Abstract
Interannual and long-term variability of the low level jetstream (LLJ) of the Asian summer monsoon during June to September was studied using reanalysis wind data. In order to study the variability, the domain of the LLJ covering the Indian and west Pacific Oceans was divided into six regions (boxes) based on their physical characteristics. The long term variability of LLJ and its relation with Indian monsoon rainfall and El Nino index were analysed. The low level circulation over Indian Ocean becomes weak during the positive phase of El Nino Southern Oscillation leads to warmer sea surface temperature over Indian Ocean. The strength of the low level jet over India depends on the convective heating of the atmosphere and not on the strength of the south Indian Ocean trade wind (ITz) which remains a passive component of the LLJ. The LLJ flow through peninsular India is weaker and the flow south of peninsular India is stronger during the recent decades in association with increasing number of break monsoon days. The cross equatorial flow over west Pacific Ocean has statistically significant increasing trend related to the increasing convection in the west Pacific Ocean.
1. Introduction
The economy of India depends heavily on agriculture and is strongly influenced by the Indian Summer Monsoon Rainfall (ISMR) during June to September which accounts for more than 70% of the annual rainfall of India. There is considerable variation in monsoon rainfall of India from year to year. Both internal and external factors control this inter-annual variability. El Nino Southern Oscillation (ENSO) is one of the external forcings on ISMR and active break cycle of the monsoon is one of the prominent internal forcings (Goswami, Citation2005)
The low level jetstream (LLJ) is one of the important semi-permanent components of the Asian summer monsoon and is a part of the monsoon Hadley circulation (Krishnamurti and Bhalme, Citation1976). The existence of LLJ over peninsular India during the monsoon season with strong wind shears both in the vertical and horizontal was established by Joseph and Raman (Citation1966). Findlater (Citation1969a) showed that this LLJ is cross-equatorial and had its origin in the easterly trade winds of the south Indian Ocean. LLJ winds of south Indian Ocean crossed the equator in a narrow longitudinal belt close to the east African coast as a southerly jetstream, with wind speeds at times as high as 50 ms−1. LLJ turned westerly over the Arabian Sea, and passed through peninsular India and the Bay of Bengal. Findlater (Citation1969b) showed that this LLJ accounted for nearly half of the global inter-hemispheric transport of air in the lower troposphere during the monsoon season June to September.
Figure a gives the wind at 850 hPa over south Asia of June to September averaged over the years 1979–2010 using JRA-55 reanalysis data which shows the mean inter-hemispheric LLJ of the Asian summer monsoon. Figure b shows the June to September averaged outgoing longwave radiation (OLR) of the period 1979–2010. It is taken as a proxy for deep convection and the associated rainfall. The moisture generated over the south Indian Ocean and the evaporative flux from the Arabian Sea are carried by the strong cross-equatorial air flow near the east African coast and the zonal flow across peninsular India into the convective heat source area A in Fig. b. The convective area B, (Fig. b) which is over the west Pacific Ocean between latitudes 5°N and 20°N east of longitude 120oE, is associated with the moisture transport through the cross-equatorial flow east of longitude 100°E and also through the zonal flow through peninsular India extending eastwards to the west Pacific Ocean.
Fig. 1. (a) Mean 850 hPa wind of monsoon season (JJAS) for the period 1979 to 2010 showing the zonal flows and the cross equatorial meridional flows. The six representative regions (as marked by boxes) of the LLJ of Asian summer monsoon are (1) Equatorial Indian-Ocean EIm (box 5°S–5°N, 35°E–55°E), (2) Central Arabian-sea CAz (box 10°N–20°N, 55°E–65°E), (3) Peninsular India PIz (box 10°N–20°N, 70°E–85°E), (4) west Pacific-Ocean WPm (box 5°S–5°N, 100°E–140°E), (5) northwest Pacific (box 5°N–15°N, 120°E–160°E) and (6) Indian-Ocean Trades ITz (box 20°S–5°S, 60°E–90°E).Suffixes z and m represent zonal and meridional components of wind (b) Mean OLR (Watts per sq, metre) of the monsoon season (JJAS) for the period 1979–2010. The two active convection zones of the monsoon season A and B are marked.
