ABSTRACT
Objective: Iron overload and oxidative stress are the major causes of serious complications and mortality in thalassemic patients. Our previous work supports the synergistic effects of antioxidant cocktails (curcuminoids or vitamin E, N-acetylcysteine, and deferiprone) in treatment of β-thalassemia/Hb E patients. This further 2-DE-based proteomic study aimed to identify the plasma proteins that expressed differentially in response to antioxidant cocktails.
Methods: Frozen plasma samples of ten normal subjects and ten β-thalassemia/Hb E patients at three-time points (baseline, month 6, and month 12) were reduced the dynamic range of proteome using ProteoMiner kit and separated proteins by two-dimensional gel electrophoresis. Differentially expressed proteins were identified using tandem mass spectrometry. Several plasma proteins were validated by ELISA and Western blot analysis.
Results: Thirteen and 11 proteins were identified with altered expression levels in the curcuminoids- and vitamin E cocktail groups, respectively. The associations between vitronectin (VTN) expression and total bilirubin levels, as well as between serum paraoxonase/arylesterase 1 (PON1) expression and blood reactive oxygen species were observed. Validation results were consistent with proteomics results.
Discussion and conclusions: These plasma proteins may provide better understanding of the mechanisms underlying the therapeutic effects of antioxidant cocktails in thalassemic patients.
Introduction
β-thalassemia/Hb E has the phenotypic diversity and high prevalence in Southeast Asia. It is responsible for up to 50% severe cases of β-thalassemia worldwide [Citation1]. In β-thalassemia, primary and secondary iron overload induce oxidative stress leading to several pathological consequences such as anemia, hypercoagulability, hepatosplenomegaly, tissue injuries, and organ dysfunctions [Citation2]. Therefore, antioxidants are required to alleviate or prevent such oxidative damages.
Previous studies revealed the therapeutic effects of antioxidants in thalassemic patients including curcuminoids [Citation3], N-acetylcysteine [Citation4], and vitamin E [Citation5]. In recent years, there is an increasing interest in using a combination of antioxidants to treat many diseases. However, only a few clinical studies were performed in thalassemia [Citation6–8]. Our previous study showed that one-year treatment with two antioxidant cocktails consisting of hydrophobic- (curcuminoids/vitamin E), hydrophilic antioxidant (N-acetylcysteine), and oral iron chelator (deferiprone) could improve anemia, decrease oxidative stress, and reverse hypercoagulability in β-thalassemia/Hb E patients [Citation7]. Although the beneficial effects of these antioxidant cocktails in thalassemic patients have been demonstrated, the underlying mechanisms of such effects still remain to be elucidated. Notably, proteomic approach has been widely used to explore biomolecular alteration associated with therapeutic effects of herbal extract in various diseases [Citation9–11].
Interestingly, two-dimensional fluorescence difference gel electrophoresis had been performed in albumin- and IgG-depleted plasma of β-thalassemia/Hb E patients treated with curcuminoids for 12 months [Citation12]. All proteins with differential expression in response to the treatment were coagulation proteins which are related to hypercoagulable state in these patients. However, the limitation of the aforementioned technique is insufficient removal of high-abundance proteins, hindering the detection of low-abundance plasma proteins. Therefore, in the present study, we apply low-abundance protein enrichment approach in order to reduce high-abundance proteins in plasma samples before proceeding with proteomic techniques to determine plasma proteome changes as well as proteins that are associated with improved clinico-biochemical conditions in thalassemic patients after antioxidant cocktails treatments.
Materials and methods
Plasma specimens
This study was approved by the Siriraj Institutional Review Board of Mahidol University, Thailand. Three time points (baseline, month 6, and month 12) of plasma from each 10 non-splenectomized, non-transfusion dependent β-thalassemia/Hb E patients treated with curcuminoids- (M/F = 5/5, age 35 ± 3 Y/O) or vitamin E antioxidant cocktail (M/F = 4/6, age 34 ± 3 Y/O) were obtained from our previous project [Citation7]. Plasma were derived from the patients who completed the treatment for 12 months, did not receive transfusion throughout the treatment, had improvements in pathological parameters, and signed informed consent form (). Plasma from 10 normal subjects was collected with the inclusion criteria as follows: (i) normal Hb typing (A2A); (ii) sex- and age-matched patients; (iii) no history of severe diseases; (iv) no alcohol consumption or smoking; and (v) no pregnancy or breastfeeding. All plasma specimens were stored at −80°C until used.
