ABSTRACT
Objectives: We aimed to determine the clinical and genetic characteristics of a boy diagnosed with the β-thalassemia trait. He also had hereditary spherocytosis (HS) that had been overlooked for 7 years.
Methods: Blood samples collected from the proband and his family were assessed by laboratory tests, and next-generation sequencing (NGS) and Sanger sequencing.
Results: The β-thalassemia trait was complicated with HS in the proband. Compound heterozygous mutations of the Spectrin Alpha, Erythrocytic 1 (SPTA1) gene, c.83G > A and c.190G > A in the proband were inherited from his mother and father, respectively, and he also had the heterozygous c.126_129delCTTT mutation in the Hemoglobin Subunit Beta (HBB) gene. The c.190G > A mutation has not yet been added to the Human Gene Mutation Database (HGMD®). The heterozygous HBB c.126_129delCTTT mutation was inherited from his mother, and his older brother also had this mutation.
Conclusion: Compared with other patients with either HS or β-thalassemia, this proband with both HS and the β-thalassemia trait had very complicated laboratory findings, which resulted in HS being overlooked for 7 years. Genetic testing is invaluable for the differential diagnosis of hereditary anemias with overlapping clinical features.
Introduction
Thalassemia is an autosomal recessive genetic disease characterized by a mutation or deletion of the globin gene, which causes blocked or complete inhibition of globin peptide chain synthesis that results in changes in the composition of hemoglobin. Different globin peptide chain deletions result in α- and β-thalassemia types [Citation1]. The World Health Organization (WHO) estimates that about 350 million people worldwide carry thalassemia genes, and 30 million of them are in China [Citation2]. Hereditary spherocytosis (HS) is the most common hereditary hemolytic anemia caused by an erythrocyte membrane defect. A mutation of the erythrocyte membrane protein gene in HS causes membrane protein defects or abnormalities in ankyrin, band 3, protein 4.2, β-spectrin, and α-spectrin, which are encoded by ANK1, SLC4A1, EPB42, SPTB, and SPTA1, respectively [Citation3,Citation4]. About 75% of HS is caused by autosomal dominant inheritance, mainly of mutations of the ANK1, SLC4A1, or SPTB genes, while the remaining 25% is associated with autosomal recessive inheritance or new mutations, with EBP42 or SPTA1 compound heterozygous mutations being the most common [Citation5,Citation6]. Hereditary spherocytosis can occur in any ethnic group, but it is more prevalent in Northern Europe and North America, with an incidence of up to 1/2000 [Citation7]. To the best of our knowledge, β-thalassemia and HS arising in an individual has seldom been reported, and mutations in both the β-thalassemia and SPTA1 genes in China have not been reported. Herein, we describe HS caused by a novel compound heterozygous mutation of SPTA1 in a 7-year-old boy who had been diagnosed with the β-thalassemia trait, whereas severe HS had been overlooked for the duration of his life. We analyzed the clinical and genetic characteristics of the occurrence of these two conditions in this patient.
Patients and methods
Clinical history
A male infant delivered in the Guangxi Zhuang Autonomous Region of China in 2012 had been diagnosed with the β-thalassemia trait (HBB:c.126_129delCTTT) at the age of 8 months. By the age of one year, his monthly hemoglobin (Hb) level had dropped to 60–70 g/L, and this was treated with repeated transfusions at a local hospital. Splenomegaly gradually worsened enlarged and crossed the midline of the abdomen. He underwent laparoscopic splenectomy in 2015 and was discharged with stable vital signs. Thereafter, the severe anemia that had necessitated repeated transfusions, improved. However, the platelet trend was upward. At the age of 7 years in 2019, his platelet count was 1160 × 109/L and he was referred to our hospital. A physical examination revealed moderate pallor, a soft abdomen, non-palpable liver and spleen under the ribs, but no abnormalities of the cardiopulmonary and other systems. His laboratory results were as follows: red blood cells (RBC) 5.31×1012/L, Hb 104 g/L, mean corpuscular volume (MCV) of 59.81 fL, mean corpuscular hemoglobin (MCH) 19.63 pg, mean sphered corpuscular volume (MSCV) of 65.63 fL, total bilirubin (TBIL) 5.50 μmol/L, indirect bilirubin (IBIL) 2.5 μmol/L, and serum ferritin 2167.89 ng/mL. Target and small spherical erythrocytes, and erythrocyte fragments were obvious in peripheral blood smears.
