ABSTRACT
Objective
High altitude (HA), with the main feature of hypobaric hypoxia, is an independent risk factor for thrombosis. However, little is known on the alterations of fibrinolytic system in adaptation to HA. In this study, we investigated changes of fibrinolytic system parameters between individuals permanently living at HA and low altitude (LA) regions, and provided data for further studies on HA-induced thrombotic disease.
Material and methods
A total of 226 eligible participants, including 103 LA participants, 100 healthy HA subjects and 23 high altitude polycythemia (HAPC) patients, were recruited in this study. Six fibrinolytic parameters, i.e. fibrinogen (Fbg), D-dimer (DDi), antithrombin III (AT-III), plasminogen activator inhibitor-1 (PAI-1), tissue plasminogen activator (tPA) and plasminogen (PLG) were analyzed respectively. PAI-1 and tPA were performed by using bio-immuno-assays and an automated coagulation analyzer was used to conduct Fbg, DDi, AT-III and PLG tests.
Results
Plasma levels of Fbg, DDi, PAI-1 and PLG were significantly higher in healthy HA group than in LA group (all p < 0.05), whereas tPA was significantly lower in healthy HA group. No significant difference in AT-III was observed between healthy HA and LA groups (p > 0.05). All these fibrinolytic parameters showed no significant distinctions between healthy HA subjects and HAPC patients (all p > 0.05). HGB showed no relationship with fibrinolytic parameters in HA cohort.
Conclusion
This study demonstrates that HA environment has a significant effect on fibrinolytic system and provides a foundation for further studies on HA hypobaric hypoxia-induced thrombotic disease.
Introduction
There are three main systems (i.e. coagulative, anticoagulative and fibrinolytic systems) involved in blood homeostatis, including fluidity, thrombosis and hemostasis. The vital function of coagulation system is to form fibrin clots to stop bleeding and to initiate vessel repair, whereas anticoagulation system primarily functions to prevent or to slow down the propagation of fibrin clots and fibrinolytic system also negatively regulates coagulation by breaking down existing fibrin clots [Citation1]. Under normal physiological conditions, coagulation, anticoagulation and fibrinolysis work cooperatively to maintain a subtle balance. Disturbances between the procoagulant and anticoagulant systems may result in bleeding or thrombotic diseases, even a threat to life. Disorders of fibrinolytic system are also relevant to thrombotic disease or bleeding [Citation2–4].
Fibrinolysis is a normal physiological process which ensures localization of fibrin clot formation and clot removal on wound healing, and prevents blood clots from growing and becoming problematic [Citation5,Citation6]. Fibrinolytic system primarily consists of plasminogen, plasmin, plasminogen activators (including tissue plasminogen activator (tPA) and urokinase plasminogen activator (uPA)) and inhibitors (including thrombin-activatable fibrinolysis inhibitor (TAFI), plasminogen activator inhibitor 1 (PAI-1) and α2-antiplasmin). Plasminogen (PLG), a key inactive proenzyme in fibrinolytic system, can be converted into the active enzyme, plasmin, by both tPA and uPA. Plasmin is the principal protease of the fibrinolytic system. Once formed, plasmin rapidly accelerates fibrinolysis by cleaving cross-linked fibrin clot to soluble fibrin degradation products (FDP) while transforms tPA and uPA from single chain to more active double-chain forms via a positive feedback action [Citation7–10]. Fibrinolysis is also negatively regulated by PAI-1 which inhibits both tPA and uPA, and α2-antiplasmin which directly inhibits plasmin [Citation11]. Plasmin-associated fibrinolysis occurs both at level of fibrinogen and at the level of fibrin clots. When inhibitors of plasminogen activation are suppressed, circulating fibrinogen can be directly broken down by plasmin, which generates small peptides (fragments Aα, Bβ and fragment fibrinopeptide B) from the three polypeptide chains of fibrinogen. And the resulting fragment X, which is coagulative, is further cleaved into the smaller non-thrombin-coagulable fragments Y, D and lastly fragment E. At the level of fibrin clots, plasmin cuts the cross-linked fibrin to release D-dimers (DDi) and other fibrin degradation products (FDPs) [Citation12].