Table 1. Clinical data of the patients recruited in this study.
Sample preparation and two-dimensional gel electrophoresis
The ProteoMinerTM Protein Enrichment Kit (Bio-Rad Laboratories, Hercules, CA, USA) was used to reduce broad concentration range of plasma proteins. Depleted plasma was removed ionic contaminants using a 2-D Clean-Up Kit (GE Healthcare Biosciences Corp., Piscataway, NJ, USA). The pellet was resuspended in lysis buffer and measured the concentration by the Bradford Total Protein Assay (Bio-Rad Laboratories).
Each 75 micrograms of plasma proteins were loaded overnight on 7 cm pH3-10 nonlinear IPG strips (GE Healthcare Biosciences) followed by the first-dimensional separation using the Ettan IPGphor 3 Isoelectric Focusing Unit (GE Healthcare Biosciences Corp.) and the second-dimensional separation on 12.5% acrylamide gel, 12 mA/gel for 3 h. Gels were stained with SYPRO® Ruby Protein Gel Stain (Invitrogen Corp., Carlsbad, CA, USA) and scanned using a Typhoon FLA 7000 (GE Healthcare). After scanning, gels were stained with Coomassie® Brilliant Blue R 250 dye (Bio-Rad Laboratories) for mass spectrometric analysis.
Spot quantitation was performed with the ImageMaster 2D Platinum 7.0 software Package (GE Healthcare). The intensity of each spot was evaluated as the relative intensity volume (%vol) normalized with the total intensities of all spots on the same gel.
In-gel digestion and LC–MS/MS analysis
Protein spots with significant difference between groups (p < .05) were excised, destained, reduced, and alkylated before incubating at 37°C overnight with a digestion buffer containing 10 ng/µl trypsin. After stopping the reaction with 2% trifluoroacetic acid, the peptides were purified using the ZipTip® Pipette Tips (C18 resin, P10; Millipore Corp., Bedford, MA, USA).
Mass spectrometric analysis was performed using nanoflow liquid chromatography coupled with electrospray ionization/ion trap tandem-mass spectrometry (Bruker Corp., Coventry, UK). Peak lists with a range from 50 to 3000 m/z were searched using the Mascot searching tool (www.matrixscience.com) against the Swiss-Prot human database. Research parameters were set as described in Weeraphan et al. [Citation12].
Enzyme-linked immunosorbent assay (ELISA)
A total of 70 plasma samples consisting of 10 normal, 30 curcuminoids cocktail-treated group (10 each at baseline, month 6, and month 12), and 30 vitamin E cocktail-treated group (10 each at baseline, month 6, and month 12) were analyzed using the commercial ELISA kits for vitronectin and serum paraoxonase/arylesterase 1 (Cloud-Clone Corp., Houston, TX, USA) following the manufacturer’s supplied protocols.
Western blot analysis
Ten micrograms total protein of depleted plasma from healthy controls (n = 10) and patients at baseline, month 6, and month 12 (n = 10 for each group) were resolved in 12.5% SDS-PAGE gels under the constant current 10 mA/gel for 3 h. Proteins were transferred to PDVF membranes under the constant voltage (100 V for 1 h and 11 min) and the membranes were then blocked with 5% bovine serum albumin (BSA) in tris-buffered saline/Tween® 20 (TBS/T) at room temperature for one hour. Primary antibodies used were mouse monoclonal anti-apolipoprotein E (1:1000 dilution, Abcam, Cambridge, MA, USA), rabbit polyclonal anti-prothrombin (1:1000 dilution, Abcam), and mouse monoclonal anti-apoA1 (1:10,000 dilution, Abcam). Blocked membranes were incubated with the primary antibodies at 4°C overnight, washed with TBS/T, and then incubated with respective horseradish peroxidase (HRP)-conjugated secondary antibodies (1:2000 dilution, Dako, Denmark) at room temperature for one hour. Detection was performed using WesternBright ECL (Advansta, California, USA) and ImageQuantTM LAS 4000 digital imaging system (GE Healthcare).
Statistical analysis
The unpaired Student’s t-test was used to analyze the differences of data between normal and patient groups, whereas the differences between time points of treatment were performed with the paired t-test using SPSS 18.0. Data represented in this study were mean ± standard error of the mean (SEM) and the significance was reported at p-value < .05.
