsten (W) filament coils (HCFL) and (b) the cold electrons from the metal cup (CCFL). These electron sources were well studied before their application to the FL tubes. Studies have also been conducted for the development of vacuum radio tubes and cathode ray tubes (CRTs).
The following is a brief summary of the electron sources emitted into a vacuum and a gas space. In 1884, T. A. Edison discovered electron emissions from a heated metal into a vacuum and a gas space (e.g., thermoelectron emission) Citation3. His results led to the W filament, which was heated to around 3000 °C in a vacuum and a gas space at low pressures. The emission of the thermoelectrons from the heated metal is the first generation of an electron source into a vacuum and a gas space. The heating temperature of the W filament remarkably drops to 800 °C from 3000 °C when the tiny particles of the superoxides (Mg-, Ca-, Sr-, and/or BaO), especially BaO, are coated onto the heated W filament Citation4. The heated superoxides, which emit thermoelectrons, are produced only through the decomposition of the carbonates at a low vacuum pressure (less than 10−5 Torr). If no superoxide is produced through the decomposition of the carbonates at a low vacuum pressure, the heated oxides will not emit electrons. The results led to the development of the vacuum radio tubes and CRTs. The W filament coils have been used to hold a large number of BaO layers on and in such coils.
In 1928, R. H. Flower and L. Nordheim reported that a sharp point (less than 1 μm) of the metal electrodes emits electrons into vacuum Citation5. The electron emission from the sharp point of the metal electrodes is the second generation of an electron source into a vacuum and a gas space. Such electron emission, called ‘cold electron emission’, led to the development of the field emission display (FED) Citation6.
3.3. Thermoelectron emission from heated BaO layers
In the studies on the electron emission from the heated BaO layers in a CRT, the actual electron emitters were assigned as the Ba atoms, which were arranged on the top BaO layer on the metal electrodes Citation7. illustrates the Ba atoms arranged on the top BaO layer on the metal electrode in a CRT. The heated BaO layers without Ba atoms on the top layer never emit electrons. The BaO layers without Ba atoms on the W filament coils are the electric insulators.
Figure 1. Structure of the thermoelectron emitter of the BaO layers on the metal in the CRT. The heated Ba atoms on the BaO layers emit thermoelectrons into the vacuum.
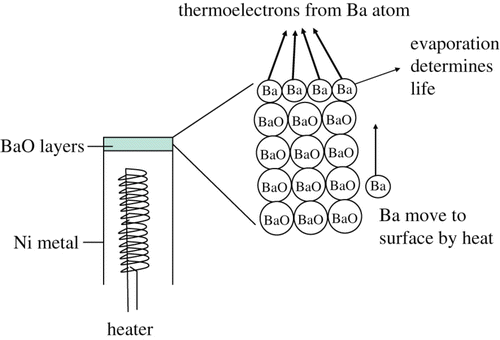
shows the equilibrium pressures (e.g., evaporation) of the Ba atoms and BaO as a function of the heating temperature. From , it is clear that Ba atoms preferentially evaporate from the BaO layer. The evaporation of the Ba atoms determines the life of the thermoelectron emission from the BaO layers. The operation life of the CRT is prolonged by the minimization of the evaporation of the Ba atoms.
In practice, the Ba atoms rapidly evaporate under the vacuum pressures above 10−4 Torr. The long-life CRTs are operated at the vacuum pressure below 10−7 Torr Citation7. The nominal HCFL tubes contain Ar gas pressures above 3 Torr and not 10−3 Torr, so the Ba atoms in the FL tubes instantly evaporate from the W filament coils, leaving the BaO layers that never emit thermoelectrons. The nominal HCFL tubes never have thermoelectrons from the heated BaO layers on the W filament coil. The electron source of the nominal HCFL tubes is another matter that has never been discussed in any report. The real electron source of such tubes will be reported elsewhere.
