ABSTRACT
Objective: To explore the correlations between RBCs indexes and the basic coagulation parameters, and provide data for further studies on high altitude-induced thrombotic disease.
Methods: A total of eligible 433 volunteers were divided into different groups according to HGB concentration and HCT, respectively. PT, APTT, TT and Fbg were measured by clotting assays. HGB content, HCT and PLT count were assessed by automated hematology analyzer.
Results: APTT and PT were significantly higher in group 4 (high HGB or HCT groups) (p < 0.05 for all comparison) and PLT count was significantly lower in group 4 than in other groups (p < 0.01 for all comparison). APTT and PT showed negative correlations with HGB concentration (r = −0.168 and −0.165 resp.; both p < 0.01), whereas positive correlations were found between APTT and HCT, PT and HCT (r = 0.225 and 0.258, resp.; both p < 0.01). PLT, TT and Fbg showed no correlation with HGB and HCT.
Conclusions: HGB and HCT may not correlate with basic coagulation parameters in high altitude population, their predictive value for high altitude-induced thrombotic disease may relatively independent and this remain to be determined in further studies.
Introduction
It was reported that over 140 million people worldwide permanently live at altitudes >2500 m above sea level. Low ambient temperature and hypobaric hypoxia are two challenges to life at high altitude (HA) [Citation1,Citation2], with an increased risk of thrombotic diseases following prolonged stay at HA [Citation3–6]. The environmental conditions in HA affect the morphology and phenotype of blood cells [Citation3]. Red blood cell (RBC) count, hemoglobin (HGB) concentrations and hematocrit (HCT) would reach a fairly high level in most of the individuals following long-term exposure to HA situations. Moreover, excessive erythropoiesis, higher HCT and overburdened HGB will lead to hyperviscosity [Citation3,Citation4,Citation7–9].
Except the change of blood flow, thrombosis also involves platelet-vessel interactions and coagulation system [Citation10]. Many studies suggested that HA-induced thromboembolic disorders are a complex or multifactorial trait. The relationship between HA and factors which may increase the incidence of thrombosis have well documented [Citation11], such as HA and RBC, HA and main clotting factors, but whether there is any association among these factors has not been studied.
The objective of this study was to evaluate the correlation of main RBC index (HGB and HCT) with the basic coagulation parameters, through a large cross-sectional study within volunteers reside in Aba Tibetan and Qiang Autonomous Prefecture (>2500 m, located in northwestern Sichuan province of China) and provide data for further studies on the potential mechanism of high altitude-induced thrombotic disease.
Materials and methods
Ethics statement
In this study, the recruited volunteers provided anonymous informed consent, with the information of age, ABO type and gender, according to the requirements of the Ethics Committee of the Institute of Blood Transfusion. All procedures concerning the experiments with human plasma had been given prior approval by the Academic Board of Institute of Blood Transfusion. All clinical research was conducted according to the principles revealed in the Declaration of Helsinki. Participants received no remuneration for their role in the research.
Sample collection and preparation
All the 433 Tibetan volunteers who aged 18y–84y and with no organic disease were resident at high altitudes (>2500 m) more than 1 year. Samples were taken at People’s Hospital of Aba Tibetan and Qiang Autonomous Prefecture, which located in Aba Tibetan and Qiang Autonomous Prefecture, Sichuan Province, China. Inclusion criteria were that all volunteers were ≥18 years and unrelated. Individuals who had prior history of thrombus or hemorrhage, usage of oral anti-coagulant therapy, hepatic disease, HIV infection, pregnancy, diabetes and others were excluded from this study. Plasma samples were drawn in 0.129 M sodium citrate in 9:1 volume ratio by plasmapheresis and then, aliquots were obtained and stored at −70°C until analysis. The samples were divided into the following categories as showed and the basic information of volunteers were showed in .