The Qinghai-Tibet Plateau, the highest plateau in the world which is famous as the ‘Roof of the World’, covers over a quarter of China's land area (>2,500,000 km2) with an average altitude of over 4000 m and more than 10 million permanent residents [Citation13–15]. There are two challenges to life at high altitude (HA), i.e. low ambient temperature and low oxygen pressure (hypobaric hypoxia), inducing an increased risk of thrombotic diseases following prolonged stay at HA [Citation16]. As a consequence of hypobaric hypoxia, long-term exposure to HA environment will induce some chronic HA diseases, such as high altitude pulmonary edema, high altitude polycythemia (HAPC) and high altitude heart disease [Citation17]. The morbidity of HAPC at Qinghai-Tibetan Plateau is approximately 5–18% and gradually increases with altitude [Citation14]. Numerous studies have showed that HA environmental conditions can activate coagulation cascades, contribute to hypercoagulability and lead to tendency to thrombosis. Most of these studies focused on HA-induced changes of red blood cells (RBC), platelets, coagulant and anticoagulant factors but few on the impact of HA on fibrinolytic system [Citation18–24]. In our previous study, we found that coagulation factors (F)II, FV, FVII, FVIII, FIX, FX, FXI, FXII were significantly lower in HA than in low altitude (LA) blood donors while anticoagulant factors (protein C, protein S and antithrombin III (AT-III)) showed no significant changes between LA and HA blood donors [Citation25]. Our previous proteomic studies also displayed that plasma proteomics profiles significantly altered between high altitude polycythemia (HAPC) patients and HA healthy controls [Citation14], and between HA and LA participants [Citation15]. Moreover, we found that, in HA participants, prothrombin time (PT) and activated partial thromboplastin time (APTT) negatively correlated with hemoglobin (HGB) concentrations [Citation26].
The present study was focused on investigating the changes of fibrinolytic system between HA and LA subjects. Additionally, we analyzed the differences of fibrinolytic parameters between HAPC patients and healthy HA controls. Our findings contribute to a better understanding of the relationship between high altitude hypobaric hypoxia and hemostasis, and may shed light on novel treatment strategies for HA-induced thrombotic or hemorrhagic diseases.
Materials and methods
Ethics statement
All procedures concerning the experiments with human blood specimens were conducted based on the ethical guidelines of the Helsinki Declaration and had been given prior approval by the Ethics Committee of Institute of Blood Transfusion, CAMS&PUMC (No. 201809).
Sample selection
A total of 226 participants with similar lifestyle, age and gender distribution were enrolled in this study (103 LA healthy subjects, 100 healthy HA participants and 23 HAPC patients). Healthy HA participants (healthy HA group) and HAPC patients (HAPC group) with no visits to LA regions in at least 1 years prior to this study were recruited at People’s Hospital of Aba Tibetan and Qiang Autonomous Prefecture, which is located in Aba Tibetan and Qiang Autonomous Prefecture, Sichuan, with an average altitude >3000 m. Healthy LA subjects (LA group) who were reported no visits to HA regions in at least 1 years prior to this study were enrolled from Meishan Blood Center, Sichuan (<500 m). As we reported previously [Citation14,Citation15], the diagnosis of HAPC was HGB ≥ 190 g/L for female or ≥ 210 g/L for male which based on the criteria defined at the 2004 Qinghai International High Altitude Medicine Conference, and it also accompanied by the following clinical manifestations: headache, dizziness, tinnitus, fatigue, insomnia, cyanosis, conjunctival congestion, dyspnea, palpitations, and venous dilatation. Inclusion criteria were that all the recruited participants were ≥18 years old and unrelated. Individuals who had prior history of thrombus or hemorrhage, usage of oral anticoagulation therapy, chronic pulmonary disorders, hepatic disease, HIV infection, pregnancy, diabetes, renal insufficiency and others were excluded from this study. And the participants who were undergoing acute infection were also excluded from the present study.
Sample preparation
Blood specimens were collected and prepared as we previously described [Citation14,Citation15,Citation25–27]. Briefly, two tubes of blood sample of each participant (∼5 mL/tube) were drawn by venipuncture. One tube of blood specimen was immediately subjected to the detection of HGB. Meanwhile, another tube of blood sample was centrifuged at 4000 g for 20 min at 4°C, and then the collected plasma was immediately stored at −70°C in aliquots. Then all the aliquots were shipped to Institute of Blood Transfusion (IBT), CAMS&PUMC in dry ice type environment. Upon arrival to IBT, the specimens were immediately stored at −70°C until analysis. All the fibrinolytic parameters were measured in IBT. Specimens showing hemolysis and/or clot formation were discarded. The demographic information of the participants is shown in .