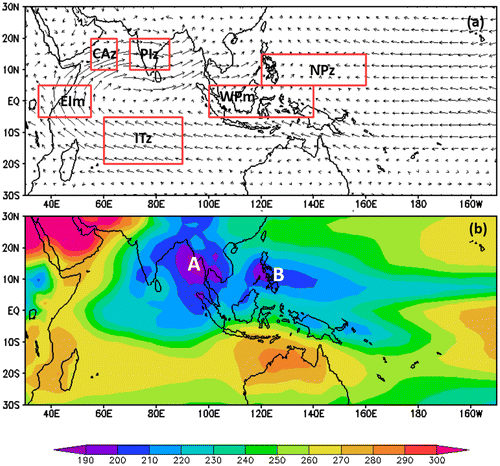
A time-dependent primitive equation model with specified zonal flow, mountains and adiabatic heating was used to study the LLJ by Hoskins and Rodwell (Citation1995) and Rodwell and Hoskins (Citation1995). Their study identified the strong sensitivity of the LLJ to changes in convective heating over the Indian Ocean. Joseph and Sijikumar (Citation2004) found that the strength of the convective heating over the Bay of Bengal and the strength of the LLJ (zonal wind component at 850 hPa) through peninsular India have the highest correlation with a lag of 2–3 days, convective heating leading. A similar relation was found for the west Pacific Ocean by Joseph and Sabin (Citation2008). In response to the increased land-sea thermal contrast due to global warming, the LLJ is found to shift poleward (Sandeep and Ajayamohan, Citation2014). Krishnamurti et al. (Citation1976) used a one-level primitive equation model and highlighted the importance of the east African Mountains, land-sea thermal contrast and beta effect on the simulation of the LLJ.
However no comprehensive study of the interannual variability of the LLJ and its relation with sea surface temperature (SST) over east Pacific (Nino 3.4) has so far been done which is the objective of our study. We have used data sets of the last five decades to study the variation of zonal/meridional flow of the LLJ associated with the boreal summer monsoon circulation over the tropical Indian Ocean and west Pacific Ocean. In addition to that the relation between LLJ and ISMR and that between LLJ and El Nino were studied. Section 2 describes the data used for the study. Inter-annual variability of LLJ and its relation to ISMR and El Nino are discussed in Section 3. Study of the long term variability of LLJ is presented in Section 4. Discussion and conclusions of the study are given in Sections 5 and 6 respectively
2. Data and methodology
ISMR is the rainfall of India from 1 June to 30 September derived by the method described in Parthasarathy et al. (Citation1994) as average of 306 climatic rain gauge stations well distributed over India (location of these rain gauge stations are shown in Figure 1 of Parthasarathy et al., Citation1994). This data updated every year is available in the website of the Indian Institute of Tropical Meteorology (IITM), Pune (www.tropmet.res.in). The standard deviation of ISMR is about its 10%.
Wind data of 850 hPa are from Japanese Reanalysis (JRA-55) data-set at 1.25° × 1.25° latitude/longitude horizontal resolution for 1958–2010 (Kobayashi et al. Citation2015) and National Centres for Environmental Prediction/ National Centre for Atmospheric Research (NCEP/NCAR) reanalysis (Kalnay et al., Citation1996) with horizontal resolution of 2.5° × 2.5° latitude/longitude for period of 1950–2010. National Oceanic and Atmospheric Administration (NOAA) interpolated OLR data (Liebmann and Smith, Citation1996) of resolution 2.5° × 2.5° are used as proxy for the convective heating of the atmosphere. Monthly SST are from the HadISST data-set as derived by Rayner et al. (Citation2003) having 1° × 1° latitude/longitude spatial resolution. Mean SST anomalies in the Nino 3.4 (5°S–5°N, 120°W–170°W) region of the season June to September and October to December (hereafter called N3.4 index) are used. The N3.4 index is taken from http://www.esrl.noaa.gov/psd/gcos_wgsp/Timeseries/Nino34/.