Erythrocyte osmotic fragility test
The initial and complete hemolysis values were 6.0 (normal range: 4.2–4.6) and 2.8 (normal range: 3.2–3.4) g/L, respectively, results of the Coombs test was negative, glucose 6-phosphate dehydrogenase (G6PD) activity was normal, as were all other laboratory findings.
Pedigree investigation
The father, mother, and older brother were aged 40, 37, and 16 years, respectively; none of them had any clinical history. Both parents were from Guangxi Zhuang, and of Chinese nationality. They were unrelated by marriage and had no family medical history. We therefore further investigated the cause of anemia using hematological, gene, and mutation analyses of the patient and his family.
Hematology
The parents of the proband provided written informed consent to all procedures associated with the study, and the Ethics Committee at the First Affiliated Hospital of Guangxi Medical University approved the study protocol. Thereafter, 4 mL of peripheral venous blood (EDTA-K2 anticoagulant) was collected from the proband and his parents for routine, reticulocyte, and morphological investigations, using an LH780 automatic blood analyzer (Beckman Coulter, Inc., Brea, CA, USA).
Genetic analysis
Variations in the sequences of exons and adjacent introns of disease-related genes in the bloodstream of the patient were analyzed using next-generation (NGS) whole-exome sequencing, to identify mutated genes and sites. Mutation sites identified in the proband and his family were verified by Sanger sequencing at the Genetics and Prenatal Diagnosis Center of the First Affiliated Hospital of Zhengzhou University in China. The pathogenicity of mutant genes was predicted using SIFT, PolyPhen-2, and Mutation Taster on-line software. The protein structure of the mutant gene site was analyzed using HOPE software [Citation8].
Results
Laboratory findings
Peripheral blood smears
The peripheral blood smears of the proband contained mature erythrocytes of various sizes, some expanded, central, lightly-stained areas, oval and spherical and target erythrocytes. Spherical erythrocytes accounted for about 5% of visible erythrocytes. Laboratory findings are shown in . A few abnormal erythrocytes were found in peripheral blood smears from the mother, but not from the father or older brother of the proband ().
Figure 1. Morphology of peripheral blood erythrocytes (Wright–Giemsa, magnification, ×400). (A) Size of mature erythrocytes from proband varies. Some areas of lightly-stained, oval, spherical, and target-shaped central are expanded. (B) Mother; (C) Father; (D) Older brother.
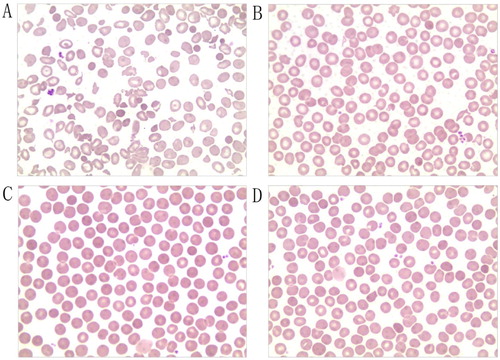
Table 1. Laboratory findings of the proband and his family members.