Results
There were 1230 ± 28, 1092 ± 28, 1143 ± 27, and 1107 ± 30 spots detected on 2-DE images of the groups of normal volunteers, patients at baseline, month 6, and month 12 of curcuminoids cocktail treatment, respectively. Sixteen spots corresponding 13 proteins presented quantitative differences between time points of the treatment (). Furthermore, 14 differentially expressed spots from patients’ plasma at baseline (1064 ± 37 spots), month 6 (1209 ± 21), and month 12 (1144 ± 34) of vitamin E cocktail treatment were identified as 11 proteins ().
Table 2. Plasma proteins differentially expressed in β-thalassemia/Hb E patients treated with curcuminoids antioxidant cocktail.
Table 3. Plasma proteins differentially expressed in β-thalassemia/Hb E patients treated with vitamin E antioxidant cocktail.
In this study, we observed the significant correlations between vitronectin (VTN) spot intensities and total bilirubin obtained from our previous study [Citation7] (r = −0.517, p = .004 in curcuminoids group; r = −0.384, p = .036 in vitamin E group), as well as between serum paraoxonase/arylesterase 1 (PON1) and blood reactive oxygen species (r = −0.434, p = .016 in curcuminoids group) ( and ).
Figure 1. (A) Correlations between vitronectin intensities and total bilirubin levels; (B) The ELISA results of vitronectin in normal subjects and patients treated with curcuminoids and vitamin E antioxidant cocktails. * p < .001 compared to normal; ** p < .05 compared to normal; a p < .001 compared to baseline; b p < .05 compared to baseline.
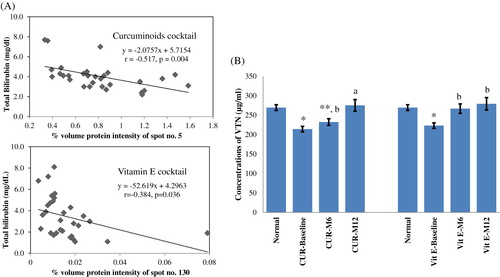
Figure 2. (A) Correlations between paraoxonase/arylesterase 1 intensities and blood reactive oxygen species; (B) The ELISA results of paraoxonase/arylesterase 1 in normal subjects and patients treated with curcuminoids antioxidant cocktail. * p < .001 compared to normal; ** p < .05 compared to normal; a p < .05 compared to baseline.
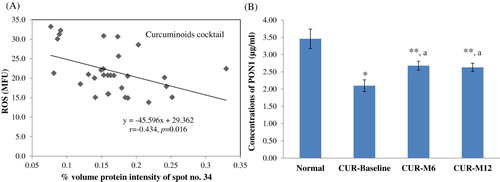
In agreement with the 2-DE results, the levels of VTN in patients’ plasma analyzed by ELISA assays were significantly decreased compared to normal (214 ± 7 and 223 ± 7 µg/ml for baseline curcuminoids and vitamin E groups, respectively, 269 ± 7 µg/ml for normal; p < .001), similar to that observed in PON1 (2.1 ± 0.2 µg/ml for baseline curcuminoids group, 3.5 ± 0.3 µg/ml for normal; p < .001) ( and ). The antioxidant cocktails could enhance their levels in patients’ plasma. During the curcuminoids cocktail treatment, VTN levels increased from 214 ± 7 (baseline) to 232 ± 9 (month 6, p < .05) and 275 ± 15 µg/ml (month 12, p < .001). In the group of vitamin E cocktail, VTN increased from 223 ± 7 (baseline) to 267 ± 12 (month 6, p < .05) and 279 ± 16 µg/ml (month 12, p < .05). PON1 in plasma of patients treated with curcuminoids cocktail rose from 2.1 ± 0.2 µg/ml (baseline) to 2.7 ± 0.1 and 2.6 ± 0.1 µg/ml (month 6 and month 12, respectively, p < .001).
By using Western blot analysis, prothrombin, apoE, and apoA-I were detected as bands at 70, 34, and 30 kDa, respectively (). Both prothrombin and apoE were significantly up-regulated after treating with curcuminoids cocktail for 12 months, whereas there were no significant changes in the vitamin E cocktail group. Both antioxidant cocktails significantly reduced ApoA-I expressions which were consistent with proteomic results.
