ABSTRACT
In this study, photoresponsive (PR) nanosegregated mesophases were prepared from a mixture system consisting of nonchiral bent-core (BC) and PR rod-like mesogens. The circular dichroism (CD) intensities depended strongly on the thermotropic polymorphism (nematic or smectic A) of the PR mesogen embedded in the BC molecular helical nanofilaments. The CD intensities could be reversibly modulated by alternating irradiation with UV and visible light in the nanosegregated mesophases. The study results presented interesting information relevant to the development of novel chiroptical materials.
1. Introduction
The intriguing chiral phenomenon in nonchiral bent-core (BC) liquid crystal (LC) phases has generated much interest since the discovery of a spontaneous chiral resolution in nonchiral BC molecular systems [Citation1–4]. The LC phases observed in BC molecular systems termed as ‘banana phases’ are specific mesophases that are different from the conventional LC phases found in rod-like mesogens [Citation5,Citation6]. Among the different ‘banana phases,’ the helical nanofilament (HNF) phase shows a hierarchical chirality that originates from a unique chiral superstructure attributable to the nanofilaments of twisted layers formed through the self-assembly of nonchiral molecules [Citation5–7]. As the BC molecules are nonchiral, two chiral domains possessing opposite twisted superstructures spontaneously appear with equal probability in the HNF phase [Citation1,Citation2].
A mixed system consisting of nonchiral BC and rod-like mesogens has been reported as a novel chiroptic material [Citation8–14]. Especially, mixed systems consisting of nonchiral BC and photoresponsive (PR) rod-like mesogens are promising as photomodulating chiroptical switching materials [Citation13,Citation14]. Kim et al. reported the first example of a mixture of nonchiral BC molecules and PR LC molecules possessing a nematic (N) phase [Citation13]. The PR molecules that were used had an azobenzene linkage; the trans isomer of the molecules stabilized the N phase, whereas the cis isomer exhibited a tendency to disturb the N phase [Citation15]. Furthermore, Kim et al. observed a nanosegregated <HNF/N> phase in the mixture. <HNF/N> indicates a nanosegregated phase in which the BC molecules are in the HNF phase and rod-like molecules are in the N phase in the mixture. A circular dichroism (CD) signal arising from a chiral superstructure was detected in this <HNF/N> phase. The detected CD signal intensity decreased after UV irradiation. Interestingly, the decreased CD signal intensity could be reverted to the initial CD signal intensity with visible-light irradiation [Citation13]. Thus, a reversible photomodulating chiroptic switching behavior could be realized in the <HNF/N> phase. The authors posited that this reversible chiroptical modulation could be used for rewritable optical storage, among other applications. More recently, Jeon et al. prepared another mixture system consisting of nonchiral BC and PR mesogens, which was different from the molecule system that was used by Kim et al. [Citation14]. In this case, the authors reported the existence of a nanosegregated <HNF/SmA> phase. The <HNF/SmA> phase is a nanosegregated phase in which the BC molecules are in the HNF phase and the rod-like molecules are in the smectic A (SmA) phase in their mixture. It was found that reversible chiroptic modulation could also be realized in the <HNF/SmA> phase [Citation14].
In this study, nanosegregated <HNF/N> and <HNF/SmA> phases were prepared from the same mixture system consisting of nonchiral BC and PR rod-like mesogens. The use of this system allowed the direct comparison of the photomodulating chiroptic behaviors in the <HNF/N> and <HNF/SmA> phases. The study results presented interesting information relevant to the development of novel chiroptical materials.
2. Materials
In this study, a mixture of nonchiral BC (30 wt%) and PR rod-like mesogens (70 wt%) was prepared. Figure shows the chemical structures of the compounds that were used in this study. The PR rod-like mesogen was a mixture of 8CB (90 wt%) and 4-butyl-4′-methoxyazobenzene (BMAB, 10 wt%). The prepared PR mesogen displayed N and SmA phases upon the cooling of its isotropic (Iso) mixture (Iso-41°C–N-30°C-SmA) (Figure (a)). In contrast, the two mesophases (N and SmA) disappeared upon UV irradiation (λ = 365 nm, 30 mW cm−2), as shown in Figure (b). This is because the BMAB molecule possesses an azobenzene linkage; the trans form of BMAB stabilizes the mesophases of PR mesogen, whereas its cis isomer tends to destabilize the mesophases, resulting in the Iso phase [Citation15].