Genetic analysis
We found c.83G > A and c.190G > A compound heterozygous mutations in exon 2 of the SPTA1 gene (NM_003126.4), and a c.126_129delCTTT heterozygous mutation of the HBB gene (NM_000518.5) in the proband. shows the family tree. The proband inherited the SPTA1:c.83G > A missense mutation from his mother (). The ClinVar database showed that this site was pathogenic, and SIFT, PolyPhen-2, and Mutation Taster software predicted that it was harmful. The proband inherited the SPTA1:c.190G > A missense mutation from his father (), and this novel mutation was not found in the Human Gene Mutation Database (HGMD®). The ClinVar database does not include the results of the pathogenicity analysis of this site, but the SIFT and PolyPhen-2 tools, and Mutation Taster software predicted that the c.190G > A genes would be respectively, benign, harmful, and harmful respectively. The proband inherited the HBB:c.126_129delCTTT frameshift mutation from his mother. The HGMD® describes that this mutation is associated with β-thalassemia. The older brother and the mother both had the same mutation.
Figure 2. Pedigree of a Chinese family with hereditary spherocytosis and β-thalassemia trait. Black arrow, proband.
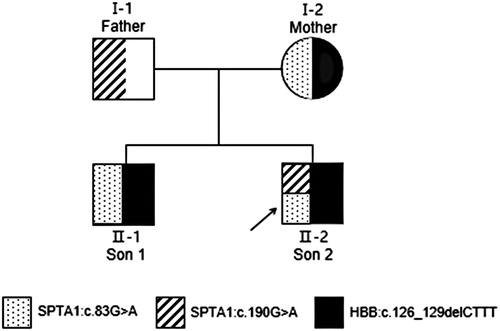
Protein structure analyses of SPTA1:c.83G > A and SPTA1:c.190g > A mutation sites
Protein structure analysis of the c.83G > A mutation site in SPTA1 uncovered a mutant that was smaller than the wild-type residue. The charge on the mutant residue was neutral, whereas the wild-type residue was positively charged, and interaction with other molecules was lost due to the loss of charge (). Protein structure analysis of the SPTA1:c.190G > A mutation site showed that the wild-type and mutant residues were negatively and a neutrally charged, respectively. The wild-type residue forms a salt bridge with Arginine at position 60 and Lysine at position 67. These differences in charge would disturb ionic interactions involving the original, wild-type residue ().
Figure 5. Protein structure analyses of SPTA1:c.83G > A. (A) Schematic structures of original (left) and the mutant (right) amino acids. Red, backbone, which is same for each amino acid. Black, side chain that is unique to each amino acid. (B) Left: overview of protein in ribbon presentation. Proteins are colored as follows: blue, α-helix; red, β-strand; green, turn; yellow, 3/10 helix; cyan, random coil; gray, other molecules. Right: Overview of mutant protein in ribbon presentation. Gray, protein; magenta, side chain of mutated residue (pink spheres). (C) Close-up of mutation. Gray, protein; green and red, wild-type and mutant residues, respectively. Structure is shown from three angles.
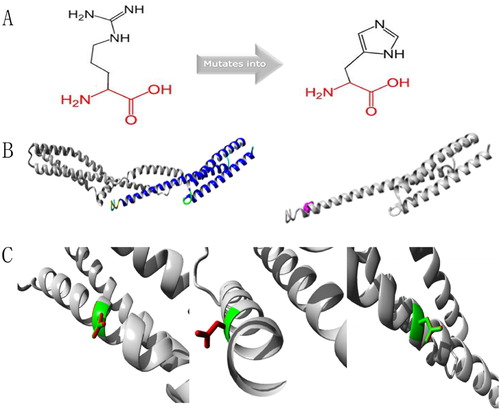
Figure 6. Protein structure analyses of SPTA1:c.190G > A.(A) Schematic structures of original (left) and the mutant (right) amino acids. Red, backbone, which is same for each amino acid. Black, side chain that is unique to each amino acid. (B) Left: overview of protein in ribbon presentation. Proteins are colored as follows: blue, α-helix; red, β-strand; green, turn; yellow, 3/10 helix; cyan, random coil; gray, other molecules. Right: Overview of mutant protein in ribbon presentation. Gray, protein; magenta, side chain of mutated residue (pink spheres). (C) Close-up of mutation. Gray, protein; green and red, wild-type and mutant residues, respectively. Structure is shown from three angles.