Figure 3. (A) The bands of Western blots corresponding to prothrombin, apolipoprotein E, and apolipoprotein A-1 of normal subjects (N), thalassemic patients at baseline, month 6 (M6), and month 12 (M12) after curcuminoids- and vitamin E antioxidant cocktails treatments. The graphs (B) and (C) represent the quantitation of the Western blots for these proteins as percentage of the mean value for normal subjects in curcuminoids- and vitamin E antioxidant cocktails groups, respectively. Values were mean ± SEM. * p < .05 and ** p < .01 compared to baseline.
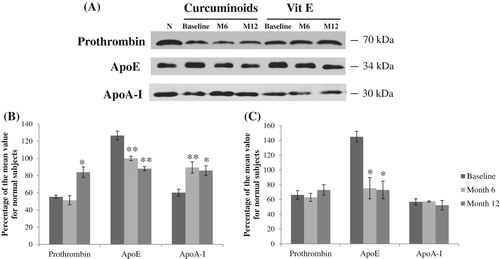
Discussion
In our previous study [Citation7], both curcuminoids- and vitamin E cocktails significantly decreased iron-induced oxidative stress, increased Hb concentrations, and reduced hypercoagulability in β-thalassemia/Hb E patients. ProteoMinerTM Protein Enrichment Kit removed 96.6% of total plasma protein from patients (data not shown), similar to previous studies [Citation13,Citation14]. The proteomic results revealed 13 and 11 plasma proteins with quantitative changes in response to curcuminoids- and vitamin E cocktails, respectively ( and ).
Iron-induced oxidative membrane damage including phosphatidylserine (PS) exposure and band 3 clustering is responsible to the complement-mediated hemolysis and hypercoagulability in thalassemia [Citation15–17]. The significantly increased amounts of immunoglobulins, complement C3, and the membrane attack complex C5b-9 on the circulating RBC membrane from β-thalassemia/Hb E patients were reported [Citation18]. Oxidative stress has been found to upregulate complement C3, a central protein of the complement system, and antioxidant treatment could suppress its expression [Citation19]. This supports our result that the complement C3 levels were decreased throughout the vitamin E cocktail treatment.
Besides alleviation of oxidative stress, antioxidant cocktails may partly improve anemia by the inhibitory effects of curcumin [Citation20] and glutathione [Citation21] on the complement system with unknown mechanism. The increased levels of various inhibitors of complement pathway (e.g. vitronectin, complement factor H, and C4b-binding protein) were also indicated ( and ). Vitronectin inhibits complement-mediated hemolysis at the common terminal pathway by blocking the late complement complex (C5b-9) formation [Citation22]. The moderate correlations between vitronectin and total bilirubin levels, a marker of extravascular and intravascular hemolysis [Citation23], were found in both groups (). Complement factor H has been reported to inhibit the alternative pathway by preventing the formation of C3 and C5 convertases [Citation24], while the alpha chain of C4b-binding protein inhibits the classical and lectin pathways [Citation25].
The proteomic results showed the enhanced levels of coagulation proteins, fibrinogen gamma chain and prothrombin, following the cocktail treatments due to decreased consumption of such coagulation factors as a result of reverse hypercoagulability (), supported by a previous study [Citation12].
The abnormality of plasma lipid profile in thalassemic patients including decreased levels of total cholesterol, high-density lipoprotein cholesterol (HDL-C), and low-density lipoprotein cholesterol (LDL-C), together with increased levels of triglycerides have been reported [Citation26–28]. Our results showed the altered levels of apolipoprotein A-I, A-IV, C-II, and E in response to the treatments. Apolipoprotein E (ApoE) plays important role in regulation of circulating lipoprotein levels, involving in the cholesterol- and triglyceride-rich lipoproteins transport and clearance [Citation29,Citation30]. High apoE levels in our thalassemic plasma were confirmed by Western blot analysis as well as reported in blood microparticles from β-thalassemia/Hb E patients [Citation30]. The levels were decreased toward normal values during the treatments. Apolipoprotein A-I (apoA-I), the major component of HDL, contributes to antioxidant effect of HDL-C by removing oxidized phospholipids and lipid hydroperoxides from LDL, thus preventing LDL oxidation and reducing atherogenesis risk [Citation31]. Curcumin and vitamin E exert anti-atherosclerosis through several mechanisms including increases of plasma HDL-C and apo A-I expressions [Citation32,Citation33]. ApoA-I levels were significantly reduced in plasma of β-thalassemia/Hb E patients and treatments with the antioxidant cocktails increased plasma apoA-I levels, as confirmed by Western blot analysis.
