ABSTRACT
Objective: To discover the function of allogeneic hematopoietic stem cell transplantation (allo-HSCT) in ASXL1-mutated acute myeloid leukemia (AML) patients.
Methods: We analyzed the prognostic value of ASXL1 mutations and explored the role of allo-HSCT in 581 AML patients.
Results: According to the definition of intermediate- and adverse-risk AML groups in the European Leukemia Net (ELN), ASXL1-mutated patients had shorter OS and DFS than ASXL1-wild-type patients in the intermediate- and adverse-risk AML groups (3-year OS: 47.5% vs. 60.8%, P<0.001; 3-year DFS: 28.5% vs. 48.9%, P<0.001). Among the cytogenetically normal acute myeloid leukemia (CN-AML), differences were found in both OS (47.4% vs.65.2%, P<0.001) and DFS (21.0% vs. 52.1%, P<0.001) between ASXL1-mutated patients and ASXL1 wild-type patients.
In the ASXL1-mutated AML cohort, the patients received allo-HSCT had longer 3-year OS (P=0.0005) and 3-year DFS (P<0.0001) than those who did not receive allo-HSCT. Multivariate analysis revealed that ASXL1 mutation was an independent prognostic factor for OS (HR 2.248, 95% CI 1.155-4.375, P=0.017), and allo-HSCT had a positive impact on OS (HR 7.568, 95% CI 3.597-15.92, P<0.001) and DFS (HR 2.611, 95% CI 1.688-4.039, P<0.001) in ASXL1-mutated patients.
Conclusion: The results indicate that the presence of ASXL1 mutations is a factor predictive of poor prognosis in AML patients and allo-HSCT could improve the survival of AML patients with ASXL1 mutations.
1. Introduction
Acute myeloid leukemia (AML) is a complicated process that transforms a regulated hematopoietic stem or progenitor cell into a proliferative cell. During this process, gene mutation plays an important role. Several mutated genes have been identified in AML, for example, Runt-related transcription factor 1 (RUNX1), FMS-like tyrosine kinase-3 (FLT3), Nucleophosmin (NPM1), Casitas B-lineage lymphoma (CBL), Ten-Eleven Translocation 2 (TET2), DNA Methyltransferase 3a (DNMT3a), and C-kitproto-oncogene protein (C-Kit). Currently, DNA sequencing for somatic mutational profiling is used to identify recurrent somatic mutations in myeloid malignancies. These reported mutations and genetic alterations help determine prognostic features.
Among these mutations, the additional sex combs like-1 (ASXL1) gene encodes a highly conserved protein which belongs to the enhancer of trithorax and polycomb (ETP) gene family, and ASXL1 has dual functions in both epigenetic activation and the repression of gene transcription [Citation1,Citation2]. The most cases of ASXL1 mutations are frameshift and nonsense mutations, which may lead to the loss of the carboxy-terminal plant homeodomain finger at the protein level [Citation3].
Alterations in the ASXL1 gene happen in hematopoietic cells from patients with a variety of AMLs [Citation1,Citation3–6]. ASXL1 mutations frequently occur in AML patients, which cause the poor survival [Citation7]. But it is still unclear about the role of allogeneic hematopoietic stem cell transplantation (allo-HSCT) in ASXL1-mutated AML patients. Various factors were investigated to deeply learn about the clinical significance of ASXL1 mutations in AML and the role of allo-HSCT in ASXL1-mutated AML. This study also examined the clinical characteristics and outcomes of AML patients with ASXL1 mutations undergoing allo-HSCT.
2. Materials and methods
2.1. Patients
During January 2015 and April 2018, there were 581 de novo AML patients in total without treatment. Those patients enrolled in this study were approved by the ethics committee of the First Affiliated Hospital of Soochow University. During 581, 48 patients have ASXL1 mutations and others has no ASXL1 mutation. To exclude bias from the inconsistent conditions of patients, M3 patients, >65 years old patients and the patients who had weak body function and poor tolerance to the high intensity chemotherapy were not included in the cohort.