Table 1. Sample grouping information in this study.*
Table 2. Demographics of volunteers in this study.*
Laboratory assays
Hematological parameters (i.e. HGB concentration, HCT and PLT counts) were assessed using an automated hematology analyzer (Sysmex XE 2100, Kobe, Japan). Prothrombin time (PT), activated partial thromboplastin time (APTT), thrombin time (TT) and fibrinogen (Fbg) were measured by clotting assays using Siemens Healthcare Diagnostics Products GmbH (Marburg, Germany) on a CA-1500 automated coagulation analyzer (Sysmex Corporation, Kobe, Japan).
Statistical analysis
Kolmogorov–Smirnov test was used for the normal distribution of all data, and values were expressed as means and standard deviation (SD) or as medians and 25th–75th quartile when appropriate. Multi-group comparisons were conducted by one-way ANOVA followed by LSD posthoc test. Partial correlation analysis and multivariable linear regression analysis were used to check the association between basic coagulation parameters (APTT, PT, TT, Fbg and PLT) and RBCs indexes (HGB and HCT). A correlation coefficient (r) of 0.10–0.29 indicates a small correlation, 0.30–0.49 a moderate correlation and 0.50–1.0 a high correlation. Statistical analysis was performed using SPSS statistics software, version 17.0 (SPSS Inc., Chicago, IL, U.S.A.). A 95% CI [2.5%–97.5%] was used and a p-value <0.05 was considered statistically significant.
Results
Effect of HGB and HCT on APTT and PT
As showed in (A,B), APTT and PT in group 4 of HGB were significantly higher than group 1, group 2 and group 3 (42.3 ± 15.4 sec vs. 38.2 ± 12.8 sec vs. 38.1 ± 12.7 sec vs. 38.1 ± 13.2 sec for APTT, 14.2 ± 2.9 sec vs. 13.0 ± 1.6 sec vs. 12.6 ± 1.7 sec vs. 12.9 ± 2.2 sec for PT, all p < 0.05). Small negative correlations between APTT and HGB (r = −0.168, p < 0.001, (A)), and PT and HGB (r =−0.165, p = 0.001, (B)) were found by Partial correlation analysis. Further, in a multivariable regression model, APTT and PT were associated with a decrease in HGB (b = −0.478 and −0.072, both p < 0.01; ).
Figure 1. Basic coagulation parameters in different groups. The box plots encompass the 25th–75th quartiles, with the centre lines (—) representing the median values and the solid boxes (▪) showing the mean values. The whisker plots represent 95% CI. ∗ p < 0.05; ∗∗ p < 0.01. The differences between main RBC indexes (HGB and HCT) and basic coagulation parameters (APTT, PT, TT, and Fbg), and main RBC indexes and platelet were calculated using one-way ANOVA followed by LSD post hoc test. (A)–(E) Basic coagulation parameters in different HGB groups; (F)–(J) Basic coagulation parameters in different HCT groups.
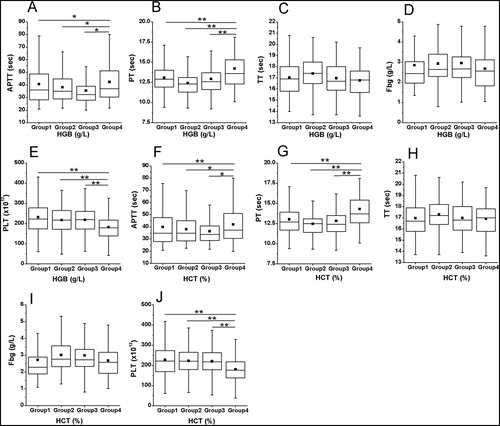
Figure 2. Associations of APTT and HGB, PT and HGB, APTT and HCT, and PT and HCT. Partial correlation analysis was used. (A) Association of APTT and HGB; (B) Association of PT and HGB; (C) Association of APTT and HCT; (D) Association of PT and HCT.