Serum paraoxonase/arylesterase 1 (PON1) is an antioxidant enzyme carried on HDL that also hydrolyzes lipid peroxidation products in LDL [Citation34]. Decreased activities of PON1 associated with increased oxidative stress, decreased Hb levels, and increased atherosclerosis risk have been reported in thalassemic patients [Citation34–37]. Curcuminoids cocktail enhances PON1 intensities in correlation with decreased ROS levels, which was consistent with the ELISA results (), supporting by a previous in vivo study [Citation38].
Glutathione peroxidase 3 (GPx3) also detoxifies hydrogen peroxide and lipid peroxides in the circulation and oxidized LDL. Oxidative stress and hypoxia are strong inducers of GPx3 expression [Citation39,Citation40], therefore the plasma GPx3 levels were decreased after vitamin E cocktail treatment, as well as observed in thalassemic patients chelated with deferiprone [Citation41], or with vitamin E alone [Citation42]. Therefore, antioxidant cocktails may provide anti-atherosclerosis benefit to thalassemic patients through increasing the expressions of apoA-I, PON-1, and GPx3. However, several proteins were also reported with unknown relation to thalassemia. They were alpha-1-microglobulin/bikunin precursor (AMBP), CD5 antigen-like protein, haptoglobin-related protein, myosin-1, gelsolin, tropomyosin alpha-3 chain, and transthyretin.
In conclusion, plasma proteomic analysis has identified several proteins which may be associated with the mechanisms underlying the pleiotropic effects of antioxidant cocktails. Further studies are required to investigate their pathophysiological roles that could improve our understanding and may lead to novel mechanisms or targets for treatment of thalassemia.
Disclosure statement
No potential conflict of interest was reported by the authors.
ORCID
Jisnuson Svasti http://orcid.org/0000-0002-2217-4517
Ruchaneekorn W. Kalpravidh http://orcid.org/0000-0002-8076-2472
Additional information
Funding
References
- Olivieri NF, Pakbaz Z, Vichinsky E. Hb E/beta-thalassaemia: a common & clinically diverse disorder. Indian J Med Res. 2011;134:522–531.
- Rund D, Rachmilewitz E. β-Thalassemia. N Engl J Med. 2005;353(11):1135–1146. doi: 10.1056/NEJMra050436
- Kalpravidh RW, Siritanaratkul N, Insain P, et al. Improvement in oxidative stress and antioxidant parameters in β-thalassemia/Hb E patients treated with curcuminoids. Clin Biochem. 2010;43(4-5):424–429. doi: 10.1016/j.clinbiochem.2009.10.057
- Ozdemir ZC, Koc A, Aycicek A, et al. N-Acetylcysteine supplementation reduces oxidative stress and DNA damage in children with β-thalassemia. Hemoglobin. 2014;38(5):359–364. doi: 10.3109/03630269.2014.951890
- Tesoriere L, D'Arpa D, Butera D, et al. Oral supplements of vitamin E improve measures of oxidative stress in plasma and reduce oxidative damage to LDL and erythrocytes in β-thalassemia intermedia patients. Free Radic Res. 2001;34(5):529–540. doi: 10.1080/10715760100300461
- Elalfy MS, Adly AA, Attia AA, et al. Effect of antioxidant Therapy on Hepatic Fibrosis and Liver iron concentrations in β-thalassemia major patients. Hemoglobin. 2013;37(3):257–276. doi: 10.3109/03630269.2013.778866
- Yanpanitch OU, Hatairaktham S, Charoensakdi R, et al. Treatment of beta-thalassemia/hemoglobin E with antioxidant cocktails results in decreased oxidative stress, increased hemoglobin concentration, and Improvement of the hypercoagulable state. Oxid Med Cell Longev. 2015;2015:537954), doi: 10.1155/2015/537954
- Hagag AA, Elfaragy MS, Elrifaey SM, et al.[CDATA[therapeutic value of combined therapy with deferiprone and Silymarin as iron chelators in Egyptian Children with Beta thalassemia major]]. Infect Disord Drug Targets. 2015;15(3):189–195. doi: 10.2174/1871526515666150731113305