All the AML patients were treated with highly intensive induction chemotherapy, which were administered for at least two courses. (shown in .) The induction chemotherapies were consisted of standard first-line treatment of an IA (Idarubicin and cytarabine)-like regimen comprised of Idarubicin 8–12 mg/m2(Days 1–3), and cytarabine 100 mg/m2(Days 1–7). The first complete remission (CR) patients were subsequently treated with intermediate/high-dose cytarabine (1-2 g/m2/d, Days 1-3)-based combination chemotherapy. Those patients, with partial remission (PR) or no remission (NR), received decitabine combined with priming regimen, including decitabine 20 mg/m2 (Days 1-5), IAG(idarubicin 8 mg/m2 (Days 1-3) and cytarabine 10 mg/m2 once every 12 h (Days 1-14) with granulocyte-colony stimulating factor (G-CSF) 300 μg/m2 (Days 0-14)), and CAG (aclacinomycin) 5-8 mg/m2 (Days 1-8) and cytarabine 10 mg/m2 once every 12 h (Days 1-14) with G-CSF 300 μg/m2 (Days 0-14).
2.2. Transplantation regimen
Allo-HSCT patients received a modified Bu/Cy conditioning regimen (mBu/Cy) as follows: cytarabine 2 g/m2/12 h (on Days −9 and −8), busulfan (Bu) 0.8 mg/kg/6 h (on Days −7 to −5), and cyclophosphamide (Cy) 1.8 g/m2 (on Days −4 and-3). The graft-versus-host disease (GVHD) prophylactic treatment included cyclosporine A (CSA, 2.5-3 mg/kg/day starting on Days-10–30, then tapering); short-term methotrexate (MTX) administered on Days +1, +3, +6, and +11 at dosages of 15, 10, 10, and 10 mg/m2, respectively, rabbit anti-thymocyte globulin (ATG; Genzyme, Cambridge, MA) 2.5 mg/kg/d (on Days −5 to −2); and mycophenolate mofetil (MMF, 1.0 g/day from Days −10 to +30). This treatment also depended on the type of transplantation.
2.3. Cytogenetic analyses
The bone marrow (BM) samples of all the AML patients were processed by standard 24 h unstimulated cultures. A conventional R-banding assay was used for karyotypic analysis.
2.4. Analysis of ASXL1 mutations
Mononuclear cells from patient BM samples were enriched by Ficoll density gradient centrifugation. Genomic DNA was extracted from samples by the Wizard® Genomic DNA Purification Kit (Promega, U.S.A.). The Ion S5 system (Personal Genome Machine, ThermoFisher, Grand Island, NY, U.S.A.) was used to evaluate the panel of 51 common variant gene targets in hematologic malignancies, including ASXL1, ASXL2, BCOR, BCORL1, BIRC3, BRAF, CALR, CBL, CEBPA, C-KIT, CSF3R, CSMD1, DNMT3A, ETNK1, ETV6, EZH2, FBXW7, FLT3, GATA2, IDH1, IDH2, IL7R, JAK1, JAK2, JAK3, KRAS, MPL, MYD88, NOTCH1, NPM1, NRAS, PAX5, PDGFRA, PDGFRB, PHF6, PI6, PIGA, PTEN, PTPN11, RUNX1, SETBP1, SETD2, SF3B1, SH2B3, SRSF2, STAG2, TET2, TP53, U2AF1, WT1, and ZRSR2. Polymerase chain reaction (PCR) fragments were bidirectionally sequenced in a 3730XL Sequencer (Applied Biosystems, U.S.A.) and were analyzed by Sequencing Analysis Software 6.0 (Applied Biosystems, U.S.A.).
2.5. Definitions
Complete remission (CR) was defined as follows: the absence of leukemic blasts in blood, <5% blasts in the bone marrow, the absence of Auer rods within the blasts, the absence of an abnormal karyotype, a lack of evidence of extramedullary disease, and an absolute neutrophil count >1 × 109/L and platelet count≥100 × 109/L. Partial remission (PR) was defined as the absence of leukemic blasts in blood, a >50% decrease in blasts, between 5%∼25% blasts in the bone marrow, or <5% blasts in the bone marrow with Auer rods in the blasts. No remission (NR) was defined as leukemic blasts in the blood and bone marrow containing more than 30% blasts.
2.6. Statistical analyses
Student’s t-test was used to compare the age, sex, white cell count, hemoglobin level, platelet count, and BM blast percentages in different groups. The χ2 test or Fisher exact test was used to analyze the interrelation of different gene mutations. Since the numerical data were not normally distributed, the Mann–Whitney test was used to compare two groups. Categorical data were compared by the chi-squared test. Survival was calculated using the Kaplan-Meier method and compared using the log-rank test. In addition, a Cox regression model was applied to identify prognostic variables in AML patients. If comparison between medians of two groups was required, the Mann–Whitney U test was used. Fisher’s exact test was used if differences in proportion comparisons between two groups were pertinent. Additionally, we did landmark analyses to assess outcomes at 3 months and between 3 months and 3 years.