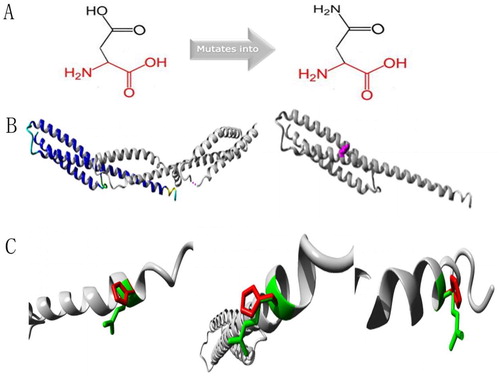
Discussion
A gene mutation of the β-globin peptide chain synthesized on chromosome 11 causes a reduction or deletion in β-globin peptide chain synthesis that causes β-thalassemias [Citation9]. Due to insufficient β-globin peptide chains, relatively abundant α-globin peptide chains polymerize and form inclusion bodies that bind to the erythrocyte membrane skeleton. This reduces deformability and renders erythrocytes vulnerable to oxidative damage, eventually leading to hemolytic and ineffective hematopoiesis [Citation1]. The β-thalassemia gene mutation HBB:c.126 _129delCTTT was discovered in the proband at the age of 8 months, when it should have been an asymptomatic β-thalassemia trait. However, severe anemia developed around that time and monthly blood transfusions were required to maintain Hb levels. However, splenomegaly gradually developed. Splenectomy at the age of 3 years significantly improved the severe anemia. Laboratory findings of the proband upon admission to our hospital showed decreased MCV, MCH, and MSCV values, various sizes of mature erythrocytes in peripheral blood smears, and obvious target and spherical erythrocytes, and erythrocyte fragments. The results of erythrocyte osmotic fragility tests showed increased initial hemolysis, decreased complete hemolysis, a negative Coombs test, and normal G6PD activity. We considered that the proband had not only the β-thalassemia trait but also the hemolytic anemia, HS.
Spherical erythrocytes in peripheral blood smears comprise an important morphological feature of HS. They arise due to a gene mutation that causes defects in the membrane protein, which in turn weaken the vertical connection between the membrane skeleton and lipid bilayer, resulting in destabilization or the loss of the membrane lipid bilayer that forms microvesicles. The erythrocyte membrane surface area eventually decreases, and the ratio of the membrane surface area to volume decreases [Citation10]. Spherical erythrocytes have increased osmotic brittleness, reduced ability to deform, and are easily destroyed as they pass through the spleen, and cause hemolysis. Anemia, jaundice, and splenomegaly are the most common clinical manifestations of HS, but due to the different types of membrane protein defects caused by genetic mutations, the HS clinical manifestations are highly heterogeneous, ranging from asymptomatic carriers to severe hemolysis [Citation11]. The HS diagnosis and management guidelines developed by the British Committee for Standards in Hematology indicate that a person with a family history of HS and typical HS clinical and blood characteristics, can be diagnosed without further tests [Citation6]. However, no family history of HS is one important reason for overlooking HS. Anemia, jaundice, and splenomegaly are clinical manifestations of thalassemia, a G6PD deficiency, and autoimmune hemolytic anemia (AIHA), and are easily confused with HS. Comorbid HS and thalassemia manifest with clinically similar hemolytic anemia phenotypes that could confound clinical judgment. Especially in areas with a high prevalence of thalassemia, HS can be easily overlooked or misdiagnosed.