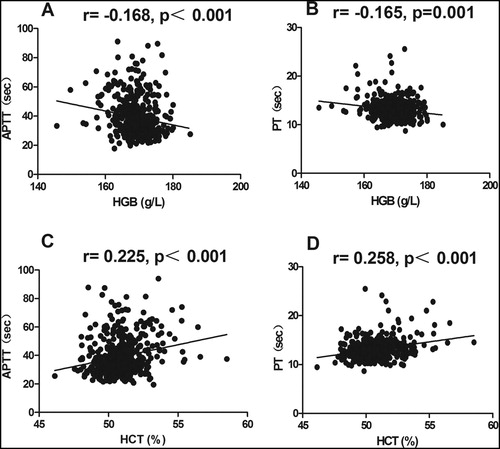
Table 3. Associations between coagulation parameters and RBCs indexes by multivariable analysis.
Similarly, APTT and PT in group 4 of HCT were significantly higher than other three groups (42.4 ± 15.3 sec vs. 37.6 ± 12.8 sec vs. 38.1 ± 12.5 sec vs. 38.6 ± 13.4 sec for APTT, 14.3 ± 2.9 sec vs. 12.9 ± 1.6 sec vs. 12.6 ± 1.4 sec vs. 12.9 ± 2.3 sec for PT, all p < 0.05; (F,G)). And, there was a small positive association between APTT and HCT (r = 0.225, p < 0.001; (C)), and PT and HCT (r = 0.258, p < 0.001; (D)). Moreover, using multivariable regression analysis, APTT and PT retained to have a significant relationship with HCT (b = 2.042 and 0.363, both p < 0.001; ).
Effect of HGB and HCT on PLT
Contrary to the results of APTT and PT we assessed in this study, PLT in group 4 of HGB and HCT were significantly lower than group 1, group 2 and group 3 ((182.0 ± 56.7)×109/L vs. (231.6 ± 77.1)×109/L vs. (220.3 ± 56.1)×109/L vs. (215.0 ± 55.3)×109/L for HGB groups and (181.5 ± 56.6)×109/L vs. (226.4 ± 76.5)×109/L vs. (225.0 ± 57.4)×109/L vs. (217.3 ± 56.0)×109/L for HCT groups; all p < 0.01; (E,J)). No correlation was found between PLT and HGB, and PLT and HCT.
Effect of HGB and HCT on TT and Fbg
TT and Fbg showed no significant distinction among different HGB groups (all p > 0.05; (C,D)), and so did HCT groups (all p > 0.05; (H,I)). Furthermore, no correlation was found between HGB and TT, HGB and Fbg, HCT and TT, and HCT and Fbg (all p > 0.05).
Discussion
With increasing altitude, a progressive reduction in barometric pressure can be observed and one of HA (2500 m above sea level) features is low oxygen pressure [Citation12,Citation13]. It was reported that staying at HA may increase the risk of thrombotic diseases. Hypoxia may induce significant changes of the factors which contribute to thrombosis, such as blood pressure elevation, increase of RBC, HGB and HCT levels, prolongation of PT and APTT, decrease of PLT count and et al. [Citation3,Citation4,Citation13–15]. However, it is still unclear that whether these factors are interrelated. The present study was designed to gain insights into relationship among major factors which play a critical role in thrombosis in hypoxic environment through the study of influences of main RBC indexes (HGB concentration and HCT) on main clotting parameters (PT, APTT, TT, Fbg and PLT count) in HA population.
As RBCs are key players in systemic oxygen transport, in line with their vital role in oxygen transport and delivery, they play a clear role in adaptations to hypoxia [Citation16]. In addition, the function of RBC can reflect the amount of RBC and HGB. It was reported that in response to the insufficient amount of oxygen, HGB and HCT levels significantly increased at HA. The increased HGB is potentially important for improving the oxygen-carrying capacity of RBCs, meanwhile significantly increased HCT values will lead to hyperviscosity and lower blood flow velocity which may augment clinical risks of thrombosis [Citation2–4,Citation12–14]. Thus, the effects of HGB and HCT on coagulation parameters may be different.