A p value < 0.05 was considered as statistically significant. All calculations were performed using the SPSS software package (version 22.0) and R version R3.1.3.
3. Results
3.1. Patient characteristics
The comparison of the clinical and hematological characteristics of patients with and without ASXL1 mutations is summarized in .
Table 1. Clinical characteristics of the 581 AML patients.
According to the clinical characteristics of 581 de novo AML patients, the patients with ASXL1 mutations were associated with ELN [Citation8] genetic groups, disease status and WHO subtype than the ASXL1-wild-type patients. However, listed that there was no significant difference between the two groups in terms of age, gender, cytogenetic classification, blast percentage in the bone marrow, white cells, hemoglobin or platelets.
The response of induction therapy was that: 64.6% of ASXL1-mutated patients and 83.9% of ASXL1-wild-type AML patients achieved CR. It was indicated more ASXL1-wild-type AML patients who achieved CR than ASXL1-mutated AML patients.
Association of ASXL1 mutations with other concurrent mutations.
Oncogenic mutations were analyzed in 581 patients. Among the 48 ASXL1-mutated AML patients, TET2 was the most common mutated gene (35.4%) coexisting with ASXL1, followed by U2AF1 (14.6%), DNMT3A (14.6%), ETV6 (14.6%), FLT3-ITD (12.5%), RUNX1 (12.5%), KRAS (12.5%), IDH2 (10.4%), WT1 (8.3%), and IDH1(6.3%). The median number of mutations per patient was 2 in the range of 1–6. Furthermore, TET2, U2AF1, ETV6 and KRAS gene mutations were associated with ASXL1 (shown in and S 1).
Table 2. Frequencies and distribution of the other mutation in the AML patients.
3.3. Frequency and types of mutations in 48 ASXL1-mutated AML patients
The ASXL1 mutations were located in exon 12 and might result in the expression of a truncated ASXL1 protein. All the ASXL1 mutations detected in this study were heterozygous. 33.33% of patients with ASXL1 mutation was p. G646fs*12. Other mutation types in our patients were mainly p. E635fs*15 and p. R693. Whith 48 ASXL1-mutated AML patients, 20 patients had frameshift mutations because of insertions or deletions. 28 patients had other anomalies based on the results of sequencing analysis. No significant difference were found between the different frequent mutation types, i.e p. G646fs*12, p. E635fs*15, and p. R693. The analysis also indicated there was no difference in prognosis among patients with different types of AML.
3.4. Survival outcomes for all AML patients with ASXL1 mutations
The survival between ASXL1-mutated AML patients and ASXL-wild-type AML patients were also compared in this study. All the AML patients received an induction chemotherapy regimen for the first time. After that, 264 patients received allo-HSCT and 317 patients received consolidation chemotherapy. Within the allo-HSCT patients, 21 patients had ASXL1-mutations, and their OS at 3 years was 47.5%,which was significantly lower than the 63.6% of OS at 3 years in the ASXL1-wild-type patients (P < 0.001). DFS was 28.5% in the ASXL1-mutated patients, and it was lower than the 51.1% in the ASXL1-wild-type patients (P < 0.001), which was shown in (A,B). During the ELN intermediate- and adverse – risk groups, (C,D) showed significant differences in 3-year OS (47.5% vs. 60.8%, P < 0.001) and DFS (28.5% vs. 48.9%, P < 0.001) between ASXL1-mutated AML patients and ASXL1-wild-type AML patients. Among the CN-AML patients, significant differences were found in both OS (47.4% vs 65.2%; P < 0.001) and DFS (21.0% vs 52.1%; P < 0.001) between ASXL1-mutated patients and ASXL1-wild-type patients (shown in E,F).