Most of the research work on LLJ reported in the literature considered the whole LLJ as one entity. Using a general circulation model, Chakraborty et al. (Citation2009) studied the influence of the east African highlands and the convective heating of the atmosphere over the Bay of Bengal on the LLJ of the Indian Ocean by dividing it into four boxes. In this study, the domain of LLJ covering a large area (Indian and west Pacific Oceans) has been divided into six zones represented by Equatorial Indian-Ocean box (5°S–5°N, 35°E–55°E), Central Arabian-sea box (10°N–20°N, 55°E–65°E), Peninsular India box (10°N–20°N, 70°E–85°E), west Pacific-Ocean box (5°S–5°N, 100°E–140°E), northwest Pacific box (5°N–15°N, 120°E–160°E) and Indian-Ocean Trades box (20°S–5°S, 60°E–90°E) abbreviated as EIm, CAz, PIz, WPm, NPz and ITz respectively as shown in Fig. . Suffixes z and m represent zonal and meridional wind components respectively. Winds of boxes EIm and WPm are predominantly meridional and winds in the other 4 boxes CAz, PIz, NPz and ITz are zonal.
The LLJ winds over Indian Ocean is divided into 4 boxes, more or less similar to the four boxes of LLJ in Chakraborty et al. (Citation2009) and the wind over west Pacific is divided into 2 boxes. The physical reason for dividing the LLJ into different region are: the LLJ originate from the easterly trade wind of the south Indian Ocean (Findlater, Citation1969a) is represented by the box ITz and is a region of generation of large amounts of moisture by evaporation at the sea surface by the strong trade winds (south easterly) of the monsoon season. Most of the inter-hemispheric transport of moisture generated in the south Indian Ocean is through the box EIm which has southerly winds. The core of the LLJ in the north Indian Ocean is found in the Arabian Sea represented by the box CAz where the wind is southwesterly. In active monsoon periods, the strong westerly wind flows through India represented by the box PIz whereas in break or weak monsoon spells the wind flow through this box become weak (Joseph and Sijikumar, Citation2004). Even though the wind over west Pacific is mostly the easterly trade winds, the westerly winds of LLJ extend eastwards beyond 120°E into box NPz during August and Septmber in the climatic mean and in all four monsoon months in El Nino years. The cross equatorial flow over the box WPm also strengthens the zonal flow over NPz during this period. So we consider these two boxes over west Pacific (WPm and NPz) to study the variability of the wind over these regions during monsoon season.
3. Results
3.1 Inter-annual relationships among components of LLJ and ISMR/N3.4
The inter-annual variability of low level wind (850 hPa zonal or meridional mean wind of the monsoon season June to September) of the six boxes covering the domain of the LLJ, four in Indian Ocean region (EIm, CAz, PIz and ITz) and two in west Pacific Ocean (WPm and NPz) are studied. From the time series of zonal/meridional wind over each box, an 11-year moving average is removed to get a time series free from decadal variability and trend for the study of inter annual variability. The Pearson’s (linear) Correlation Coefficient (PCC hereafter called correlation) between ISMR/Nino 3.4 and zonal/meridional wind over each of the six boxes are shown in Table and correlation between winds over a pair of boxes are shown in Table . We have taken negative value for the south easterly trade wind over the box ITz in order to get the same sign for the correlation as for the other boxes. We have used wind data of JRA-55 (1958–2010) for this study as it has higher spatial resolution than NCEP/NCAR reanalysis data-set. For the verification we have used NCEP/NCAR reanalysis (1950–2010) data-set. Statistically significant correlation coefficients (using a Student’s t-test) at the 99.9% confidence level are marked in red colour, at 99% in green and at 95% in blue.
Table 1a. Correlation between interannual components of pairs of LLJ box parameters and ISMR / N3.4 Red, green and blue colours represents 99.9, 99 and 95% levels of statistical significance.
