ABSTRACT
In this work, polymer-stabilized simple cubic blue phase (PSBPII) samples with three reflective elementary colors (red, green, and blue) were successfully fabricated. The PSBPII samples showed uniform alignment after rubbing treatment, and a wide temperature range of over 40–50°C, including room temperature. They also exhibited robustness against lattice deformation by an applied electric field.
1. Introduction
Chiral liquid crystals exhibit self-assembled periodic structures, giving rise to photonic band structures [Citation1,Citation2]. Among the liquid crystalline phases, cubic blue phases (BPs) have a double-twisted cylinder (DTC) structure, and the self-assembly of DTCs in a three-dimensional space determines their unique periodic lattice structures [Citation3]. Cubic BPs can be broadly categorized into two types: a simple cubic BP (BPII) and a body-centered cubic BP (BPI), in the order of decreasing temperature [Citation4–6]. Unfortunately, each cubic BP appears only over a narrow temperature range, typically 0–2°C, between the high-temperature isotropic (Iso) phase and the low-temperature cholesteric (N*) phase [Citation4–6]. For this reason, polymer stabilization methods have frequently been employed to expand the temperature ranges at which cubic BPs can exist [Citation3,Citation7–8]. Although polymer-stabilized (PS) BPI samples possessing wide temperature ranges (several tens of degrees Celsius), however, have been reported by several groups [Citation8–10], such wide temperature ranges for PS simple cubic blue phase (PSBPII) samples have been rarely achieved [Citation7]. This is because the temperature ranges in which a pure BPII appears are narrowly compared with those in which pure BPI appears, and as such, in situ polymerization is more difficult in BPII than in BPI. Thus, BPII possesses a very tight process window [Citation7].
Recently, the authors succeeded in fabricating PSBPII over a temperature range of 50°C, including room temperature (RT) [Citation7]. The approach that was employed was as follows. First, a host chiral nematic mixture (HN*M) consisting of the conventional nematic liquid crystals (NLCs) and bent-core (BC) molecules were prepared. By doping the BC molecules, the temperature ranges of both pure BPI and BPII were successfully expanded. Next, a precursor consisting of the HN*M and photopolymerizable monomers was prepared. The prepared precursor showed supercooled BPII, with a temperature range of ∼10°C. By employing this broad process window, in situ polymerization in BPII was easily carried out; consequently, PSBPII was successfully fabricated. Only PSBPII with a green reflective color was presented in the authors’ previous work [Citation7].
In the present study, an attempt was made to obtain PSBPII with three reflective elementary colors (red, green, and blue). By controlling the amount of chiral dopant, PSBPII with red, green, and blue reflective colors were fabricated. PSBPII tended to easily achieve a relatively uniform alignment via rubbing treatment. The fabricated PSBPII samples exhibited a wide temperature range of over 40°C, including RT. In addition, PSBPII showed robustness against lattice deformation by an applied electric field. It is anticipated that the robust and monodomain-like PSBPII will have applications in high-performance photonic crystal devices.
2. Materials
Three precursors (P-R, P-G, and P-B, corresponding to the colors red, green, and blue, respectively) consisting of HN*Ms and photopolymerizable monomers were prepared. By controlling the amount of chiral dopant, a variety of reflective colors were successfully expressed. Only the typical three colors were presented in this work, however, to evaluate the characteristics of PSBPII. The HN*Ms consisted of commercial rod-like NLCs (HTW109100-100, HCCH), BC molecules, and chiral dopants (S811, Merck). The BC molecules were synthesized. The details of the mixing fractions are summarized in Table . The BC molecule that was used was different from the one that was used in the authors’ previous work [Citation7]; it possessed alkyl chains (instead of alkoxy chains) at the terminal positions. Although the terminal chains of the BC molecules were different, the same doping effect was observed; that is, the temperature range of the BPs was expanded by adding the BC molecules. The details of the doping effect for the temperature range of the BPs are given in Ref. [Citation5]. To prepare the precursors for stabilizing BPII, two types of photopolymerizable monomer were used, which are generally employed to fabricate PSBPs: TMPTA (Aldrich) and RM257 (Merck). The chemical structures of the BC molecule, TMPTA, and RM257 are shown in Figure .
Table 1. Constituent fractions of the precursors prepared in this work (wt%).