Table 1. Demographics of participants.
Laboratory assays
HGB concentration was assessed by using an automated hematology analyzer (Sysmex XE 2100, Kobe, Japan). PT, APTT, thrombin time (TT), fibrinogen (Fbg), AT-III, DDi, and PLG were measured by clotting assays using products from Siemens Healthcare Diagnostics Products GmbH (Marburg, Germany) on a CA-1500 automated coagulation analyzer (Sysmex Corporation, Kobe, Japan). PAI-1 and tPA activities were performed by using bio-immuno-assays (Hyphen BioMed, Neuville-sur-oise, France) with Spectra Max M2e (Molecular Devices, Sunnyvale, CA, USA). PT, APTT, TT, and Fbg were assayed by clot-based endpoint method. AT-III and PLG were measured with chromogenic substrate assays. And the activities of PAI-1 and tPA were assessed by ELISA methods.
Statistical analysis
The normal distributions of all data were assessed by Kolmogorov–Smirnov test, and values were expressed as means and standard deviation (SD) or as medians and 25th–75th quartile when appropriate. Comparisons between LA and healthy HA groups, and between healthy HA and HAPC groups were accomplished using Chi-square test or two-tailed unpaired Student’s t-test. When compared healthy HA with HAPC groups, to reduce sample selection bias, an age-, gender-, and sample size-matched model was used (i.e. n = 23 for HAPC vs. n = 23 for healthy HA subjects with age- and gender-matched with HAPC). Bivariate correlation analysis was used to calculate the associations of HGB and fibrinolytic parameters (Fbg, DDi, AT-III, PAI-1, tPA and PLG) in HA group. A Pearson’s correlation coefficient (r) of 0.10–0.29 represents for a small correlation, 0.30–0.49 for a moderate correlation and 0.50–1.0 for a high correlation. Statistical analysis was performed using SPSS statistics software, version 17.0 (SPSS Inc., Chicago, USA). A p-value <0.05 was considered statistically significant.
Results
Participant demographics
Characteristics of participants are shown in . The gender ratio and mean age showed no significant differences between LA and healthy HA groups, so did the healthy HA group and HAPC group. HGB, RBC (red blood count), HCT (hematocrit), PT, APTT, TT and Fbg were significantly higher in healthy HA group than in LA group. Meanwhile, HGB, RBC, HCT, PT, and APTT of HAPC groups were obviously higher than that of healthy HA group, and TT and Fbg showed no difference between the two cohorts.
Fibrinolytic parameters in LA and healthy HA groups
As showed in (A, C, D, and F), Fbg, DDi, PAI-1, and PLG were significantly higher in healthy HA group than in LA group (2.73 ± 0.96 g/L vs. 2.29 ± 0.40 g/L, 0.56 ± 0.48 mg/L vs. 0.44 ± 0.34 mg/L, 2.23 ± 1.34 ng/ml vs. 1.25 ± 1.20 ng/ml and 92.39 ± 15.57% vs. 83.49 ± 10.18%, respectively; all p < 0.05), whereas tPA was significantly lower in healthy HA group than in LA group (0.14 ± 0.06 IU/ml vs. 0.40 ± 0.15IU/ml, p < 0.001; E). No significant difference in AT-III was observed between healthy HA and LA groups (p > 0.05; B). No correlation was found between HGB and other parameters (Fbg, AT-III, DDi, PAI-1, PLG, and tPA) in healthy HA groups (data not shown).
Figure 1. Fibrinolytic parameters in LA group and healthy HA group. The 25th–75th quartiles of the data are shown in box plots, with the center lines (—) and solid boxes (▪) representing the median values and mean values respectively. The whisker plots represent 95% CI (2.5%–97.5%). ∗p < 0.05; ∗∗p < 0.01, ∗∗∗ p < 0.001. The differences between LA group and healthy HA group were calculated using two-tailed unpaired Student’s t-test.