PT and APTT tests are commonly ordered in the evaluation of bleeding symptoms and they are measures of the procoagulant cascade. Some studies suggested that changes in the coagulation profile at HA can activate the coagulation cascade and lead to increased thrombosis [Citation15]. However, it seems to be contradictory to that HA is a risk factor for thrombosis, since some studies demonstrated that PT and APTT notably prolonged at HA [Citation2,Citation11,Citation16–19]. And as expected, the results of our study showed HGB and HCT had different influence on APTT and PT: HGB was weakly and negatively associated with APTT and PT (r = −0.168 and r = −0.165 resp., both p < 0.001), otherwise, there was a small positive association among HCT with APTT and PT (r = 0.225 and r = 0.258 resp.; both p < 0.001).These results may be explained by the fact that hypoxia-induced compensatory hyperplasia of RBCs and the increasing of blood viscosity may accelerate the consumption of coagulation factors and ultimately prolonged PT and APTT. It should be noted that correlations between the included variables were weak, they were probably an epiphenomenon, some statistical findings of changes produced by high-altitude itself, and these weak correlations may have little clinical or physiological significance. And it is difficult to make firm conclusions between the erythrocytes and the basic parameters of coagulation with these results. Furthermore, it was reported that some factors such as hypoxia-inducible factor-1 (HIF-1) can influence erythropoiesis and endothelial activation, which finally led to the changes of RBCs and coagulation cascade [Citation20]. Therefore, further studies on these factors are needed to determine the potential mechanism of high altitude-induced thrombotic disease.
PLT is another key factor which plays an indispensable role in thrombogenesis. Our study showed PLT count had no correlation with HGB and HCT in HA volunteers. In addition, there were studies showed different effect of HA conditions on PLT count [Citation11]. Wang et al. showed that PLT counts were lower (p = 0.017) in HA than in low altitude [Citation14], Vij, Lehmann et al., Gray et al. and Chatterji et al. consistent with this result [Citation19,Citation21–23]. Conversely, Sharma, Simon-Schnass et al., Hartmann et al., Hudson et al. and Kotwal et al. demonstrated an elevation in PLT count at HA [Citation24–28]. Furthermore, Sharma found there was no significant difference in PLT counts when 50 subjects were moved from low altitude to 3650 m [Citation29]. These results may be explained by the fact that PLT function may be influenced by physiological changes that occur during adaptation to HA, such as increase of aggregation and adhesion of PLT, but not PLT count [Citation19,Citation21,Citation30]. In addition, the factors which can affect the functions of RBC and PLT, such as 2,3-diphosphoglycerate (2,3-DPG) can facilitate O2 release from hemoglobin, meanwhile, 2,3-DPG inhibits PLT aggregation, but it not change HGB concentration, HCT, and count of PLT [Citation21,Citation31].
Another blood viscosity parameter is Fbg, and TT is used to diagnose blood coagulation disorders and to assess the effectiveness of fibrinolytic therapy. Many studies showed, plasma Fbg level increased at HA and it was positively associated with PAI-1 level [Citation5,Citation11,Citation14,Citation19,Citation28]. PAI-1 is an inhibitor of plasminogen activator and can decrease levels of plasmin, resulting in a decrease of fibrinolytic activity, and thus causes a tendency to thrombosis [Citation28]. The results of our study showed that HGB and HCT had no significant effect on Fbg and TT, which suggest that the effect of Fbg on thrombotic diseases is relatively independent of RBCs at HA, and its mechanism of action remains to be further studied.
Limitations
Naturally, some limitations of the study should be considered. The first limitation of this study is its cross-sectional nature. Second, volunteers are often not fully comparable because they are at different altitude levels and are confounded by a number of different factors, such as climate and latitude. Finally, in this study, we provide evidence that HGB concentration and HCT were showed different effects on factors contributing to clot strength such as PLT count, Fbg, PT, APTT and TT, but how these parameters might relate to the risk of subsequent thrombotic events is not clear.