3. Results and discussion
3.1. BPII in precursors
The prepared precursors were used to infiltrate sandwich cells (cell gaps 5 μm) composed of two glass substrates coated with a unidirectionally rubbed, polyimide-based alignment layer (AL22620, JSR) at an ISO temperature. Figure shows the typical polarized optical microscopic (POM) images for P-R, P-G, and P-B during the cooling process (1°C/min). As shown in Figure , unknown phases with red, green, and blue reflective colors appeared in the ISO phase in P-R, P-G, and P-B, respectively. Interestingly, the unknown phases observed via POM showed monodomain-like uniform textures and were changed to N* with oily streaks upon further cooling. Figure shows plots of the temperature dependence of the maximum Bragg reflection wavelengths of P-R, P-G, and P-B, respectively. The said figure shows that discontinuous changes at the unknown phase -N* transition during the cooling process were clearly observed in all the three precursors. In the unknown uniform phases that were observed in all the three cases, the maximum Bragg reflection wavelengths shifted towards slightly shorter wavelengths with decreasing temperature. This trend was similar to that observed in the previous studies on BPII [Citation4,Citation6–7]. In addition, the unknown phases showed a monodomain-like texture in the surface-treated cells. In general, it was found that BPII with a monodomain-like phase could be easily realized in the surface-treated cells. Hence, it was concluded that the unknown uniform phases were BPII, whose range was expanded owing to the supercooling effect. The estimated temperatures of the supercooled BPII of the prepared P-R, P-G, and P-B in this work were about 25°C, 22°C, and 10°C, respectively. The temperature range of BPII decreased with strong chirality. Hence, the temperature window decreased with increasing chiral dopant.
3.2. PSBPII
Next, an attempt was made to stabilize the supercooled BPII with a wide temperature range via polymer networks. Three kinds of precursors were added to small amounts (0.2 wt%) of photoinitiator (DMPAP), and were injected into surface-treated sandwich cells at an ISO temperature. The cells were slowly cooled (1°C/min) to 35°C, at which BPII was maintained. The cells filled with the precursors were then irradiated with UV light (365 nm, 400 mW/cm2) for 10 min to stabilize the supercooled BPII of the precursors.
Figure shows the typical POM images and the Bragg reflection spectra for a range of temperatures for PSBPII with red, green, and blue reflective colors. All the stabilized phases exhibited monodomain-like uniform textures with relatively high reflection. The high reflective properties were due to the uniform textures and indicate potential applications in versatile photonic devices.
Figure shows the temperature dependence of the reflection peak wavelength for a range of temperatures for PSBPII with red, green, and blue reflective colors. Constant reflection peaks at around 620 nm (red), 555 nm (green), and 480 nm (blue) were observed over a wide temperature range in all the PSBPII samples fabricated in this work. The BPII lattices were stabilized by the polymer network; thus, there was barely any shift in the Bragg reflection wavelengths [Citation7]. These results demonstrate the successful fabrication of PSBPII over a temperature range of 40–50°C, including RT.
Figure 5. Temperature dependence of the reflection peak wavelength for a range of temperatures for PSBPII with red, green, and blue reflective colors.
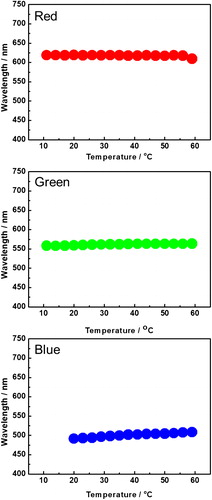
Figure shows the reflectance spectra of the PSBPII samples with red, green, and blue reflective colors for different voltages at RT. As the electric field increased, the LC molecules were reoriented along the electric field, and the DTC structures were gradually unwound; as such, the reflectance decreased (Figure (a)). When most of the LC molecules were reoriented parallel to the electric field, the PSBPII samples lost their unique periodic structures, and the reflectance was reduced to minimum [Citation7,Citation11]. By contrast, when the electric field was decreased, the samples reverted to their original reflectance (Figure (b)). In addition, the PSBPII samples showed robustness against lattice deformation because there was barely any shift in the Bragg reflectance wavelengths when the samples were subjected to an applied electric field. For PSBPI, the independent defect lines stabilized by the polymers do not contact one another inside the unit lattice [Citation7,Citation12]. By contrast, the defect lines of BPII have an intersection point at the center of the unit lattice [Citation7,Citation12]. Hence, PSBPII is expected to be more robust against lattice deformation by an applied electric field compared with PSBPI.
4. Summary
Here, PS simple cubic blue phase (PSBPII) samples with red, green, and blue reflective colors were successfully fabricated. PSBPII tended to easily achieve a relatively uniform alignment via rubbing treatment. The fabricated PSBPII samples exhibited a wide temperature range of over 40–50°C, including RT. They also showed robustness against lattice deformation when subjected to an applied electric field. These PSBPII materials appear promising for application in photonic devices.
Disclosure statement
No potential conflict of interest was reported by the authors.