Table 1b. The PCC in the inter-annual component among six parameters of LLJ using JRA55 and NCEP (marked top left) data. Red, green and blue colours represents 99.9, 99 and 95% levels of statistical significance.
The correlation between ISMR and monsoon season wind over each box indicates that low level flow over the Indian Ocean are positively and significantly correlated with monsoon rainfall and that of west Pacific Ocean are negatively correlated. The last four columns in Table represents the relationship between the SST anomaly over Nino 3.4 region (mean of months OND and JJAS) and zonal/meridional wind over each box of the months JJAS. The cross equatorial flow (EIm) and south westerly flow (CAz) over Indian Ocean are negatively and significantly related to Nino 3.4 index whereas the wind over west Pacific Ocean (WPm and NPz) are positively related with high correlation that are statistically significant at 99.9%. The 51-yr (43-yr) correlations between wind over most pairs of boxes have strong correlation coefficients (Table ). For the 51-yr (43-yr) period, a correlation larger than 0.44 (0.49) is significant at the 99.9% confidence level (marked in red color). The correlation between 0.35 and 0.44 (0.39 and 0.49) is significant between 99% and 99.9% (green) and the correlation between 0.27 and 0.35 (0.30 and 0.39) is between 95% and 99% (blue) confidence level.
The correlation between N3.4 on one side and wind over Pacific Ocean (NPz and WPm) on the other are very high at 0.78. Increase in N3.4 (stronger El Nino) increases both cross equatorial flow (WPm) and zonal flow (NPz) over west pacific. Anomalous westerlies in the north west Pacific (NPz) creates increased cyclonic vorticity in the boundary layer causing increased convection (rainfall) in box-NPz. Convection in box-NPz on one side and zonal winds in box NPz and meridional winds in the box WPm on the other grow in a positive feedback. It is well known that El Nino is associated with deficient ISMR. Nino 3.4 index and ISMR have a correlation of −0.51. The correlation between N3.4 and cross equatorial flow over west Indian Ocean (EIm) is negative and very high at −0.62. This shows that when N3.4 is high, the meridional wind in EIm becomes weak and when this wind is weak, the along-shore winds along the coasts of Somalia and Arabia become weak reducing the coastal upwelling there generating warm SST anomalies in western Indian Ocean. Weaker winds in this area are also associated with weaker ISMR. Here lies the explanation for the findings of Babu and Joseph (Citation2002) that monsoon droughts accompanied by El Nino creates stronger warm SST anomalies over north Indian Ocean than drought monsoon not accompanied by El Ninos. The cross equatorial flow over India (EIm) and cross equatorial flow over west Pacific (WPm) are negatively correlated with a correlation of −0.45. The meridional flow WPm (EIm) strengthens (weakens) when N3.4 is high.
An important finding is that the LLJ component south of the equator in the Indian Ocean (ITz) has very low correlation with ISMR, N3.4 and the wind components EIm, CAz, WPm and NPz on the interannual time scale. There is a general concept that the trade winds in the ITz region cross the equator (EIm) and turn right to become the monsoon flow (CAz and PIz). The correlation between ITz on one side and EIm, CAz and PIz on the other side should have been high which is not the case. There is a high correlation between convective heating of the atmosphere and the LLJ components CAz and PIz in the Indian Ocean and NPz in the west Pacific Ocean at a lag of 2–3 days (convection leading) in positive feedbacks as described in Chakraborty et al. (Citation2002), Joseph and Sijikumar (Citation2004) and Joseph and Sabin (Citation2008). Strength of the LLJ zonal wind components CAz and PIz depend on the convective heating of the atmosphere and not on the strength of the trade winds in south Indian Ocean (ITz) which remains a passive component of the LLJ on the interannual time scale. The surface wind in box ITz is however important in the generation of moisture that is conveyed through the LLJ for the generation of monsoon rain in area A of Fig. b.