3.4. Cold electron emission from metal cup electrodes
The CCFL tubes have a similar story. The metal cup electrodes do not have sharp points, such as in the field emission array shown in [2, ]. The metal cup electrodes do not emit cold electrons into vacuum, but the metal cup electrodes in the Ar space may emit electrons into the Ar space. As the inner surface wall of a metal cup electrode is contaminated with the tiny powders of the electric insulators or metals, the Ar gas atoms are ionized inside the electrode. The ionized Ar space in the metal cup electrode has a mixture of (a) free electrons, (b) positively ionized Ar (Ar+), (c) excited Ar (Ar*), and (d) Ar atoms, including the vaporized metal atoms. Only excited Ar* atoms emit visible sky-blue light; the others are invisible to the eyes. The cup electrodes were developed as hollow cathodes for the light source of the atomic-absorption spectrometer Citation8. The electrons are extracted from the ionized Ar space inside the metal cup electrodes. shows a photograph of electrons being taken out from inside the metal cup electrode of a nominal CCFL tube. The extracted electrons collide (actually, Coulomb's repulsion) with the Ar and Hg atoms for the excitation. The excited Ar and Hg atoms emit sky-blue light, which can be seen by the naked eye.
Figure 3. Photograph showing that the lighted Ar and Hg gas atoms come from inside the metal cup electrode of the CCFL tube.
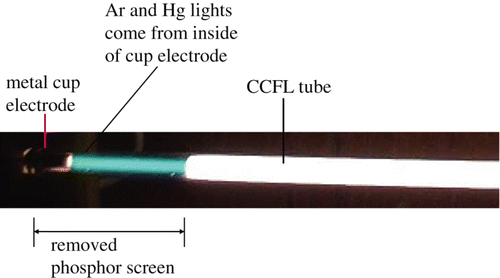
Figure 4. Schematic illustration of the mechanisms of the third-generation electron source in a vacuum chamber containing rare gases at above 3 Torr.
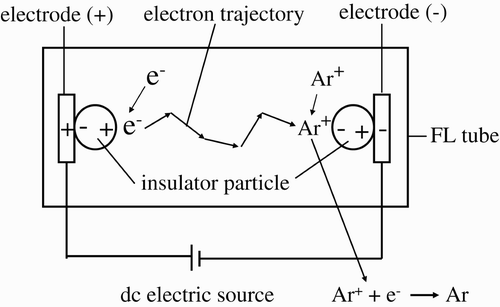
As described above, the practical HCFL and CCFL tubes do not use the first- and second-generation electron sources. The electrons emitted from the cathode reach the anode electrode, which collects the electrons from the gas space. In the early studies on the collection of electrons by the anode (before 1900), the shapes and sizes of the anode electrode were not considered important factors influencing electron collection. One may use a plate, cylinder, wire, or other materials for such purpose. The strange explanation for this involves the collection of electrons in the FL tubes. The W filament coils at both sides are covered with the layers of BaO particles (electric insulator). It has been said that the anode metal electrode covered with BaO layers in the HCFL tubes collects electrons from the Ar gas space without any difficulty. Further studies must be conducted on the electron sources and electron collection sources of the nominal HCFL tubes.
3.5. Third-generation electron source for FL tubes
A study that was conducted on the EEFL tubes explained many valuable properties relevant to the FL tube operation that have not been detected in the ordinal FL tubes. The EEFL tubes use neither first- nor second-generation electron sources. Both the electron source and electron collection source must be found so that the internal electron circuit in the vacuum-sealed FL tubes could be closed. Recently, the third-generation electron source, including an electron collection source, in the FL tubes was reported for such purpose Citation9.
The fundamentals of the lighted FL tube are the same as those of the different electrodes. Therefore, the FL tube rather than the EEFL tube was used for the experiments on the third-generation electron source. The formation of the third-generation electron source in an FL tube is explained herein using the diagram shown in . The FL tube contains Ar gas with 5 Torr pressure, and the metal electrodes at both ends are covered with electric-insulator particles. As they are also covered with a thin layer of frit glass (not shown in ), the insulator particles adhere to the surfaces of the metal electrodes. Such electrodes covered by a thin layer of frit glass are similar to the EEFL tube and do not inject electrons into the vacuum in the FL tube. The third-generation electron source and the electron collection source are formed in front of the polarized insulator particles (equivalent to the glass wall) on the electrodes, at both ends.