- Hashiguchi A, Tian J, Komatsu S. Proteomic contributions to medicinal plant research: from plant metabolism to pharmacological action. Proteomes. 2017;5(4):35), doi: 10.3390/proteomes5040035
- Guerranti R, Ogueli IG, Bertocci E, et al. Proteomic analysis of the pathophysiological process involved in the antisnake venom effect ofMucuna pruriens extract. Proteomics. 2008;8(2):402–412. doi: 10.1002/pmic.200700265
- Li R, Zhao L, Wu N, et al. Proteomic analysis allows for identifying targets of Yinchenwuling powder in hyperlipidemic rats. J Ethnopharmacol. 2016;185:60–67. doi: 10.1016/j.jep.2016.03.029
- Weeraphan C, Srisomsap C, Chokchaichamnankit D, et al. Role of curcuminoids in ameliorating oxidative modification in β-thalassemia/Hb E plasma proteome. J Nutr Biochem. 2013;24(3):578–585. doi: 10.1016/j.jnutbio.2012.02.008
- Sihlbom C, Kanmert I, Bahr H, et al. Evaluation of the combination of bead technology with SELDI-TOF-MS and 2-D DIGE for detection of plasma proteins. J Proteome Res. 2008;7(9):4191–4198. doi: 10.1021/pr800340c
- Millioni R, Tolin S, Puricelli L, et al. High abundance proteins depletion vs low abundance proteins enrichment: comparison of methods to reduce the plasma proteome complexity. PLoS One. 2011;6(5):e19603), doi: 10.1371/journal.pone.0019603
- Voskou S, Aslan M, Fanis P, et al. Oxidative stress in β-thalassaemia and sickle cell disease. Redox Biol. 2015;6:226–239. doi: 10.1016/j.redox.2015.07.018
- Chapin J, Terry HS, Kleinert D, et al. The role of complement activation in thrombosis and hemolytic anemias. Transfus Apher Sci. 2016;54(2):191–198. doi: 10.1016/j.transci.2016.04.008
- Taher AT, Otrock ZK, Uthman I, et al. Thalassemia and hypercoagulability. Blood Rev. 2008;22(5):283–292. doi: 10.1016/j.blre.2008.04.001
- Malasit P, Mahasorn W, Mongkolsapaya J, et al. Presence of immunoglobulins, C3 and cytolytic C5b-9 complement components on the surface of erythrocytes from patients with?-thalassaemia/HbE disease. Br J Haematol. 1997;96(3):507–513. doi: 10.1046/j.1365-2141.1997.d01-2070.x
- Yang J, Ahn HN, Chang M, et al. Complement component 3 inhibition by an antioxidant is neuroprotective after cerebral ischemia and reperfusion in mice. J Neurochem. 2013;124(4):523–535. doi: 10.1111/jnc.12111
- Kulkarni AP, Ghebremariam YT, Kotwal GJ. Curcumin inhibits the classical and the alternate pathways of complement activation. Ann N Y Acad Sci. 2005;1056:100–112. doi: 10.1196/annals.1352.007
- Perricone C, De Carolis C, Giacomelli R, et al. Inhibition of the complement system by glutathione: molecular mechanisms and potential therapeutic implications. Int J Immunopathol Pharmacol. 2011;24(1):63–68. doi: 10.1177/039463201102400108
- Sheehan M, Morris CA, Pussell BA, et al. Complement inhibition by human vitronectin involves non-heparin binding domains. Clin Exp Immunol. 1995;101(1):136–141. doi: 10.1111/j.1365-2249.1995.tb02289.x
- Barcellini W, Fattizzo B. Clinical Applications of Hemolytic Markers in the differential Diagnosis and Management of Hemolytic anemia. Dis Markers. 2015;2015:635670), doi: 10.1155/2015/635670
- Kopp A, Hebecker M, Svobodova E, et al. Factor h: a complement regulator in health and disease, and a mediator of cellular interactions. Biomolecules. 2012;2(1):46–75. doi: 10.3390/biom2010046
- Ermert D, Blom AM. C4b-binding protein: The good, the bad and the deadly. novel functions of an old friend. Immunol Lett. 2016;169:82–92. doi: 10.1016/j.imlet.2015.11.014
- Al-Quobaili FA, Abou Asali IE. Serum levels of lipids and lipoproteins in Syrian patients with beta-thalassemia major. Saudi Med J. 2004;25(7):871–875.