Conclusion
In summary, this study initiated the evaluation of the influences of HGB concentration and HCT on APTT, PT, TT, Fbg and PLT count in a HA population and provided evidence that HGB and HCT may not correlate with APTT and PT, and no associations were observed among TT, Fbg, PLT and HGB, TT, Fbg, PLT and HCT. These new data showed the changes of RBC, Fbg and PLT at HA may have independent implications to the risk of thrombotic events. Our findings might have important implications for further studies of the formation mechanism of altitude-related thromboses. And we hope that our study would encourage further research to explore the multifactorial induced thromboembolic disorders at HA.
Acknowledgements
We thank all colleagues for their collaboration and assistance. Rong Zhang conceived and designed the research, analyzed the data, wrote the paper, prepared figures and/or tables, reviewed drafts of the paper. Xiaochuan Yu designed the research and collected the data. Yuanzhen Shen, Chunhui Yang and Fengjuan Liu collected the data and prepared figures and/or tables. Shengliang Ye and Haijun Cao performed the research and analyzed the data. Xi Du and Li Ma performed the research and reviewed drafts of the paper. Zongkui Wang and Changqing Li conceived and designed the experiments, wrote the paper, prepared figures and/or tables, reviewed drafts of the paper. The authors thank the People’s Hospitals of Aba for their cooperation in our survey.
Disclosure statement
No potential conflict of interest was reported by the authors.
ORCID
Rong Zhang http://orcid.org/0000-0003-4480-7191
Shengliang Ye http://orcid.org/0000-0003-4477-3075
Additional information
Funding
References
- Liu Y, Zhang JH, Gao XB, et al. Correlation between blood pressure changes and AMS, sleeping quality and exercise upon high-altitude exposure in young Chinese men. Mil Med Res. 2014;1:19. doi: 10.1186/2054-9369-1-19
- León-Velarde F, Maggiorini M, Reeves JT, et al. Consensus statement on chronic and subacute high altitude diseases. High Alt Med Biol. 2005;6(2):147–157.
- Zhong R, Liu H, Wang H, et al. Adaption to high altitude: an evaluation of the storage quality of suspended red blood cells prepared from the whole blood of Tibetan plateau migrants. PLoS One. 2015;10(12):e0144201.
- Liu C, Liu B, Zhang EL, et al. Elevated pentose phosphate pathway is involved in the recovery of hypoxia-induced erythrocytosis. Mol Med Rep. 2017;16(6):9441–9448.
- Rocke AS, Paterson GG, Barber MT, et al. Thromboelastometry and platelet function during Acclimatization to high altitude. Thromb Haemost. 2018;118(1):63–71.
- Chen Y, Jiang C, Luo Y, et al. Interaction of CARD14, SENP1 and VEGFA polymorphisms on susceptibility to high altitude polycythemia in the Han Chinese population at the Qinghai-Tibetan Plateau. Blood Cells Mol Dis. 2016;57:13–22.
- Wu T, Wang X, Wei C, et al. Hemoglobin levels in Qinghai-Tibet: different effects of gender for Tibetans vs. Han. J Appl Physiol. 2005;98(2):598–604.
- Xu J, Yang YZ, Tang F, et al. Epas1 gene polymorphisms are associated with high altitude polycythemia in Tibetans at the Qinghai-Tibetan plateau. Wilderness Environ Med. 2015;26(3):288–294.
- Li K, Gesang L, Dan Z, et al. Transcriptome reveals the overexpression of a kallikrein gene cluster (KLK1/3/7/8/12) in the Tibetans with high altitude-associated polycythemia. Int J Mol Med. 2017;39(2):287–296.
- Johari V, Loke C. Brief overview of the coagulation cascade. Dis Mon. 2012;58(8):421–423.
- Gupta N, Ashraf MZ. Exposure to high altitude: a risk factor for venous thromboembolism? Semin Thromb Hemost. 2012;38(2):156–163.