The details of the formation of the third-generation electron source and electron collection source in front of the polarized insulator particles on the metal electrodes will be described herein. The necessary condition for the formation of the sources is the polarization of the electric-insulator particles on the metal electrodes. As an insulator particle is placed under the electric field F from the metal electrode, such particle undergoes induced polarization due to the deformation of its crystal structure. The polarity inside the polarized particles that attach onto the electrode is opposite the polarity of the electrodes. The polarity at the exposed side of the gas space coincides with that of the metal electrodes, as illustrated in . The electrodes are connected to a DC power supply. The amount of polarized charges (Q) in the insulator particle at one side is a linear function of the dielectric constant , where F is given by
where V e is the voltage applied to the electrodes, and r is the distance from the surface of the electrode to the particle. Therefore,
The value r is minimized by the adhesion of the insulator particle on the surface of the metal electrodes. If the electrodes settle on the surface of the outer glass tube, they must be considered part of the thickness of the glass tube (e.g., 0.5 mm). Q of the EEFL tube then becomes a small Q value. As the insulator particles settle on the surfaces of the metal electrodes in the FL tube, the electric field V part applied to the Ar gas space is amplified from V e of the electrodes. The high voltage applied to the Ar gas space gives the arc current in the low Ar gas pressure of the ordinal FL tube (5 Torr). Adjustment of the applied V e and Ar gas pressure is definitely requested for the operation with the structure shown in . The optimization of the Ar gas pressure and applied voltages of the ordinal FL tubes is under way. The nominal CCFL tubes use the third-generation electron source and thus have Ar gas pressures of around 70 Torr to avoid the arc current. The amplification of the voltages applied to the Ar gas space is described below.
The insulator particles may be used in general, but the preferred insulator particles are those formed by asymmetric crystals, which induce a large amount of polarized charges through the large mechanical deformation of the crystals. As the asymmetric particles adhere on the entire surfaces of the metal electrodes of the FL tubes, they instantly exhibit an electric field much higher than Ve. The commercial phosphor particles (crystals) are asymmetric particles. According to Laporte's rule, optical transitions are allowed only between electric states with different parities. This parity selection rule is removed when the luminescent centers are located at the lattice sites that lack a center of inversion symmetry. The probability of the allowed electric transitions in an asymmetric field is extremely high compared with that in a symmetric field Citation10. All the host crystals that are used in the commercial phosphor particles do not have inversion symmetry. This explains why the commercial phosphor particles have a high energy conversion efficiency (η values). The commercial phosphor particles are the suitable insulator particles for the layers on the metal electrodes in the FL tube. This explains why the EEFL tube forms an internal electron circuit.
The optimum conditions of the insulator particles on the electrodes may be calculated Citation11. The total charges induced in the insulator particles on the electrodes are proportionate to the total surface area of the insulator particles: large on the large surface area. If the entire surface area S
0 of the metal electrode is covered with a single layer of insulator particles, the total surface area S of the insulator particles will be given by π S
0 regardless of the particle size. S on the electrode is a function of the number of layers of particles: a large S with a large number of layers. Practically, the surface of the electrode is covered with three to five layers of insulator particles with acceptable adhesion on the metal electrodes. The Q value also decreases with the large number of particle layers (with a large r to the top layer). Better adhesion of the insulator particles on the metal electrodes is achieved by screening the slurry, which is a mixture of the insulator powder (90 wt.%) and the crystallized frit glass powder (10 wt.%).