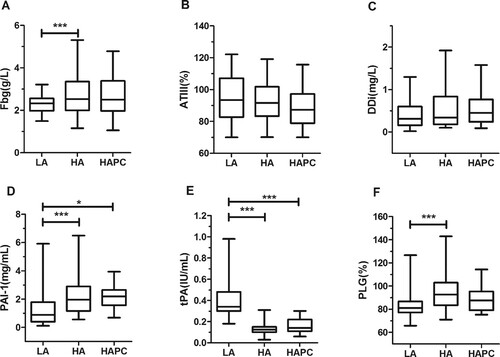
Fibrinolytic parameters in healthy HA and HAPC groups
As shown in , fibrinolytic parameters showed no statistical distinction between healthy HA group and HAPC group (all p > 0.05).
Table 2. Fibrinolytic parameters in healthy HA group and HAPC group.
Discussion
Despite the influences of high altitude on hematological status have been extensively studied in recent years, however, few studies focused on changes of fibrinolytic parameters (such as Fbg, DDi, PAI-1, tPA, and PLG). Besides, it is still unclear that what the differences of fibrinolytic parameters between healthy HA population and HAPC patients are, and whether fibrinolytic parameters are influenced by the elevated HGB levels at HA. In the present study, circulating Fbg, DDi, PAI-1, and PLG were overwhelmingly higher in healthy HA group than in LA group, whereas tPA was lower in healthy HA group. All these fibrinolytic parameters showed no significant distinctions between healthy HA subjects and HAPC patients. And there was no relationship between HGB and fibrinolytic parameters in healthy HA cohort.
Fibrinolysis process occurs inside the body to break down blood clots, including plasmin generation by PLG activators and the subsequent digestion of fibrin or Fbg by plasmin [Citation4]. tPA and PAI-1 play important roles in regulating balance of fibrinolysis. Consistent with previous reports [Citation24,Citation28], we demonstrated that compared to LA group, PAI-1 and PLG of healthy HA group were significantly increased whereas tPA was prominently decreased in HA group (D–F, all p < 0.001). These results suggested that plasmin was attenuated and thus resulted in the decrease of fibrinolytic activity in the HA environment.
It’s well known that thrombin catalyzes Fbg conversion to fibrin clots. Thrombin is the enzyme that is involved in the final step of coagulation cascade and therefore is the target of most anticoagulants [Citation29]. In accordance with of our previous study [Citation25], higher plasma levels of Fbg were found in healthy HA participants. However, Fbg showed no alterations between healthy HA and HAPC groups which was in line with our previous proteomics analysis [Citation14]. AT-III, the predominant anticoagulant in human blood, showed no significant changes between LA and HA groups in our study, which is consistent with previous reports [Citation25,Citation28]. The decreased production of plasmin and no changed AT-III levels may partially explain the elevated Fbg levels in HA participants compared with LA subjects.
Given that DDi has served as a predictive and prognostic biomarker for a number of thrombotic diseases, including disseminated intravascular coagulation [Citation30], deep vein thrombosis [Citation31] and pulmonary embolism [Citation32], as it is a fibrin degradation product which reflects degree of thrombosis and plasmin activity [Citation2]. Our study showed that plasma levels of DDi significantly increased in healthy HA group compared with LA group (C, p < 0.05) which indicated that the secondary fibrinolysis increased in HA subjects even with the decrease of plasmin production. This phenomenon may be a physiologically compensatory response to HA-induced hypercoagulable state when long-term exposure to high altitude conditions (such as hypobaric hypoxia), and play a potential role in adaption to HA environment. However, our findings were inconsistent with other two studies [Citation24,Citation33] which did not displayed significant changes in DDi when exposure to HA. A possible explanation may be that we recruited HA (>2500 m) natives with no reports on visiting to LA areas (<1000 m) in at least 1 year prior to this study, whereas other studies recruited participants with short-term exposure to HA. Further studies are required to verify the physiological relevance and to illuminate the mechanisms.
To the best of our knowledge, it is the first study to analyze the changes of fibrinolytic parameters between healthy HA population and HAPC patients. The incidence of HAPC among Qinghai-Tibetan Plateau populations is approximately 5–18% [Citation34], and it has been a serious public health problem in China. It is well known that RBC counts and HGB concentrations increase in most of individuals following long-term exposure to HA situations [Citation18,Citation19,Citation24,Citation25]. This is the normal physiological response which is helpful to acclimatize to the high altitude-induced hypobaric hypoxia, but continuous increases in RBC counts and levels of HGB will prone to HAPC [Citation14]. One striking feature of HAPC is hyperviscosity of blood. In the present study, all fibrinolytic parameters showed no significant differences between healthy HA people and HAPC patients. In addition, no correlation was found between fibrinolytic parameters and HGB in HA population. These results demonstrated that the hyperviscosity of HAPC patients is mainly caused by increase of RBC counts.