3.2 Long term variability of LLJ
We have carried out trend analysis of 850 hPa zonal wind and meridional wind for the monsoon seasons of the period 1958–2010 using JRA-55 reanalysis data (Fig. a and b respectively) and for the period 1950–2010 using NCEP/NCAR reanalysis data (figures not shown). These data sets have used actual measured wind data at several levels in the atmosphere for the reanalysis and therefore are highly reliable. The westerlies in the north Indian Ocean between 10°N and 20°N has shown a decreasing trend while that between equator and 5°N shows increasing trend. The increasing trend of northerlies in the west coast of India and westerlies between equator and 5°N implies that the LLJ bypasses India more frequently during the recent decades. This is linked to the increasing frequency of break monsoon days of the monsoon season in the recent decades (Joseph and Simon, Citation2005; Rameshkumar et al., Citation2009) as the LLJ axis shift towards the equator east of longitude 70°E during break monsoon spells (Joseph and Sijikumar, Citation2004). Significant strengthening of easterlies is found in the south Indian Ocean south of 15°S. The meridional wind WPm shows statistically significant increasing trend. Satyaban et al. (Citation2016) found that there is increasing trend of moisture over west Pacific Ocean during this period. The increasing trend of the meridional wind WPm may be in response to the increasing convection in the west Pacific Ocean (see Fig. c which supports this) caused by the increased moisture.
4. Discussion
The variability of the low level wind over the tropical Indian Ocean and the west Pacific Ocean during the boreal summer monsoon season has been studied using 850 hPa reanalysis wind data of JRA-55 and NCEP. The domain of the LLJ over Indian Ocean was divided into four boxes and that over west Pacific into two boxes. The interannual variability of zonal/meridional wind over these boxes and their trend analysis was carried out. The relationship between zonal/meridional wind over these boxes and ISMR/El Nino has also been studied. A schematic diagram showing the prominent correlations among the parameters studied in their inter-annual components using JRA-55 wind data is shown in Fig. . On the inter-annual time scale the relation between ISMR and the low level wind over Indian Ocean (EIm, CAz and PIz) may be taken as two way interactions and the correlations are very high. The magnitude of westerly LLJ over the Arabian Sea is directly linked to the strength of the monsoonal heat source (Chakraborty et al., Citation2009) The convective heating of the atmosphere results in the strengthening of the LLJ winds. Stronger LLJ winds are associated with higher cyclonic vorticity in the atmospheric boundary layer leading to increased convection (rainfall). There is a positive feedback between convection and wind on the intra-seasonal time scale as discussed in Joseph and Sijikumar (Citation2004) and Joseph and Sabin (Citation2008). Strength of the LLJ zonal wind components CAz, PIz and NPz depend on the convective heating of the atmosphere and not on the strength of the trade winds of south Indian Ocean ITz, which remains a passive component of the LLJ on the interannual time scale.
Fig. 3. Schematic diagram representing inter-annual correlations among ISMR, Nino 3.4 and LLJ components (positive PCC by red arrows and negative PCC by green). All the PCCs marked are at 99.9% level of statistical significance. A one way relation that is statistically significant is marked by an arrow with single head. If there is a high and significant PCC between two parameters with two way interaction, it is represented by lines with arrows on both ends.