It should be noted that the layers of the electric-insulator particles on the electrodes act as the capacitance. As such, a large number of insulator layers on the electrodes will lead to an increase in the capacitance C tube values. The AC in the external driving circuit relates to the value of C tube. The optimized conditions are (a) a few layers of the insulator particles, and (b) an average insulator particle size of around 4 μm, which was determined with the use of a microscope.
Two conditions (necessary and sufficient) are required for the formation of both sources in front of the insulator particles on the metal electrodes in the FL tubes. The necessary condition is the polarization of the particles on the metal electrode, and the sufficient condition is the ionization of the Ar atoms around the polarized insulator particles.
For the ionization of the Ar atoms around the polarized insulator particles, the metal electrodes, which are covered by layers of insulator particles, settle in the Ar gas space inside the FL tube. The Ar atoms around the polarized insulator particles are instantly ionized under the high electric field V
part, with high frequencies, but there is a threshold condition. The Ar atoms ionized in the high frequency (20 kHz) apply to the electrodes of the FL tube. The Ar atoms do not ionize with the V
e (<500 V) of the frequencies below 1 kHz. Through ionization, the Ar atoms separate into Ar+ and free electrons, which are attracted by the countercharges inside the polarized insulator particles. Attracted charges do not penetrate the insulator particles but stay in the vacuum and bind with the countercharges inside the polarized insulator particles in the FL tube. The electrons bind with the positive charges in the polarized insulator particle, and Ar+ binds with the negative charges in the polarized insulator particles.
The amount of bound charges on the given insulator particles increases with V part. A large amount of bound charges is accumulated in front of the insulator particles with a high V part. The accumulated electrons and Ar+ in front of the insulator particles form an electron source and an electron collection source. The appearance and disappearance of both sources in front of the insulator particles are controlled by the electric voltages applied to the metal electrodes. As the response time of the appearance and disappearance of both sources is fast, the sources are operated by the AC-applied voltages with frequencies ranging from 20 to 60 kHz.
The negative field from the orbital electrons of the Ar atoms fills the vacuum between such atoms Citation12. The Ar atoms around the electron source are ionized by the electric field from the electrodes. As such, there are many Ar+ atoms and electrons around the electrodes. As the Ar+ atoms do not emit light, the presence of Ar+ atoms and electrons in front of the insulator particles cannot be perceived by the naked eye. The presence of Ar+ atoms and electrons may be confirmed by the lighting of the Ar (and Hg) atoms by the moving electrons. The presence of Ar+ atoms neutralizes the negative field in the vacuum between the Ar atoms. The neutralization of the negative field in the vacuum serves as a trigger for the moving electrons. Consequently, the electrons from the electron source move into the neutralized vacuum between the Ar atoms. The difficulty in triggering the lighting of Ar atoms can be addressed by the application of the third-generation electron source. The movement of the electrons in the FL tubes is controlled by the (a) longitudinal electric field (vector) and (b) scattering of the moving electrons with Coulomb's repulsion from the Ar atoms and negative charges on the phosphor screen (scalar products).
As there are many available electrons around the polarized insulator particles, the binding force of the individual bound electrons with positive charges in the polarized insulator particles fluctuates with time. The bound electrons in a state of fluctuation are smoothly removed by the longitudinal electric field. Thus, an electron source is formed by the bound electrons in front of the polarized insulator particles at the end of an FL tube, and by the positive charges (bound Ar+ atoms) on the polarized insulator particles at the other end. The lighting of the gas atoms in the FL tube is generated by the moving electrons between the electron source and the accumulated positive charges at the other end of the FL tube. The electrons that arrive at the accumulated positive charges recombine with the Ar+ atoms, and the Ar+ atoms return to the Ar atoms. The returned Ar atoms are again ionized by the electric field of the polarized particles. The ionization of the Ar atoms supplies electrons and positive ions for the formation of both an electron source and an electron collection source during the operation of the FL tubes. No Ar atom consumption is required in the operation of the internal DC electron circuit; the Ar atoms in the FL tubes are preserved in the operation of such tubes.