3. Results and discussion
Figure shows the transmittance intensity of light (λ = 633 nm) as a function of temperature for the mixture of BC and PR mesogens in this study. The mixtures were injected into a sandwich cell (cell gaps: 10 mm) consisting of two glass substrates without surface treatment. The light transmitted through the cell was monitored under cross-polarizers using a photodiode. As no absorption occurred at λ = 633 nm for either the BC or PR molecules, the observed transmittance intensities were related only to the birefringence of the prepared mixture. After decreasing the temperature from that of the Iso mixture, two transition points were checked near 41°C and 30°C, which coincided with the phase transition temperatures of the PR mesogens blended with the BC molecules. Based on the temperature dependence of transmittance and the previous reports, three nanosegregated phases – <HNF/Iso>, <HNF/N>, and <HNF/SmA> – were identified with decreasing temperature in the mixture in this study [Citation7,Citation14]. In general, the birefringence of SmA is larger than that of N. Hence, the transmittance of <HNF/SmA> was higher than that of <HNF/N>.
Figure 3. Transmittance (λ = 633 nm) as a function of temperature of the mixture of BC and PR mesogens.
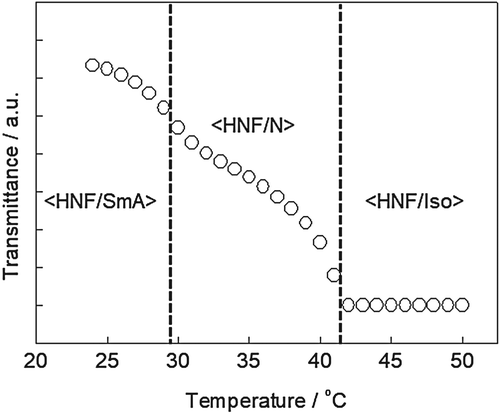
Figure shows the typical CD profiles at the three aforementioned nanosegregated phases. To observe the CD spectra, the mixture was injected into a thin sandwich cell (cell gaps: 1 μm) consisting of two smooth quartz substrates without surface treatment. The cells were then monitored using a CD spectrometer (J-720WI, Jasco). Of the two chiral domains that appeared spontaneously with equal probability, only the positive chiral domain was focused on. Here, the chiral domains possessing a positive sign at λ = 300 nm were regarded as positive chiral domains. The CD intensities in the mixture depended strongly on the thermotropic polymorphism of the RC mesogen embedded in the BC molecular HNFs [Citation14]. Among the three different phases before UV irradiation, the largest CD intensity was detected in <HNF/SmA>. The differences in CD intensity among the three different phases (<HNF/Iso>, <HNF/N>, and <HNF/SmA>) were due to the different chiral superstructures in such phases [Citation14]. The CD profiles after UV irradiation are also shown in Figure . In the case of the <HNF/Iso> phase, there was no change after UV irradiation. In contrast, in <HNF/N> and <HNF/SmA>, distinct changes were detected after UV irradiation; the CD intensities detected at λ = 300 nm were lowered to that of <HNF/Iso> as a result of UV irradiation. These results revealed that the LC phases (N or SmA) of the PR mesogens embedded in the BC molecular HNFs were transformed into the Iso phase upon UV irradiation.
Figure 4. Typical CD profiles at the three different nanosegregated phases (<HNF/Iso>, <HNF/N>, and <HNF/SmA>).
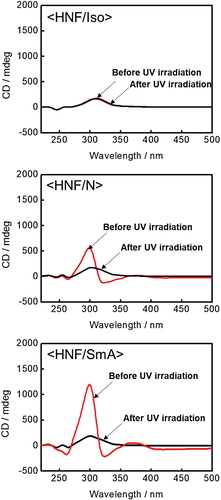
The aforementioned decreased CD intensities in <HNF/N> and <HNF/SmA> could be reverted to the initial CD intensities upon irradiation with visible light (450 nm; 30 mW cm−2). This is because the Iso phase of the PR mesogens induced by the trans–cis photoisomerization process reverted to the original phase (N or SmA) as the activated cis isomers reverted to the trans isomers upon irradiation with visible light. A probable explanation of the phenomenon that occurred after UV irradiation at <HNF/SmA> is shown in Figure . The absolute CD intensities were collected at λ = 300 nm for <HNF/N> and <HNF/SmA> in the positive domains as a function of alternating irradiation with UV and visible light (Figure ). The first data point for the initial <HNF/N> or <HNF/SmA> was checked, and then the subsequent points were monitored after alternating UV and visible-light irradiation for 2 s. The absolute CD intensities could be reversibly modulated by alternating irradiation with UV and visible light in both the <HNF/N> and <HNF/SmA> phases. To the best of the authors’ knowledge, a repeatable change in the absolute CD intensity was realized for several hours. As shown in Figure , the CD intensity ratio before and after UV irradiation in the case of <HNF/SmA> was much larger than that for the <HNF/N> phase.