Additional information
Funding
Notes on contributors
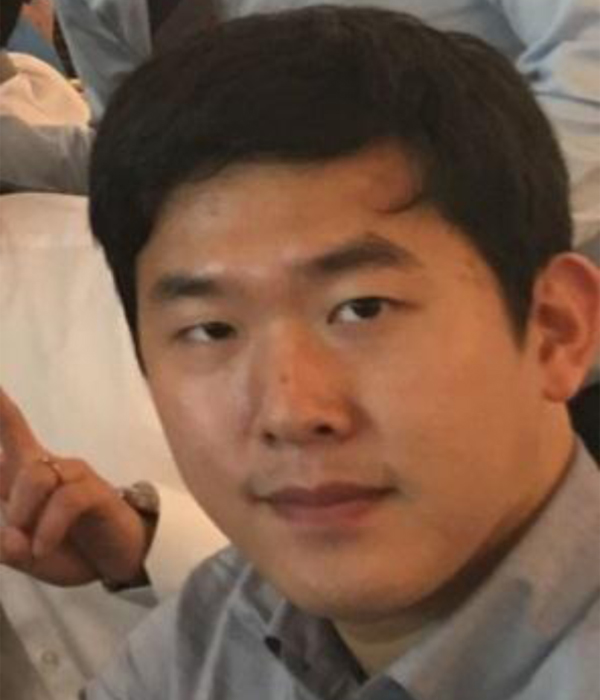
Jae-Hyun Bae
Jae-Hyun Bae obtained his B.S. degree from the Department of Advanced Material Engineering for Information & Electronics of Kung Hee University, South Korea in 2015. He is currently an M.S. candidate in the same university and has been researching on photonic crystals related to liquid crystalline materials.
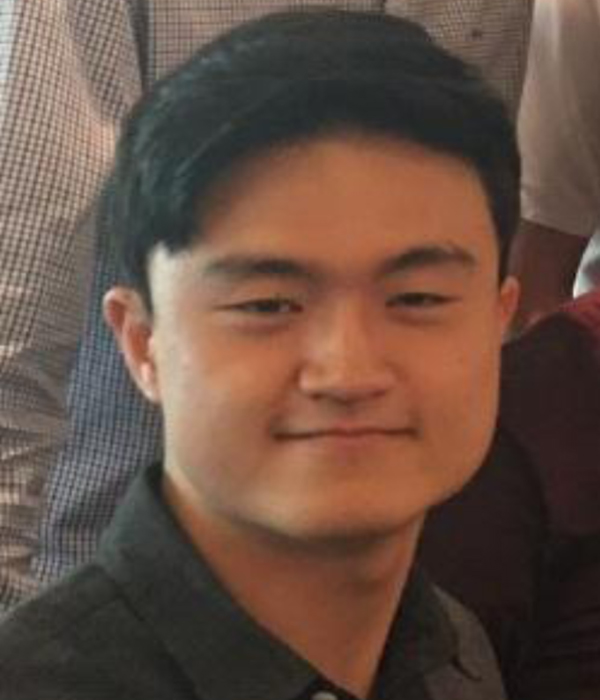
Byeong-Cheon Kim
Byeong-Cheon Kim obtained his B.S. degree from the Department of Advanced Material Engineering for Information & Electronics of Kung Hee University in South Korea in 2017. He is currently an M.S. candidate in the same university and has been researching on liquid crystalline materials related to chirality.
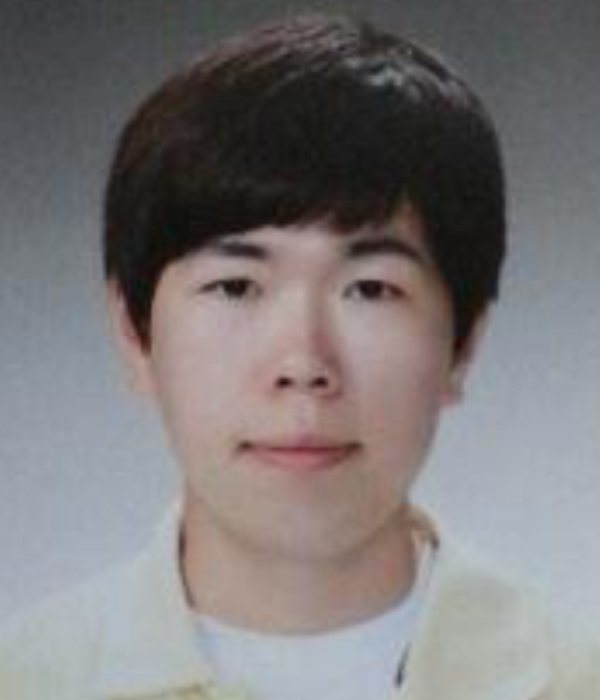
Seong-Yong Jo
Seong-Yong Jo obtained his B.S. and M.S. degrees from the Department of Advanced Material Engineering for Information & Electronics of Kung Hee University in South Korea in 2015 and 2017, respectively. He is currently a Ph.D. candidate in the Graduate School of Engineering of Osaka University in Japan. He has been researching on photonic crystals and metamaterials related to liquid crystalline materials.