Figure 2. Survival outcomes for AML patients with different classification. (A) OS of AML patients with and without ASXL1 mutations; (B) DFS of AML patients with and without ASXL1 mutations; (C) OS of AML patients with and without ASXL1 mutations in intermediate/adverse group; (D) DFS of AML patients with and without ASXL1 mutations in intermediate/adverse group; (E) OS of CN-AML patients with and without ASXL1 mutations (F) DFS of CN-AML patients with and without ASXL1 mutations
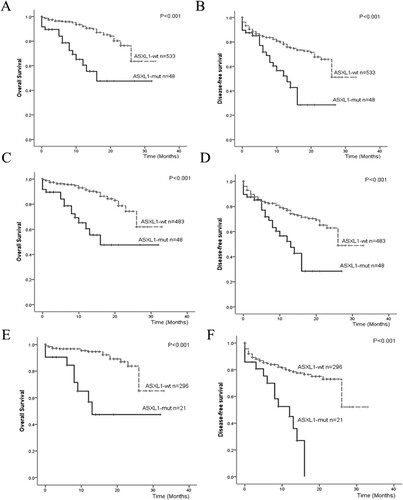
To exclude bias caused by patients who relapsed or died too early to receive transplantation, landmark analysis was performed to compare the outcomes of patients following transplantation with those receiving chemotherapy only.
The time point for landmark analysis was set as 3 months which was the median time from diagnose to transplantation. Significant differences were found between allo-HSCT patients and chemotherapy patients in OS (P = 0.0005) and DFS (P < 0.0001) within the group of ASXL1-mutated AML patients which were shown in (A,B).
3.5. Multivariable analyses for outcomes in AML patients
Multivariate analysis was used to determine the impacts of OS and DFS within 581 AML patients. Those impact factors were age, ASXL1 mutations, FLT3-ITD mutations, treatment, CR status after induction regimens, ELN risk stratification and hemoglobin level. The results indicated that treatment, status after induction regimens, ELN risk stratification and hemoglobin level were significantly and independently associated with worse OS and DFS, Whereas, ASXL1 mutations were associated with worse DFS in univariate analysis and worse OS in multivariate analysis. Furthermore, the results revealed that age was significantly and independently associated with worse OS and DFS in univariate analysis and worse DFS in multivariate analysis (shown in ).
Table 3. Result of Univariable and multivariable analysis for OS and DFS in AML
4. Discussion
The additional sex combs like-1 (ASXL1) gene, an epigenetic regulator expressed in most hematopoietic cell types, is one of the most frequently mutated genes in all subtypes of myeloid malignancies and is associated with an increased risk of mortality in myeloid malignancies[Citation4,Citation9,Citation10].
ASXL1 mutations have been observed in chronic myelomonocytic leukemia (CMML, 45%), myelodysplastic syndromes (MDSs, 16%), primary myelofibrosis (35%), and AML (10.8%) [Citation11,Citation12]. Some studies suggested that the prevalence of ASXL1 mutations in primary AML ranges from 5% to 30%, with a higher prevalence in secondary AML [Citation3,Citation13–15]. Most ASXL1-mutated patients (85%) had concurrent mutations in other genes at diagnosis [Citation16].
In this study of 581 AML patients derived from the prospective single-center data, the M3 patients, >65 years old patients and the patients who had weak body function and poor tolerance to the high intensity chemotherapy were not included. Among these AML patients, an overall incidence of ASXL1 mutations were 8.26%, and the top 4 coexisting genes with ASXL1 mutation in 48 ASXL1-mutated AML patients are TET2, U2AF1, ETV6, and KRAS However, those 4 coexisting genes were not independent risk factors of ASXL1-mutated AML patients in the multivariable analysis, which could be exclusive to ASXL1. Moreover, these patients with mutated ASXL1 had significantly shorter OS and DFS. ASXL1 mutations were identified as adverse factors for OS and DFS in univariable analysis and for OS in multivariable analysis, but not for DFS in multivariable analysis in this study. However, some studies have demonstrated that ASXL1 mutations frequently coexist with RUNX1 mutations [Citation12,Citation17,Citation18] and IDH2 mutations [Citation12,Citation19] and are positively associated with FAB-M0 karyotype [Citation13,Citation14], t(8; 21) [Citation14,Citation20,Citation21], trisomy 8 [Citation12,Citation14] and del(7q)/−7 chromosomal aberrations [Citation9,Citation12] in AML patients. Thol et al.[Citation4] and Bejar et al.[Citation10] demonstrated that ASXL1-mutated MDS patients had poorer OS and higher rates of AML progression, especially in lower-risk patients, but not in higher-risk patients. The results were different from our study, and might be caused by the following reasons. Firstly, sequencing data and samples were missing in a proportion of patients with various cytogenetic risks, induction chemotherapy, transplantation, conditioning regimens and so on in this retrospective study. Secondly, the patient populations were heterogeneous regarding the results, and some censored patients were not followed up. Last but not the least, the subgroup of older patients with CN-AML was included. More research and more clinical data are needed in the future to learn more about the value of ASXL1mutations in predictions of prognosis in AML patients. ASXL1 mutations often are considered as a poor prognosis in AML. Therefore, this study investigated the improvement of the survival of AML patients with ASXL1 mutations by allo-HSCT. This study was the first time to compare the impact of allo-HSCT and chemotherapy on the OS and DFS of AML patients with ASXL1 mutations. The results showed that allo-HSCT had much better performance. It also indicated that allo-HSCT could be considered as the clinical utility to treat the AML patients with ASXL1 mutations.