A significant feature of the use of the third-generation electron source in the FL tubes is that there is no sputtering of cathode metal electrodes, which gives rise to a stable Ar gas pressure in the operation of the FL tubes. The results ensure a very long operation time of more than 100,000 h, with the initial brightness. Life tests of the FL tubes are under way.
3.6. Difference between the third-generation electron source and dielectric barrier discharge
The difference between the third-generation electron source and the reported dielectric barrier discharge must be clarified Citation13. The third-generation electron source has an electron source and an electron collection source for the closure of the internal electron circuit in the FL tube. On the other hand, the dielectric barrier discharge does not have an electron source and an electron collection source. This is the basic difference between the two. A question then arises as to what the discharge means as it relates to physics. Webster's Dictionary defines discharge as ‘the unloading of a ship or cargo’, and (elect.) ‘the equalization of a potential difference at two points in an electric field by the charge flow from one point to the other’. If the word discharge is used to mean lighting of the gas atoms, the word is misused, which leads to the vagueness of the lighting of the gas atoms. The lights do not gush out from the vacuum. The lighting of the gas atoms is generated solely by the atoms’ excitation. As gas atoms are electrically neutral and have heavy masses, they do not move in the Ar gas space. The excitation of the gas atoms is achieved by sampling the moving electrons between the electron source and the electron collection source. If the moving electrons will be considered in the dielectric barrier discharge, where do such electrons come from, and where do they go after the closure of the electron circuit? The description of the dielectric barrier discharge did not answer these questions.
If the wavelength of the electron (64 Å) will be considered in the formation of the electron source, the electrons may penetrate the insulator layer at a thickness of 10 nm. The diameter of the atoms is about 0.6 nm. If the insulator layer of the metal cathode is made up of 15 atomic layers of insulator atoms (9 nm thickness) at most, the electrons of the metal electrode may penetrate the insulator layers and may proceed to the vacuum. As the insulator particles that are generally used within FL devices are in micrometers, the thicknesses of the insulator layers in the FL devices should also be in micrometers. There is no rationalization of the electron source (i.e., cathode) in the explanation of the dielectric barrier discharge. No explanation of the collection of electrons (i.e., the role of the anode) in the gas space is given in the description of the dielectric barrier discharge. Thus, if there are moved electrons, where are they accumulated? Furthermore, ionized ions do not emit light; only excited gas atoms do. The third-generation electron source and the electron collection source principally differ from the dielectric barrier discharge.
3.7. Characteristic properties of EEFL tubes
Here is a problem. If the third-generation electron source is applied to the ordinary HCFL and CCFL tubes, which have below 5 Torr Ar gas pressure, the arc current with a high V part to the Ar gas space may be observed. The mean free path of the moving electrons in the FL tubes is determined by the (a) magnitude of the electric field applied to the gas space, and (b) gas pressures. To avoid the arc current, the FL tubes have high Ar gas pressures. The simply increased Ar gas pressures give rise to the large capacitance, C tube, to the external AC driving circuit Citation1. The authors do not have the proper driving devices for wide-diameter FL tubes. The narrow FL tubes (<3 mm diameter) have an advantage in terms of the application of the third-generation electron source. With this reason, the following experiments were performed with EEFL tubes with 2.5 mm outer diameters. The Ar gas pressures were 70 Torr.
The improvement of the luminance of the EEFL tubes has been achieved through trial and error, without finding the reasons for the dim luminance. It is not hard to find a way to increase the luminance of the EEFL tubes as it linearly increases with V e to the electrodes. shows, for instance, the results of (a) the increase in luminance from the phosphor screen of the EEFL tubes, and (b) the DC internal electron current of the EEFL tubes as a function of the voltage applied (V e) to the electrodes between 1 and 6 kV. The results show that a high V e was applied to the electrodes of the EEFL tubes to increase the luminance.