4. Summary
In summary, a mixture of nonchiral BC and PR rod-like mesogens was prepared in this study. In this mixture system, both nanosegregated <HNF/N> and <HNF/SmA> phases were observed. The CD intensities depended strongly on the thermotropic polymorphism of the PR mesogen embedded in the BC molecular HNFs; the largest CD intensity was detected in <HNF/SmA>. The CD intensities could be reversibly modulated by alternating irradiation with UV and visible light in both the <HNF/N> and <HNF/SmA> phases. As the CD intensity ratio before and after UV irradiation at <HNF/SmA> was much larger than that at <HNF/N>, it is likely that the <HNF/SmA> phase is more promising than the <HNF/N> phase as a reversible photomodulated chiroptical material for rewritable optical storage.
Acknowledgements
The authors thank Dr. Fumito Araoka in RIKEN, Japan, for CD measurements.
Disclosure statement
No potential conflict of interest was reported by the authors.
Additional information
Funding
Notes on contributors
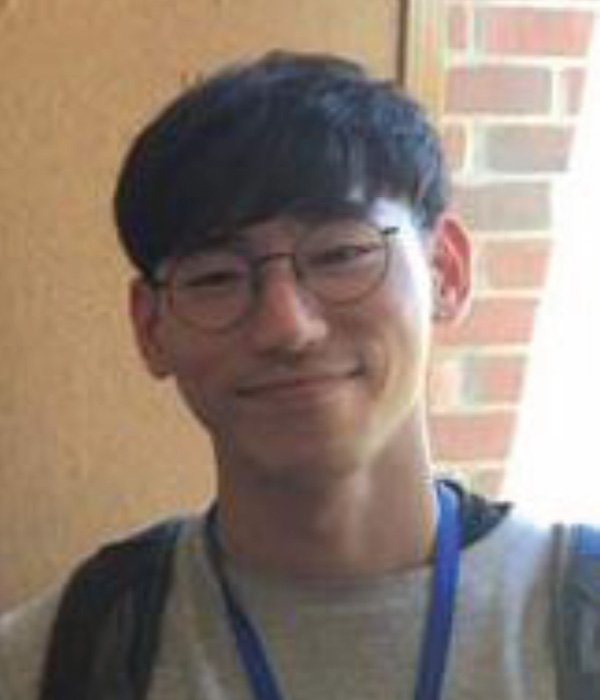
Sung-Wook Jeon
Sung-Wook Jeon obtained his B.S. and M.S. degrees from the Department of Advanced Material Engineering for Information & Electronics of Kyung Hee University, South Korea in 2016 and 2018, respectively. He has been working at LG Chem Ltd. since spring 2018.
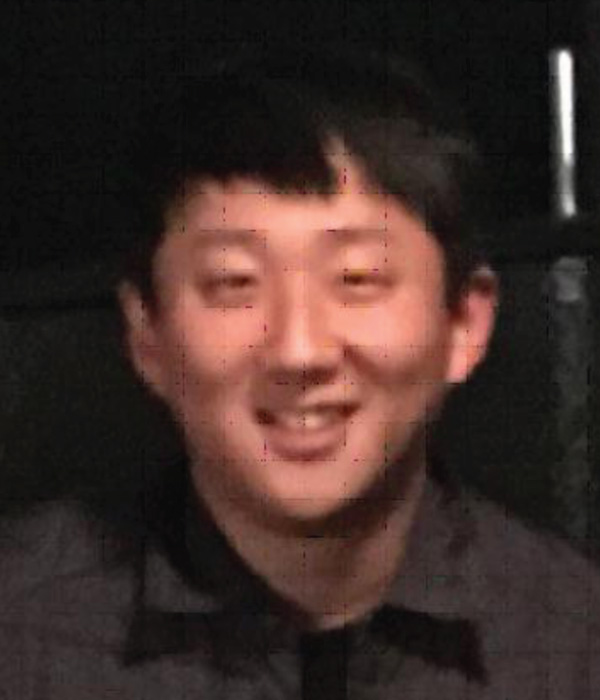
Hyeon-Joon Choi
Hyeon-Joon Choi obtained his B.S. degree from the Department of Advanced Material Engineering for Information & Electronics of Kyung Hee University, South Korea in 2018, and is now an M.S. candidate in the same university.