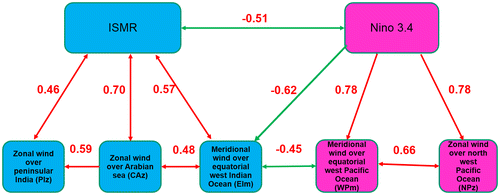
The cross equatorial flow over west Indian Ocean (EIm) weakened (strengthened), while cross equatorial flow over west Pacific Ocean (WPm) strengthened (weakened) during a warm (cold) phase of ENSO. Zhu (Citation2012) has also found similar relationship. The cross equatorial flow, EIm and WPm are connected to the strength of two highs, Mascerene High and Australian High respectively as shown by Wang and Li (Citation1982) and Xue et al. (Citation2004). Australian High tends to be intensified with the occurrence of El Nino (Xue et al., Citation2004). Convective heating over the central Pacific and the intensified Australian High during El Nino creates the meridional pressure gradient that accelerates the cross equatorial flow over west Pacific, WPm. The strengthened WPm tends to increase evaporation over the tropical west Pacific and maritime continent accounting for the cooling of SST therein (Fig. a). This leads to anomalous westerlies near the surface by the weakening of trade winds (Fig. b). Zhu (Citation2012) found that the strength of the cross-equatorial flow near the Somali coast is negatively related to the SST over western tropical Indian Ocean and Arabian Sea. When the along-shore winds off the coasts of Somalia and Arabia become weak the coastal upwelling reduces generating warm SST anomalies there. This may be the reason for the post monsoon SST anomalies in north Indian Ocean become much higher in monsoon drought years accompanied by El Nino as compared to those not accompanied. Lin et al., (Citation2014) found that strong cross-equatorial flow and westerlies are associated with the presence of monsoon trough over west Pacific during west north Pacific summer monsoon. Most of the strong monsoon trough years are taken based on the vorticity over 5°N–20°N, 130°E–175°E during Aug–Sep are the El Nino years.
Fig. 4. (a) Mean JJAS SST anomaly of El Nino years during 1958–2010 over west Pacific. (b) same as (a) but for 850 hPa wind anomaly (JRA-55). Years with N3.4 index equal to and greater than 1 of the season October to December is taken as El Nino years (1965, 1972, 1977, 1982, 1986, 1987, 1991, 1994, 1997, 2002, 2006, 2009).
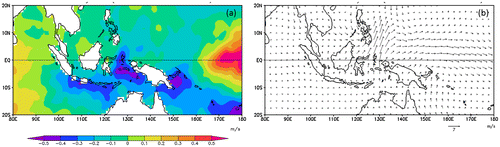
The strength of the monsoon depends on the moisture transport through peninsular India and the intensity of wind over CAz/PIz. Both CAz and PIz has decreasing trend and are related to the convective heating of the atmosphere. Joseph (Citation2014) has shown that during the period studied in this paper, the frequency of monsoon depression, forming in north Bay of Bengal had a steep decreasing trend. The LLJ through peninsular India (PIz) has a control on the frequency of monsoon depressions as it is related to the low level cyclonic relative vorticity over north Bay of Bengal situated just north of the LLJ axis when monsoon is in the active phase. The strength of the monsoon flow are not favouable for the intensification of lows into depressions and cyclones during the last two decades (Dash et al., Citation2004). The decreasing trend of PIz and the increasing trend of northerlies in the west coast of India and westerlies between equator and 5°N results in the increasing trend of break monsoon days during the monsoon season as found by Joseph and Simon (Citation2005) and Rameshkumar et al. (Citation2009) and the axis of LLJ shifts equatorwards east of longitude 70°E during break monsoon spells (Joseph and Sijikumar, Citation2004). Most of the wind that carries moisture from the south Indian Ocean (SIO) and Arabian Sea bypasses India causing less precipitation over Indian subcontinent (Sathyaban et al. Citation2016). Also Zhao et al. (Citation2012) found that Asian monsoon circulation strengthened during the positive phase of Asia Pacific Oscillation and it has negative trend in recent decades. As a result CAz and PIz shows decreasing trend. Increasing trend of convection over northwest Pacific and the increased inter-hemispheric moisture transport as found by Sathyaban et al. (Citation2016) results in the increasing trend of cross equatorial flow over west Pacific (WPm).
5. Conclusions
The domain of the LLJ covering the Indian and west Pacific Oceans was divided into six representative zones (boxes) with different physical charateristics and the linear correlation between the mean monsoon winds (zonal or meridional) in these boxes and Indian Summer Monsoon Rainfall/El Nino Index at the inter-annual time scale were studied. The climate change during the last five decades is also studied by analysing the linear trends of 850 hPa wind.
On interannual timescale, the strength of the low level jet over India depends on the convective heating of the atmosphere and not on the strength of the south Indian Ocean trade wind (ITz) which remains a passive component of the LLJ. The strength of the El Nino (N3.4) has negatively correlated with cross equatorial flow over the western Indian Ocean (EIm) and it explains why El Nino is associated with large and positive SST anomaly in tropical western Indian Ocean.