The kinetic energies of the moving electrons linearly increase with V e. The Hg atoms are excited only with the moving of the electrons that have kinetic energies between 6.7 and 10.3 eV. Only the electrons attenuated above the aforementioned range can excite the vaporized Hg atoms. The attenuation of the kinetic energies higher than the excitation energy is achieved through the ionization of Ar+. It was assumed that the moving electrons lose 15.7 eV kinetic energy with each collision. The numbers of Ar+ atoms in the given EEFL tubes linearly increase with V e. The ionization of the Ar atoms heats the Ar gas space in the EEFL tube by changing the entropy. The temperature of the heated Ar gas space determines the number of vaporized Hg atoms from the droplets. The increase in luminance from the phosphor screens shown in was caused by the increase in the numbers of vaporized Hg atoms in the heated Ar gas spaces in the EEFL tubes Citation12 and not by the increase in the number of electrons. In solids, the numbers of electrons are proportionate to the voltages applied to the electrodes. In the Ar gas space, the number of electrons does not increase with the voltages applied to the electrodes.
The internal electron currents were also measured with a high-resistance probe. The constant electron current (0.2 mA) was measured within the experimental range (2–6 kV) of the EEFL. The results show that the increase in luminance was not caused by the increase in the electron current. The incredibly small numbers of the internal electron current (0.2 mA) in are in accordance with the electron currents calculated from the number of photons in the FL tubes Citation12.
There are many factors that can control the quality of FL tubes that are yet to be clarified. They are (a) a degassing process from a glass tube and phosphor screen, (b) a large amount of degassing from a melting glass tube for sealing from pumping facilities, (c) the degassing of an Hg droplet, (d) the electric properties of a phosphor screen, (e) the binding of phosphor particles to the inner wall of a glass tube, (f) the driving devices of the FL tubes, etc. A study on these is under way.
4. Conclusion
The principle of a new electron source and electron collection source for the internal electron circuit was studied. The new sources are formed in front of the polarized insulator particles on the metal electrodes. The formation of the sources is controlled by the voltages applied to the metal electrodes. The appearance and disappearance of the source are synchronized with the AC frequencies up to 50 kHz. The sources originate from the electrons and Ar+, which are generated by the ionization of the Ar atoms. The internal electron circuit is closed at the electron collection source by recombining the electrons and Ar+ for a return to the Ar atoms. The Ar atoms are preserved during the operation of the FL tube, ensuring a long operation life. A practical study on FL tubes applying the third-generation electron source must be conducted in the future.
Acknowledgements
The authors wish to express their gratitude to Mr. J. C. McKain (USA) for his help with the language of the text.
References
- F. Meyer, US Pat. 2,182, 732 (1928)
- Annual Report (2007) from Japanese Department of Economy, Industry and Machinery
- T.A. Edison, USP 307,031 (1884)
- Whenelt , W. 1904 . Ann. Phys. Leipzich , 14 : 425
- Flower , R. H. and Nordoheim , L. 1928 . Proc. Roy. Soc. Lond. Ser. A , 119
- Spindt , C. A. , Brodie , L. , Hemphrey , L. and Westerberg , R. E. 1976 . J. Appl. Phys. , 47 : 5248
- Ozawa , L. 1996 . Disp. Imag. , 5 : 59 in Japanese
- Yasuda , K. and Hasegawa , K. 1972 . Analysis by Atomic Absorption Spectrometer , 60 Tokyo, , Japan : Kodansha Scientific .
- WO 2009/050816 A1, PCT/JP2007/070431
- Shionoya , S. and Yen Eds , W. M. 1998 . Phosphor Hand Book , Boca Raton, FL : CRC Press, Taylor & Francis Group .
- Ozawa , L. 2007 . Cathodoluminescence and photoluminescience , Boca Raton, FL : CRC Press, Taylor & Francis Group .
- Ozawa , L. and Tian , Y. 2010 . J. Inform. Disp. , 11 : 128
- Utabe , T. , Harada , S. , Saikatsu , T. and Karino , M. 1996 . J. Light Vis. Eng. , 20 : 20