ASXL1 mutations located in one region within exon 12, were mainly frameshift mutations, which were caused by the deletion or duplication of a nucleotide, and led to the loss of the carboxy-terminal plant homeodomain finger at the protein level [Citation11]. The p.G646fs*12, p.E635fs*15 and p.R693* mutations were the most common mutations in our cohort. The prognostic value of ASXL1 frameshift mutations, which showed no relationship between the presence of ASXL1 frameshift mutations and adverse outcomes in AML patients. The study also found some nonsynonymous changes which were not reported previously. The functional relevance of these unreported mutations is not clear and needs to be investigated in future studies.
Recent biological analysis demonstrated that mutated ASXL1 played pivotal roles in leukemogenesis and led to increased susceptibility to myeloid transformation by altering histone modifications [Citation9]. ASXL1 mutations in genes encoding epigenetic modifiers were usually acquired early and were present in the founding clone [Citation22]. The detection of increased ASXL1 mutations in patients with chronic myeloid disorders such as MDS or CMML before evolution into AML supported that mutations in genes involved in epigenetic regulation occurred as early events in preleukemic progenitor cells [Citation11,Citation12,Citation22–27].
In conclusion, this work suggested that ASXL1 mutation was an independent risk factor for OS in AML patients, and it could be a prognostic indicator unaffected by treatment. Allo-HSCT had a positive impact on the treatment performance of the ASXL1-mutated AML. Determinations of ASXL1 mutations might be useful for the development of other new therapies to improve treatment performance of patients with ASXL1-mutated AML.
Supplemental Material
Download MS Word (360.5 KB)Acknowledgements
Tianhui Xu and Depei Wu performed the research. Zixuan Ding offered results of patients’ mutation. Chang Hou, HuiyingQiu, Xiaowen Tan, Aining Sun, Suning Chen and Yang Xu offered detailed materials for this manuscript. Lili Zhou and Jingnan An wrote the paper.
Disclosure statement
No potential conflict of interest was reported by the author(s).
Additional information
Funding
References
- Rocquain J, Carbuccia N, Trouplin V, et al. Combined mutations of ASXL1, CBL, FLT3, IDH1, IDH2, JAK2, KRAS, NPM1, NRAS, RUNX1, TET2 and WT1 genes in myelodysplastic syndromes and acute myeloid leukemias. BMC Cancer. Aug 2 2010;10:401.
- Fisher CL, Randazzo F, Humphries RK, et al. Characterization of Asxl1, a murine homolog of additional sex combs, and analysis of the Asx-like gene family. Genes (Basel). Mar 15 2006;369:109–118.
- Boultwood J, Perry J, Pellagatti A, et al. Frequent mutation of the polycomb-associated gene ASXL1 in the myelodysplastic syndromes and in acute myeloid leukemia. Leukemia. May 2010;24(5):1062–1065.
- Thol F, Friesen I, Damm F, et al. Prognostic significance of ASXL1 mutations in patients with myelodysplastic syndromes. J Clin Oncol. Jun. 20 2011;29(18):2499–2506.
- Gelsi-Boyer V, Trouplin V, Roquain J, et al. ASXL1 mutation is associated with poor prognosis and acute transformation in chronic myelomonocytic leukaemia. Br J Haematol. Nov 2010;151(4):365–375.
- Abdel-Wahab O, Pardanani A, Patel J, et al. Concomitant analysis of EZH2 and ASXL1 mutations in myelofibrosis, chronic myelomonocytic leukemia and blast-phase myeloproliferative neoplasms. Leukemia. Jul 2011;25(7):1200–1202.
- O'Donnell MR, Tallman MS, Abboud CN, et al. Acute myeloid leukemia, version 3.2017, NCCN clinical practice guidelines in oncology. J Natl Compr Canc Netw. Jul 2017;15(7):926–957.