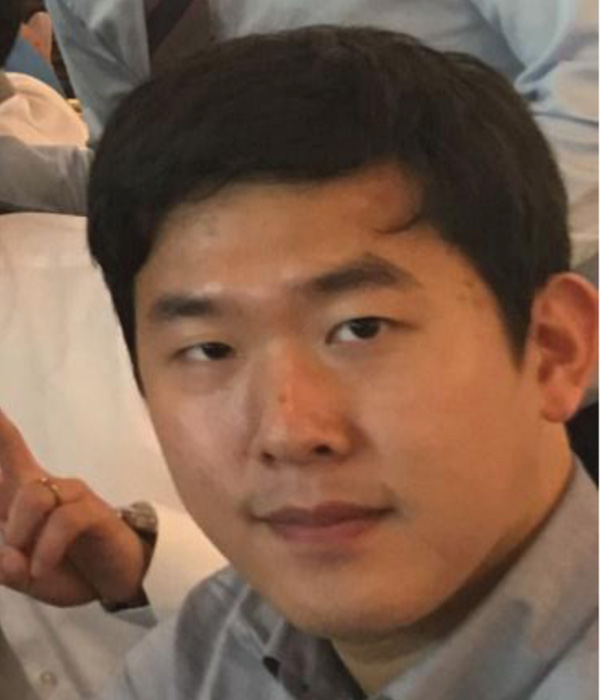
Jae-Hyun Bae
Jae-Hyun Bae obtained his B.S. degree from the Department of Advanced Material Engineering for Information & Electronics of Kyung Hee University, South Korea in 2015, and is now an M.S. candidate in the same university. He has been researching on photonic crystals related to liquid crystalline materials.
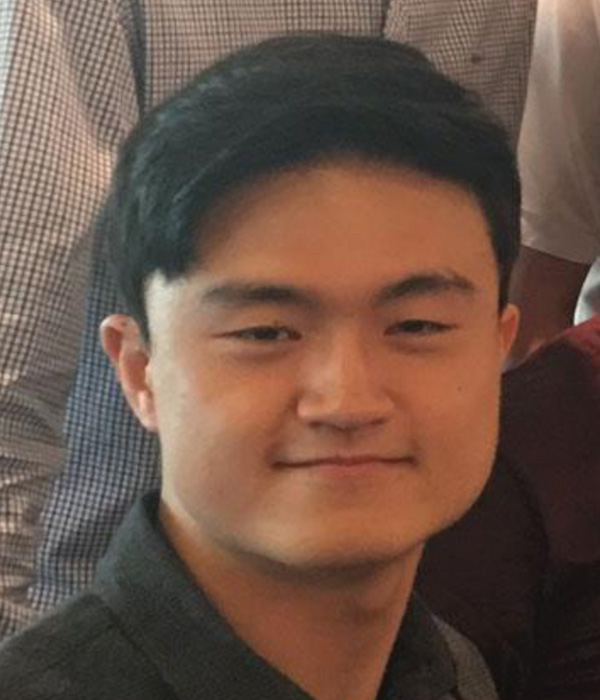
Byeong-Cheon Kim
Byeong-Cheon Kim obtained his B.S. degree from the Department of Advanced Material Engineering for Information & Electronics of Kyung Hee University, South Korea in 2017, and is now an M.S. candidate in the same university. He has been researching on liquid crystalline materials related to chirality.
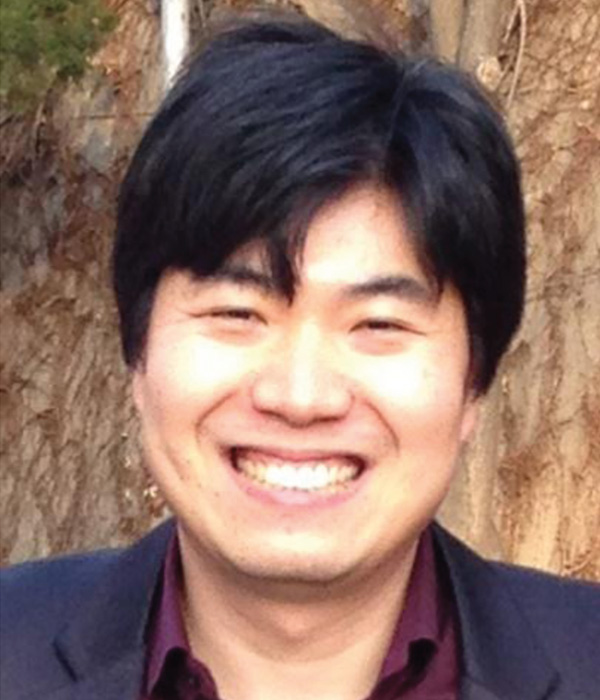
Suk-Won Choi
Suk-Won Choi is a tenured professor in the Department of Advanced Material Engineering for Information & Electronics of Kyung Hee University, South Korea. He obtained his B.S. degree from Hanyang University in South Korea, and his M.S. and Ph.D. degrees from Tokyo Institute of Technology in Japan. Since joining the faculty of Kyung Hee University in 2008, he has been researching on the liquid crystalline materials for optics and photonics applications.