- Parati G, Agostoni P, Basnyat B, et al. Clinical recommendations for high altitude exposure of individuals with pre-existing cardiovascular conditions: a joint statement by the European Society of Cardiology, the Council on Hypertension of the European Society of Cardiology, the European Society of Hypertension, the International Society of Mountain Medicine, the Italian Society of Hypertension and the Italian Society of Mountain Medicine. Eur Heart J. 2018;39(17):1546–1554.
- Li C, Li X, Liu J, et al. Investigation of the differences between the Tibetan and Han populations in the hemoglobin-oxygen affinity of red blood cells and in the adaptation to high-altitude environments. Hematology. 2018;23(5):309–313.
- Wang Z, Liu H, Dou M, et al. The quality changes in fresh frozen plasma of the blood donors at high altitude. PLoS One;2017:12(4):e0176390.
- Damodar D, Donnally CJ, Sheu JI, et al. A higher altitude is an independent risk factor for venous thromboembolisms after total hip arthroplasty. J Arthroplasty. 2018;33(8):2627–2630. doi: 10.1016/j.arth.2018.03.045
- D'Alessandro A, Nemkov T, Sun K, et al. Altitude omics: red blood cell metabolic adaptation to high altitude hypoxia. J Proteome Res. 2016;15(10):3883–3895.
- Pichler Hefti J, Risch L, Hefti U, et al. Changes of coagulation parameters during high altitude expedition. Swiss Med Wkly. 2010;140(7–8):111–117.
- Wheatley K, Creed M, Mellor A. Haematological changes at altitude. J R Army Med Corps. 2011;157(1):38–42.
- Vij AG. Effect of prolonged stay at high altitude on platelet aggregation and fibrinogen levels. Platelets. 2009;20(6):421–427.
- Bovill EG, van der Vliet A. Venous valvular stasis-associated hypoxia and thrombosis: what is the link? Annu Rev Physiol. 2011;73:527–545.
- Lehmann T, Mairbäurl H, Pleisch B, et al. Platelet count and function at high altitude and in high-altitude pulmonary edema. J Appl Physiol. 2006;100(2):690–694.
- Gray GW, Bryan AC, Freedman MH, et al. Effect of altitude exposure on platelets. J Appl Physiol. 1975;39(4):648–652.
- Chatterji JC, Ohri VC, Das BK, et al. Platelet count, platelet aggregation and fibrinogen levels following acute induction to high altitude (3200 and 3771 metres). Thromb Res. 1982;26(3):177–182.
- Sharma SC. Platelet count in temporary residents of high altitude. J Appl Physiol Respir Environ Exerc Physiol. 1980;49(6):1047–1048.
- Simon-Schnass I, Korniszewski L. The influence of vitamin E on rheological parameters in high altitude mountaineers. Int J Vitam Nutr Res. 1990;60(1):26–34.
- Hartmann S, Krafft A, Huch R, et al. Effect of altitude on thrombopoietin and the platelet count in healthy volunteers. Thromb Haemost. 2005;93(1):115–117.
- Hudson JG, Bowen AL, Navia P, et al. The effect of high altitude on platelet counts, thrombopoietin and erythropoietin levels in young Bolivian airmen visiting the Andes. Int J Biometeorol. 1999;43(2):85–90.
- Kotwal J, Apte CV, Kotwal A, et al. High altitude: a hypercoagulable state: results of aprospective cohort study. Thromb Res. 2007;120(3):391–397.
- Sharma SC. Platelet count on slow induction to high altitude. Int J Biometeorol. 1986;30(1):27–32.
- Alper AT, Sevimli S, Hasdemir H, et al. Effects of high altitude and sea level on mean platelet volume and platelet count in patients with acute coronary syndrome. J Thromb Thrombolysis. 2009;27(2):130–134.
- Kicken CH, Ninivaggi M, Konings J, et al. Hypobaric hypoxia causes elevated thrombin generation mediated by FVIII that is balanced by decreased platelet activation. Thromb Hemost. 2018;118(5):883–892.