Through laboratory examination and genetic tests of the proband and his family, we confirmed that the proband had both HS and the β-thalassemia trait. Due to an HS-negative family history, and the similar clinical manifestations between HS and β-thalassemia, the laboratory findings of the proband were ambiguous, and eventually led to HS being overlooked for 7 years. Liao et al. [Citation12] showed that the sensitivity and specificity of a diagnosis HS are 98.25% and 99.10%, respectively, when MCV is higher than MSCV. Among 57 patients with HS in their study, only one had a lower MCV than MSCV, and that patient had HS and β-thalassemia. The proband with both HS and the β-thalassemia trait also had a lower MCV than MSCV. Akar et al. [Citation13] found significantly reduced MCV and MCH among patients with HS and β-thalassemia compared with controls. The MSCV is a reticulocyte parameter. The volume of reticulocytes is smaller than that of normal reticulocytes in patients with HS, which might be due to loss of the spherical erythrocyte membrane surface area at the reticulocyte stage [Citation14]. This might be an important cause of the lower MSCV than MCV in patients with HS. However, when HS is combined with β-thalassemia, MCV might be lower than MSCV because the MCV of β-thalassemia is always decreased. However, healthy people and patients with thalassemia can have a lower MCV than MSCV [Citation12]. Therefore, when HS is combined with thalassemia, HS can be easily overlooked. The pathological changes associated with HS include a reduced surface area of the erythrocyte membrane and cell spheroidization, whereas those associated with thalassemia include deficient cell contents, a relative increase in the erythrocyte membrane surface area, and cellular targeting. In terms of the membrane surface area, these two opposing changes cause interference between hemolysis indices. For example, the osmotic fragility of spherical and target erythrocytes respectively increases and decreases. However, the proband described herein with both HS and the β-thalassemia trait had increased initial and decreased complete hemolytic values in erythrocyte osmotic fragility tests. The erythrocyte fragility did not show a change in the same direction, which might have been due to the mixture of spherical and target erythrocytes.
Relatively few reports have described HS caused by an SPTA1 gene mutation. Changes in ∼5% of patients with HS are caused by an α-spectrin deficiency caused by an inherited, autosomal recessive mutation of the SPTA1 gene [Citation15]. The SPTA1 gene is located at 1q23.1, and the encoded α-spectrin is a component of the erythrocyte skeleton. The α- and β-spectrin protein heterodimers self-aggregate in a head-to-head manner to form tetramers that bind to the lipid membrane via ankyrin, protein 4.2, and band 3 [Citation16]. Tole et al. [Citation17] showed that children with autosomal recessive SPTA1-HS had the most severe clinical phenotype with almost all patients requiring splenectomy in early childhood. Consistent with their findings, the proband with severe clinical manifestations in this study had the novel compound heterozygous mutations, c.83G > A and c.190G > A of the SPTA1 gene, and these were respectively inherited from an asymptomatic mother and father. The novel compound heterozygous mutation is termed a ‘pathogenic variant’ in the ACMG guidelines [Citation18]. The clinical manifestations were severe in the proband but absent in his parents, whose peripheral blood smears contained no obvious abnormal erythrocytes. They were diagnosed as HS carriers only by genetic analyses. The α-spectrin encoded by SPTA1 might be synthesized 2–4-fold faster than β-spectrin during erythropoiesis. Therefore, a single heterozygous mutation in the SPTA1 gene can still synthesize enough α-spectrin to balance β-spectrin and maintain the erythrocyte skeleton, without obvious abnormal erythrocytes in the peripheral blood and clinical manifestations. Only homozygous or compound heterozygous mutations might be involved in the pathogenesis of recessive spectrum protein-deficient HS, and its severity is associated with the amount of α-spectrin in the erythrocyte membrane skeleton [Citation19,Citation20]. The production of <25% α-spectrin results in diseases [Citation21]. The CGT codon in exon 2 c.83 G > A of SPTA1 is mutated to CAT, leading to an Arg 28 His missense mutation, which has also been identified in patients with polycythemia ellipsoides in the HGMD® database. These conditions might be phenotypic manifestations of the diverse expressivity of genetic variants that affect the same genes associated with diseases [Citation22]. Protein structure analysis found that the residue was linked to contact with multimers. The smaller residues introduced at this position by the mutation might be too small to make multimer contacts, which could affect the tetramerization domain. The mutated residue is located on the surface of a domain with an unknown function. The residue was not found to be in contact with other domains with known functions inside the used structure. However, contact with other molecules or domains is still possible and might be affected by this mutation. The GAT codon in exon 2 c.190 G > A of SPTA1 is mutated to AAT, leading to an Asp 64 Asn missense mutation, which has not been added to the HGMD®. Protein structure analysis revealed that the mutation is located within a stretch of repeated residues in the protein, namely, spectrin 1. A mutation of another residue might disrupt this repeat and any subsequent function. The mutated residue is located in a domain that is important for binding to other molecules and is in contact with residues in a domain that is also important for binding. The mutation might disrupt the interaction between these two domains and hence affect the function of the protein. Therefore, the compound heterozygous mutations of c.83G > A and c.190G > A in the SPTA1 gene might have caused HS in this proband with the β-thalassemia trait.