References
- H. Takezoe and Y. Takanishi, Jpn. J. Appl. Phys. 45, 597 (2006). doi: 10.1143/JJAP.45.597
- R.A. Reddy and C. Tschierske, J. Mater. Chem. 16, 907 (2006). doi: 10.1039/B504400F
- S.-Y. Jo, B.-C. Kim, S.-W. Jeon, J.-H. Bae, M. Walker, M. Wilson, S.-W. Choi and H. Takezoe, RSC Adv. 7, 1932 (2017). doi: 10.1039/C6RA27158H
- B.-C. Kim, M. Walker, S.-Y. Jo, M. Wilson, H. Takezoe and S.-W. Choi, RSC Adv. 8, 1292 (2018). doi: 10.1039/C7RA11589J
- K.V. Le, H. Takezoe and F. Araoka, Adv. Mater. 29, 1602737 (2017); L.E. Hough, H.T. Jung, D. Krüerke, M.S. Heberling, M. Nakata, C.D. Jones, D. Chen, D.R. Link, J. Zasadzinski, G. Heppke, J.P. Rabe, W. Stocker, E. Körblova, D.M. Walba, M.A. Glaser and N.A. Clark, Science 325, 456 (2009). doi: 10.1002/adma.201602737
- F. Araoka, G. Sugiyama, K. Ishikawa and H. Takezoe, Adv. Funct. Mater. 23, 2701 (2013); H. Kim, S.H. Ryu, M. Tuchband, T.J. Shin, E. Korblova, D.M. Walba, N.A. Clark and D.K. Yoon, Sci. Adv. 3, e1602102 (2017). doi: 10.1002/adfm.201201889
- D. Chen, J.E. Maclennan, R. Shao, D.K. Yoon, H. Wang, E. Korblova, D.M. Walba, M.A. Glaser and N.A. Clark, J. Am. Chem. Soc. 133, 12656 (2011); D. Chen, M.R. Tuchband, B. Horanyi, E. Korblova, D.M. Walba, M.A. Glaser, J.E. Maclennan and N.A. Clark, Nat. Commun. 6, 7763 (2015). doi: 10.1021/ja203522x
- K. Kim, S. Kim, S.-Y. Jo and S.-W. Choi, J. Inf. Disp. 16,155 (2015). doi: 10.1080/15980316.2015.1062809
- S.-Y. Jo, S.-W. Jeon, B.-C. Kim, H.-H. Bae, F. Araoka and S.-W. Choi, ACS Appl. Mater. Interfaces 9, 8941 (2017). doi: 10.1021/acsami.7b01502
- J.-H. Bae, B.-C. Kim, S.-J. Jo and S.-W. Choi, J. Inf. Disp. 18, 191 (2017). doi: 10.1080/15980316.2017.1384408
- Y. Takanishi, G.J. Shin, J.C. Jung, S.-W. Choi, K. Ishiwawa, J. Watanabe, H. Takezoe and P. Toledano, J. Mater. Chem. 15, 4020 (2005). doi: 10.1039/b507875j
- T. Otani, F. Araoka, K. Ishikawa and H. Takezoe, J. Am. Chem. Soc. 131, 12368 (2009). doi: 10.1021/ja904447b
- K. Kim, H. Kim, S.-Y. Jo, F. Araoka, D.K. Yoon and S.-W. Choi, ACS Appl. Mater. Interfaces 7, 22686 (2015). doi: 10.1021/acsami.5b07543
- S.-W. Jeon, D.-Y. Kim, F. Araoka, K.-U. Jeong and S.-W. Choi, Chem. Eur. J. 23, 17794 (2017). doi: 10.1002/chem.201703778
- T. Ikeda, J. Mater. Chem. 13, 2037 (2003); S.H. Ryu, M.-J. Gim, W. Lee, S.-W. Choi and D.K. Yoon, ACS Appl. Mater. Interfaces 9, 3186 (2017). doi: 10.1039/b306216n