Monsoon mean zonal wind through Peninsular India has a statistically significant decreasing linear trend. It is related to the decreasing trend in the frequency of monsoon depressions and increasing number of break monsoon days observed. The cross equatorial flow over west Pacific Ocean WPm has significant increasing trend related to the increasing convection in the west Pacific Ocean.
Disclosure statement
No potential conflict of interest was reported by the authors.
Funding
This work was supported by the Research Council of Norway [grant number 216554].
Acknowledgements
The authors acknowledge the support of the Research Council of Norway through NORKLIMA-INDNOR program (contract #216554) lead by Ola M Johannessen and P. V. Joseph. One of the authors, Ms. Shinu Sheela Wilson is thankful to Nansen Scientific Society, Bergen, Norway for providing her a Nansen Fellowship for her Ph.D and to the Nansen Center in Bergen for research periods there including supervision. We thank the NOAA Earth System Research Laboratory, Boulder, Colorado, USA for providing the Interpolated OLR data from their Web site at http://www.esrl.noaa.gov/psd/. We also thank Japanese Meteorological Agency (JMA) for providing JRA-55 reanalysis dataset.
References
- Babu, C. A. and Joseph, P. V. 2002. Post monsoon sea surface temperature and convection anomalies over Indian and Pacific Oceans. International Journal of Climatology 22, 559–567.10.1002/(ISSN)1097-0088
- Chakraborty, A., Nanjundiah, R. S. and Srinivasan, J. 2002. Role of Asian and African orography on Indian summer monsoon. Geophysical Research Letters 29(20), 1989 50-1–50-4. DOI:10.1029/2002GL015522.
- Chakraborty, A., Nanjundiah, R. S. and Srinivasan, J. 2009. Impact of African orography and the Indian summer monsoon on the low-level Somali jet. Int. J. Climatol 29, 983–992.10.1002/joc.v29:7
- Dash, S. K., Janamani, R. K. and Shekhar, M. S. 2004. On the decreasing frequency of monsoon depressions over the Indian region. Curr Sci 86, 1404–1411.
- Findlater, J. 1969a. A major low-level air current near the Indian Ocean during the northern summer. Quart J Roy Meteor Soc 95, 362–380.10.1002/(ISSN)1477-870X
- Findlater, J. 1969b. Interhemispheric transport of air in the lower troposphere over the western Indian Ocean. Quart J Roy Meteor Soc 95, 400–403.10.1002/(ISSN)1477-870X
- Goswami, B. N. 2005. South Asian monsoon. In: Intra-seasonal variability in the atmosphere–ocean climate system (eds. W. K. M. Lau and D. E. Waliser). Springer, United Kingdom, pp. 19–61.10.1007/b138817
- Hoskins, B. J. and Rodwell, M. J. 1995. A model of the Asian Summer Monsoon. Part I: The global scale. J Atmos Sci 52, 1329–1340.10.1175/1520-0469(1995)052<1329:AMOTAS>2.0.CO;2
- Joseph, P. V. 2014. Role of ocean in the variability of Indian Summer Monsoon Rainfall. Surveys Geophys 35, 723–738.10.1007/s10712-013-9274-7
- Joseph, P. V. and Simon, Anu 2005. Weakening trend of the southwest monsoon current through peninsular India from 1980 to the present. Curr Sci 89, 687–694.
- Joseph, P. V. and Raman, P. L. 1966. Existence of low level westerly jetstream over peninsular India during July. Indian J Meteor Geophys 17, 407–410.