Conclusion
In conclusion, this study estimated the changes of fibrinolytic system between HA natives and LA subjects. It should be noted that this study for the first time reported the differences of fibrinolytic parameters involved in healthy HA population and HAPC patients. The results revealed that plasmin production decreased whereas secondary fibrinolysis increased under HA conditions. No difference of fibrinolytic parameters was found between healthy HA volunteers and HAPC patients, which implied that the changes of fibrinolysis is not one of the main reasons which lead to hyperviscosity of HAPC patients. All these data can be utilized in further studies of the formation mechanism of HA-related thromboses.
Supplemental Material
Download MS Word (25.9 KB)Acknowledgements
The study was supported by the funds from Provincial Science and Technology of Sichuan (Nos. 2019YFS0308 and 2019YFH0018), and CAMS Innovation Fund for Medical Sciences (No. 2016-I2M-1-018). The authors sincerely appreciate Wu Zhao (Sichuan Academy of Medical Sciences & Sichuan Provincial People’s Hospital) for the professional advice on the manuscript.
Disclosure statement
No potential conflict of interest was reported by the author(s).
Additional information
Funding
References
- Sun H. The interaction between pathogens and the host coagulation system. Physiology. 2006;21(4):281–288.
- Chapin JC, Hajjar KA. Fibrinolysis and the control of blood coagulation. Blood Rev. 2015;29(1):17–24. doi:https://doi.org/10.1016/j.blre.2014.09.003.
- Prabhudesai A, Shetty S, Ghosh K, et al. Dysfunctional fibrinolysis and cerebral venous thrombosis. YBCMD blood cells. Mol Dis. 2017;65:51–55.
- Jin NZ, Gopinath SCB. Potential blood clotting factors and anticoagulants. Biomed Pharmacother. 2016;84:356–365.
- Ong CC, Gopinath SCB, Rebecca LWX, et al. Diagnosing human blood clotting deficiency. Int J Biol Macromol. 2018;116:765–773. doi:https://doi.org/10.1016/j.ijbiomac.2018.05.084.
- Batty P, Smith JG. Haemostasis. Surgery (Oxford). 2010;28(11):530–535. doi:https://doi.org/10.1016/j.mpsur.2010.08.008.
- Lijnen HR. Elements of the fibrinolytic system. Ann N Y Acad Sci. 2001;936(1):226–236.
- Wiman B, Collen D. Molecular mechanism of physiological fibrinolysis. Nature. 1978;272(5653):549–550.
- Collen D, Lijnen HR. Basic and clinical aspects of fibrinolysis and thrombolysis. Blood. 1991;78(12):3114–3124. doi:https://doi.org/10.1182/blood.V78.12.3114.bloodjournal78123114.
- Franchini M, Mannucci PM. Primary hyperfibrinolysis: facts and fancies. Thromb Res. 2018;166:71–75.
- Collen D. The plasminogen (fibrinolytic) system. Thromb Haemost. 1999;82(08):837–870.
- Cesarman-Maus G, Hajjar KA. Molecular mechanisms of fibrinolysis. Br J Haematol. 2005;129(3):307–321. doi:https://doi.org/10.1111/j.1365-2141.2005.05444.x.
- Yang R, Xie T, Yang H, et al. Historical trends of organochlorine pesticides (OCPs) recorded in sediments across the Tibetan plateau. Environ Geochem Health. 2018;40(1):303–312.
- Wang Z, Liu F, Ye S, et al. Plasma proteome profiling of high-altitude polycythemia using TMT-based quantitative proteomics approach. J Proteomics. 2019;194:60–69. doi:https://doi.org/10.1016/j.jprot.2018.12.031.
- Du X, Zhang R, Ye S, et al. Alterations of human plasma proteome profile on adaptation to high-altitude hypobaric hypoxia. J Proteome Res. 2019;18(5):2021–2031. doi:https://doi.org/10.1021/acs.jproteome.8b00911.