Conclusion
Ambiguous laboratory findings of comorbid HS and the β-thalassemia trait might have caused complications that led to HS being overlooked for 7 years in a young boy. Genetic tests are invaluable for differentially diagnosing hereditary anemias with overlapping clinical manifestations.
Geolocation information
Department of Clinical Laboratory, First Affiliated Hospital of Guangxi Medical University, 6 Shuangyong Road, Nanning, Guangxi 530021, Zhuang Autonomous Region, P.R. China.
Acknowledgements
This work was supported by the National Natural Science Foundation of China (No. 81360263).
Disclosure statement
The authors have no potential conflicts of interest to disclose.
Notes on contributors
Min Chen is the postgraduate of First Affiliated Hospital of Guangxi Medical University in Nanning, Guangxi Zhuang Autonomous Region, China.
Yu-Ping Ye is the postgraduate of First Affiliated Hospital of Guangxi Medical University in Nanning, Guangxi Zhuang Autonomous Region, China.
Lin Liao is the teacher in Department of Clinical Laboratory of First Affiliated Hospital of Guangxi Medical University in Nanning, Guangxi Zhuang Autonomous Region, China.
Xue-Lian Deng is the teacher in Department of Clinical Laboratory of First Affiliated Hospital of Guangxi Medical University in Nanning, Guangxi Zhuang Autonomous Region, China.
Yu-Ling Qiu is the teacher in Department of Pediatrics, First Affiliated Hospital of Guangxi Medical University, Nanning, Guangxi Zhuang Autonomous Region, China
Fa-Quan Lin is the Professor in Guangxi Medical University, Department of Clinical Laboratory, First Affiliated Hospital of Guangxi Medical University and is the Director of Teaching Office, Department of Laboratory Medicine, First Affiliated Hospital of Guangxi Medical University in Nanning, Guangxi Zhuang Autonomous Region, China.
Additional information
Funding
Notes
Hb, hemoglobin; IBIL, indirect bilirubin; MCHC, mean corpuscular hemoglobin concentration; MCH, mean corpuscular hemoglobin; MCV, mean corpuscular volume; MSCV, mean sphered corpuscular volume; MRV, mean reticulocyte volume; RBC, red blood cell; RDW, red cell volume distribution width; Ret, hematocrit; TBIL, total bilirubin.
References
- Taher AT, Weatherall DJ, Cappellini MD. Thalassaemia. The Lancet. 2018;391(10116):155–167.
- Modell B. Global epidemiology of haemoglobin disorders and derived service indicators. Bull World Health Organ. 2008;2008(6):480–487.
- He BJ, Liao L, Deng ZF, et al. Molecular genetic Mechanisms of hereditary spherocytosis: Current Perspectives. Acta Haematol. 2018;139(1):60–66.