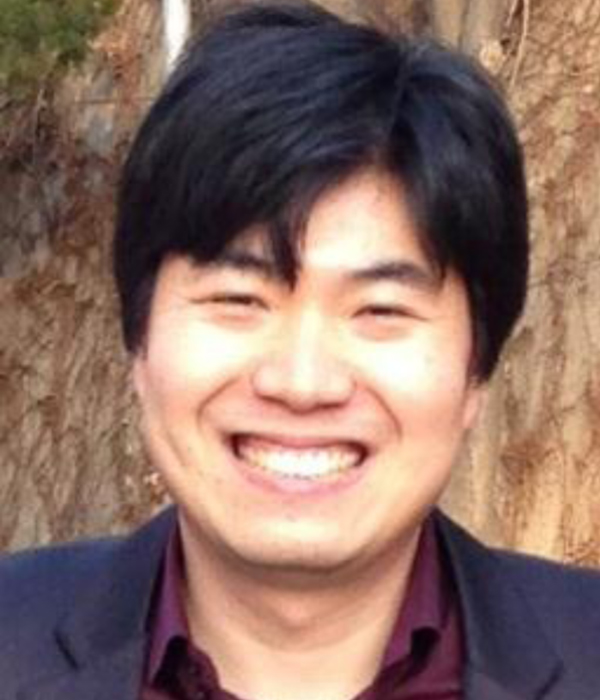
Suk-Won Choi
Suk-Won Choi is a professor in the Department of Advanced Material Engineering for Information & Electronics of Kyung Hee University in South Korea. He obtained his B.S. degree from Hanyang University in South Korea, and his M.S. and Ph.D. degrees from Tokyo Institute of Technology in Japan. Since joining the faculty of Kyung Hee University in 2008, he has been researching on the liquid crystalline materials for optics and photonics applications.
References
- D. Pijper and B.L. Feringa, Soft Matter. 4, 1349–1372 (2008). doi: 10.1039/b801886c
- S.-T. Hur, B.R. Lee, M.-J. Gim, K.-W. Park, M.H. Song and S.-W. Choi, Adv. Mater. 25, 3002–3006 (2013). doi: 10.1002/adma.201204591
- H. Kikuchi, Struct. Bond. 128, 99–117 (2008). doi: 10.1007/430_2007_075
- K.-W. Park, M.-J. Gim, S. Kim, S.-T. Hur and S.-W. Choi, ACS Appl. Mater. Interf. 5, 8025–8029 (2013). doi: 10.1021/am403109q
- S.-T. Hur, M.-J. Gim, H.-J. Yoo, S.-W. Choi and H. Takezoe, Soft Matter. 7, 8800–8803 (2011). doi: 10.1039/c1sm06046e
- K. Kim, S. Kim, S.-Y. Jo and S.-W. Choi, J. Inf. Disp. 16, 155–160 (2015). doi: 10.1080/15980316.2015.1062809
- S.-Y. Jo, S.-W. Jeon, B.-C. Kim, H.-H. Bae, F. Araoka and S.-W. Choi, ACS Appl. Mater. Interf. 9, 8941–8947 (2017). doi: 10.1021/acsami.7b01502
- H. Kikuchi, M. Yokota, Y. Hisakado, H. Yang and T. Kajiyama, Nat. Mater. 1, 64–68 (2002). doi: 10.1038/nmat712
- J. Yan and S.-T. Wu, Opt. Mater. Express. 1, 1527–1535 (2011). doi: 10.1364/OME.1.001527
- Y.-H. Chen, C.-T. Wang, C.-P. Yu and T.-H. Lin, Opt. Express. 19, 25441–25446 (2011). doi: 10.1364/OE.19.025441
- J. Yan, S.-T. Wu, K.-L. Cheng and J.-W. Shiu, Appl. Phys. Lett. 102, 081102 (2013). doi: 10.1063/1.4793750
- H. Choi, H. Higuchi, Y. Ogawa and H. Kikuchi, Appl. Phys. Lett. 101, 131904 (2012). doi: 10.1063/1.4752461