- Joseph, P. V. and Sabin, T. P. 2008. An ocean–atmosphere interaction mechanism for the active break cycle of the Asian summer monsoon. Clim Dyn 30, 553–566.10.1007/s00382-007-0305-2
- Joseph, P. V. and Sijikumar, S. 2004. Intraseasonal variability of the low-level jet stream of the Asian Summer Monsoon. J Clim 17, 1449–1458.10.1175/1520-0442(2004)017<1449:IVOTLJ>2.0.CO;2
- Kalnay, E.and Co-authors. 1996. The NCEP/NCAR 40-year reanalysis project. Bull Amer Meteor Soc 77, 437–471.10.1175/1520-0477(1996)077<0437:TNYRP>2.0.CO;2
- Kobayashi, S. and Coauthors. 2015. The JRA-55 reanalysis: general specifications and basic characteristics. J Met Soc Jap 93, 5–48.10.2151/jmsj.2015-001
- Krishnamurti, T. N. and Bhalme, H. N. 1976. Oscillations of a monsoon system. Part I. Observational aspects. J. Atmos. Sci 33, 1937–1954.10.1175/1520-0469(1976)033<1937:OOAMSP>2.0.CO;2
- Krishnamurti, T. N., Molinari, J. and Pan, H. L. 1976. Numerical simulation of the Somali jet. J. Atmos. Sci 33, 2350–2362.10.1175/1520-0469(1976)033<2350:NSOTSJ>2.0.CO;2
- Liebmann, B. and Smith, C. A. 1996. Description of a complete (interpolated) OLR dataset. Bull Amer Meteor Soc 77, 1275–1277.
- Lin, Y.-W. LinHo and Chou, C., 2014. The role of the New Guinea cross-equatorial flow in the interannual variability of the western North Pacific summer monsoon. Environ. Res. Lett 9, 044003. DOI:10.1088/1748-9326/9/4/044003.
- Parthasarathy, B., Munot, A. A. and Kothawale, D. R. 1994. All India monthly and seasonal rainfall series 1871–1993. Theor Appl Climatol 49, 217–224.10.1007/BF00867461
- Ramesh Kumar, M. R., Krishnan, R., Sankar, S., Unnikrishnan, A. S. and Pai, D. S. 2009. Increasing trend of break monsoon conditions over India – role of Ocean-atmosphere processes in the Indian Ocean. IEEE Geosci Remote Sens Lett 6, 332–336.10.1109/LGRS.2009.2013366
- Rayner, N. A. and Coauthors., 2003. Global analyses of sea surface temperature, sea ice, and night marine air temperature since the late nineteenth century. J Geophys Res 108(D14), 4407. DOI:10.1029/2002JD002670.
- Rodwell, M. J. and Hoskins, B. J. 1995. A model of the Asian Summer Monsoon.Part II: cross-equatorial flow and PV behavior. J Atmos Sci 52, 1341–1356.10.1175/1520-0469(1995)052<1341:AMOTAS>2.0.CO;2
- Sandeep, S. and Ajayamohan, R. 2014. Poleward shift in Indian summer monsoon low level jetstream under global warming. Clim. Dyn. 45, 337–351.
- Satyaban, B. R., Cherchi, A., Joseph, P. V., Behera, S. K., Abish, B. and Masina, S. 2016. Moisture variability over the Indo-Pacific region and its influence on the Indian summer monsoon rainfall. Clim Dyn 46, 949–965.
- Wang, J. Z. and Li, M. C. 1982. Cross-equator flow from Australia and monsoon over China (in Chinese). Sci. Atmos. Sin. 6, 1–10.
- Xue, F., Wang, H. J. and He, J. H. 2004. Interannual variability of Mascarene high and Australian high and their influences on summer rainfall over East Asia. Chin. Sci. Bull. 48, 492–497. DOI:10.1007/BF03183258.
- Zhao, P., Wang, B. and Zhou, X. 2012. Boreal summer continental monsoon rainfall and hydroclimate anomalies associated with the Asian-Pacific Oscillation. Clim Dyn. 39, 1197–1207. DOI:10.1007/s00382-012-1348-6.
- Zhu, Y. L. 2012. Variations of the summer Somali and Australia cross-equatorial flows and the implications for the Asian summer monsoon. Adv. Atmos. Sci 29, 509–518. DOI:10.1007/s00376-011-1120-6.