- Boudrahem-Addour N, Izem-Meziane M, Bouguerra K, et al. Oxidative Status and plasma lipid profile in β-thalassemia patients. Hemoglobin. 2015;39(1):36–41. doi: 10.3109/03630269.2014.979997
- Ghorban K, Shanaki M, Mobarra N, et al. Apolipoproteins A1, B, and other prognostic biochemical cardiovascular risk factors in patients with beta-thalassemia major. Hematology. 2016;21(2):113–120. doi: 10.1179/1607845415Y.0000000016
- Phillips MC. Apolipoprotein E isoforms and lipoprotein metabolism. IUBMB Life. 2014;66(9):616–623. doi: 10.1002/iub.1314
- Chaichompoo P, Kumya P, Khowawisetsut L, et al. Characterizations and proteome analysis of platelet-free plasma-derived microparticles in β-thalassemia/hemoglobin E patients. J Proteomics. 2012;76 Spec No.:239–250. doi: 10.1016/j.jprot.2012.06.004
- Podrez EA. Anti-oxidant properties of high-density lipoprotein and atherosclerosis. Clin Exp Pharmacol Physiol. 2010;37(7):719–725. doi: 10.1111/j.1440-1681.2010.05380.x
- Shin SK, Ha TY, McGregor RA, et al. Long-term curcumin administration protects against atherosclerosis via hepatic regulation of lipoprotein cholesterol metabolism. Mol Nutr Food Res. 2011;55(12):1829–1840. doi: 10.1002/mnfr.201100440
- Kaga E, Karademir B, Baykal AT, et al. Identification of differentially expressed proteins in atherosclerotic aorta and effect of vitamin E. J Proteomics. 2013;92:260–273. doi: 10.1016/j.jprot.2013.06.015
- Zohaib M, Ansari SH, Hashim Z, et al. Serum paraoxonase activity and Malondialdehyde serum concentrations remain unaffected in response to Hydroxyurea therapy in beta-thalassemia patients. J Clin Pharmacol. 2016;56(7):869–874. doi: 10.1002/jcph.675
- Selek S, Aslan M, Horoz M, et al. Oxidative status and serum PON1 activity in beta-thalassemia minor. Clin Biochem. 2007;40(5–6):287–291. doi: 10.1016/j.clinbiochem.2006.10.028
- Labib HA, Etewa RL, Gaber OA, et al. Paraoxonase-1 and oxidative status in common Mediterranean β-thalassaemia mutations trait, and their relations to atherosclerosis. J Clin Pathol. 2011;64(5):437–442. doi: 10.1136/jcp.2011.090209
- Morales N P, Cherlermchoung C, Fucharoen S, et al. Paraoxonase and platelet-activating factor acetylhydrolase activities in lipoproteins of beta-thalassemia/hemoglobin E patients. Clin Chem Lab Med. 2007;45(7):884–889.
- Varatharajalu R, Garige M, Leckey LC, et al. Protective role of dietary curcumin in the prevention of the oxidative stress induced by chronic alcohol with respect to hepatic injury and antiatherogenic markers. Oxid Med Cell Longev. 2016;2016:5017460), doi: 10.1155/2016/5017460
- Bierl C, Voetsch B, Jin RC, et al. Determinants of human plasma glutathione peroxidase (GPx-3) expression. J Biol Chem. 2004;279(26):26839–26845. doi: 10.1074/jbc.M401907200
- Hybertson BM, Gao B, Bose SK, et al. Oxidative stress in health and disease: the therapeutic potential of Nrf2 activation. Mol Aspects Med. 2011;32(4–6):234–246. doi: 10.1016/j.mam.2011.10.006
- Bartfay WJ, Bartfay E. Selenium and glutathione peroxidase with beta-thalassemia major. Nurs Res. 2001;50(3):178–183. doi: 10.1097/00006199-200105000-00009
- Rashidi M, Aboomardani M, Rafraf M, et al. Effects of vitamin E and zinc supplementation on antioxidants in beta thalassemia major patients. Iran J Pediatr. 2011;21(1):8–14.