- Wang R, Yang S, Xu M, et al. Exome sequencing confirms molecular diagnoses in 38 Chinese families with hereditary spherocytosis. Sci China Life Sci. 2018;61(8):947–953.
- Mohandas N. Inherited hemolytic anemia: a possessive beginner’s guide. Hematology. 2018;2018(1):377–381.
- Bolton-Maggs PH, Langer JC, Iolascon A, et al. General Haematology Task Force of the British Committee for Standards in H. guidelines for the diagnosis and management of hereditary spherocytosis–2011 update. Br J Haematol. 2012;156(1):37–49.
- King MJ, Garcon L, Hoyer JD, et al. ICSH guidelines for the laboratory diagnosis of nonimmune hereditary red cell membrane disorders. Int J Lab Hematol. 2015;37(3):304–325.
- Venselaar H, Te Beek TA, Kuipers RK, et al. Protein structure analysis of mutations causing inheritable diseases. An e-Science approach with life scientist friendly interfaces. BMC Bioinformatics. 2010;11:548.
- Origa R. β-Thalassemia. Genet Med. 2016;19(6):609–619.
- Manciu S, Matei E, Trandafir B. Hereditary spherocytosis - diagnosis, Surgical Treatment and Outcomes. A Literature Review. Chirurgia (Bucharest, Romania). 2017;112(2):110.
- Christensen RD, Yaish HM, Gallagher PG. A Pediatrician's Practical Guide to diagnosing and Treating hereditary spherocytosis in Neonates. Pediatrics. 2015;135(6):1107–1114.
- Liao L, Deng ZF, Qiu YL, et al. Values of mean cell volume and mean sphered cell volume can differentiate hereditary spherocytosis and thalassemia. Hematology. 2014;19(7):393–396.
- Akar N, Gokce H. Red blood cell indexes in patients in patients with hereditary spherocytosis and beta-thalassemia combination. Pediatr Hematol Oncol. 2002;19(8):569–573.
- Nair SC, Arora N, Jain S, et al. Mean reticulocyte volume enhances the utility of red cell mean sphered cell volume in differentiating peripheral blood spherocytes of hereditary spherocytosis from other causes. Indian J Pathol Microbiol. 2015;58(3):307–309.
- Ma S, Deng X, Liao L, et al. Analysis of the causes of the misdiagnosis of hereditary spherocytosis. Oncol Rep. 2018;40(3):1451–1458.
- Wang X, Liu A, Lu Y, et al. Novel compound heterozygous mutations in the SPTA1 gene, causing hereditary spherocytosis in a neonate with Coombsnegative hemolytic jaundice. Mol Med Rep. 2019;19(4):2801–2807.
- Tole S, Dhir P, Pugi J, et al. Genotype-phenotype correlation in children with hereditary spherocytosis. Br J Haematol. 2020;191(3):486–496.
- Rehm HL, Bale SJ, Bayrak-Toydemir P, et al. ACMG clinical laboratory standards for next-generation sequencing. Genetics in Medicine: Official Journal of the American College of Medical Genetics. 2013;15(9):733–747.
- Nussenzveig RH, Christensen RD, Prchal JT, et al. Novel α-spectrin mutation in trans with α-spectrin causing severe neonatal jaundice from hereditary spherocytosis. Neonatology. 2014;106(4):355–357.
- Chonat S, Risinger M, Sakthivel H, et al. The spectrum of SPTA1-associated hereditary spherocytosis. Front Physiol. 2019;10(815):1–7.
- Delaunay J, Nouyrigat V, Proust A, et al. Different impacts of alleles alphaLEPRA and alphaLELY as assessed versus a novel, virtually null allele of the SPTA1 gene in trans. Br J Haematol. 2004;127(1):118–122.
- Iolascon A, Andolfo I, Russo R. Advances in understanding the pathogenesis of red cell membrane disorders. Br J Haematol. 2019;187(1):13–24.