- Parati G, Agostoni P, Basnyat B, et al. Clinical recommendations for high altitude exposure of individuals with pre-existing cardiovascular conditions: A joint statement by the European Society of Cardiology, the Council on Hypertension of the European Society of Cardiology, the European Society of Hypertension, the International Society of Mountain Medicine, the Italian Society of Hypertension and the Italian Society of Mountain Medicine. Eur Heart J. 2018;39(17):1546–1554.
- West JB. English translation of “Nomenclature, classification, and diagnostic criteria of high altitude disease in China”. High Alt Med Biol. 2010;11(2):169–172. doi:https://doi.org/10.1089/ham.2010.1014.
- Li C, Li X, Liu J, et al. Investigation of the differences between the Tibetan and Han populations in the hemoglobin-oxygen affinity of red blood cells and in the adaptation to high-altitude environments. Hematology. 2018;23(5):309–313. doi:https://doi.org/10.1080/10245332.2017.1396046.
- Zhong R, Liu H, Wang H, et al. Adaption to high altitude: An evaluation of the storage quality of suspended Red blood cells prepared from the whole blood of tibetan plateau migrants. PLoS One. 2015;10(12):e0144201, doi:https://doi.org/10.1371/journal.pone.0144201.
- Liu C, Liu B, Zhang EL, et al. Elevated pentose phosphate pathway is involved in the recovery of hypoxiainduced erythrocytosis. Mol Med Rep. 2017;16(6):9441–9448. doi:https://doi.org/10.3892/mmr.2017.7801.
- Damodar D, Donnally CJ, Sheu JI, et al. A higher altitude is an independent risk factor for venous thromboembolisms after total Hip arthroplasty. J Arthroplasty. 2018;33(8):2627–2630.
- Rocke AS, Paterson GG, Barber MT, et al. Thromboelastometry and platelet function during acclimatization to high altitude. Thromb Haemost. 2018;118(1):063–071.
- Wheatley K, Creed M, Mellor A. Haematological changes at altitude. J R Army Med Corps. 2011;157(1):38–42.
- Gupta N, Ashraf MZ. Exposure to high altitude: A risk factor for venous thromboembolism? Semin Thromb Hemost. 2012;38(2):156–163.
- Wang Z, Liu H, Dou M, et al. The quality changes in fresh frozen plasma of the blood donors at high altitude. PLoS One. 2017;12(4):e0176390. doi:https://doi.org/10.1371/journal.pone.0176390.
- Zhang R, Yu X, Shen Y, et al. Correlation between RBC changes and coagulation parameters in high altitude population. Hematology. 2019;24(1):325–330. doi:https://doi.org/10.1080/16078454.2019.1568658.
- Wang Z, Dou M, Du X, et al. Influences of ABO blood group, age and gender on plasma coagulation factor VIII, fibrinogen, von Willebrand factor and ADAMTS13 levels in a Chinese population. PeerJ. 2017;5:e3156. doi:https://doi.org/10.7717/peerj.3156.
- Kotwal J, Apte CV, Kotwal A, et al. High altitude: a hypercoagulable state: results of a prospective cohort study. Thromb Res. 2007;120(3):391–397.
- Johari V, Loke C. Brief overview of the coagulation cascade. Disease A Month. 2012;58(8):421–423.
- Khalafallah A, Morse M, Stewart P, et al. Evaluation of the innovance d-dimer assay for the diagnosis of disseminated intravascular coagulopathy in different clinical settings. Clin Appl Thromb Hemost. 2014;20(1):91–97.
- van der Hulle T, Tan M, den Exter PL, et al. selective D-dimer testing for the diagnosis of acute deep vein thrombosis: a validation study. J Thromb Haemost Haemost. 2013;11(12):2184–2186.
- Van der Hulle T, Den Exter PL, Erkens PGM, et al. Variable D-dimer thresholds for diagnosis of clinically suspected acute pulmonary embolism. J Thromb Haemostasis. 2013;11(11):1986–1992.
- Crosby A, Talbot NP, Harrison P, et al. Relation between acute hypoxia and activation of coagulation in human beings. Lancet. 2003;361(9376):2207–2208.
- León-Velarde F, Maggiorini M, Reeves JT, et al. Consensus statement on chronic and subacute high altitude diseases. High Altitude Med Biol. 2005;6(2):147–157.