- Dohner H, Estey E, Grimwade D, et al. Diagnosis and management of AML in adults: 2017 ELN recommendations from an international expert panel. Blood. Jan 26 2017;129(4):424–447.
- Asada S, Fujino T, Goyama S, et al. The role of ASXL1 in hematopoiesis and myeloid malignancies. Cell Mol Life Sci. Jul 2019;76(13):2511–2523.
- Bejar R SK, Abdel-Wahab O, et al. Clinical effect of point mutations in myelodysplastic syndromes. N Engl J Med. Jun. 30 2011;364(26):2496–2506.
- Kakosaiou K, Panitsas F, Daraki A, et al. ASXL1 mutations in AML are associated with specific clinical and cytogenetic characteristics. Leuk Lymphoma. Oct 2018;59(10):2439–2446.
- Paschka P, Schlenk RF, Gaidzik VI, et al. ASXL1 mutations in younger adult patients with acute myeloid leukemia: a study by the German-Austrian acute myeloid leukemia study group. Haematologica. Mar 2015;100(3):324–330.
- Carbuccia N, Trouplin V, Gelsi-Boyer V, et al. Mutual exclusion of ASXL1 and NPM1 mutations in a series of acute myeloid leukemias. Leukemia. Feb 2010;24(2):469–473.
- Chou WC, Huang HH, Hou HA, et al. Distinct clinical and biological features of de novo acute myeloid leukemia with additional sex comb-like 1 (ASXL1) mutations. Blood. Nov 18 2010;116(20):4086–4094.
- Shen Y, Zhu YM, Fan X, et al. Gene mutation patterns and their prognostic impact in a cohort of 1185 patients with acute myeloid leukemia. Blood. Nov 17 2011;118(20):5593–5603.
- Chen TC, Hou HA, Chou WC, et al. Dynamics of ASXL1 mutation and other associated genetic alterations during disease progression in patients with primary myelodysplastic syndrome. Blood Cancer J. Jan 17 2014;4:e177.
- Papaemmanuil E, Gerstung M, Malcovati L, et al. Clinical and biological implications of driver mutations in myelodysplastic syndromes. Blood. Nov. 21 2013;122(22):3616–3627. quiz 3699.
- Schnittger S, Eder C, Jeromin S, et al. ASXL1 exon 12 mutations are frequent in AML with intermediate risk karyotype and are independently associated with an adverse outcome. Leukemia. Jan 2013;27(1):82–91.
- Molenaar RJ, Thota S, Nagata Y, et al. Clinical and biological implications of ancestral and non-ancestral IDH1 and IDH2 mutations in myeloid neoplasms. Leukemia. Nov 2015;29(11):2134–2142.
- Micol JB, Duployez N, Boissel N, et al. Frequent ASXL2 mutations in acute myeloid leukemia patients with t(8;21)/RUNX1-RUNX1T1 chromosomal translocations. Blood. Aug 28 2014;124(9):1445–1449.
- Krauth MT, Eder C, Alpermann T, et al. High number of additional genetic lesions in acute myeloid leukemia with t(8;21)/RUNX1-RUNX1T1: frequency and impact on clinical outcome. Leukemia. Jul 2014;28(7):1449–1458.
- Bullinger L, Dohner K, Dohner H. Genomics of acute myeloid leukemia diagnosis and pathways. J Clin Oncol. Mar 20 2017;35(9):934–946.
- Cancer Genome Atlas Research N, Ley TJ, Miller C, et al. Genomic and epigenomic landscapes of adult de novo acute myeloid leukemia. N Engl J Med. May 30 2013;368(22):2059–2074.
- Corces-Zimmerman MR, Hong WJ, Weissman IL, et al. Preleukemic mutations in human acute myeloid leukemia affect epigenetic regulators and persist in remission. Proc Natl Acad Sci U S A. Feb 18 2014;111(7):2548–2553.
- Jan M, Snyder TM, Corces-Zimmerman MR, et al. Clonal evolution of preleukemic hematopoietic stem cells precedes human acute myeloid leukemia. Sci Transl Med. Aug 29 2012;4(149): 149ra118.
- Kronke J, Bullinger L, Teleanu V, et al. Clonal evolution in relapsed NPM1-mutated acute myeloid leukemia. Blood. Jul 4 2013;122(1):100–108.
- Shlush LI, Zandi S, Mitchell A, et al. Identification of pre-leukaemic haematopoietic stem cells in acute leukaemia. Nature. Feb 20 2014;506(7488):328–333.