ABSTRACT
Objective
Hypomethylating agents (HMAs) have been reported to target the Sonic Hedgehog (Shh) signaling pathway in myelodysplastic syndrome (MDS). However, the synergistic inhibitory effect of Smo inhibitor jervine and its combination with decitabine in MUTZ-1 cell lines remains lacking.
Methods
We used a CCK-8 assay to detect the in-vitro proliferation rate of MUTZ-1 cell lines. Besides, the Annexin V-FITC/PI double staining flow cytometry was utilized to detect the apoptosis rate and cell cycle changes. The expression levels of mRNA were quantified by using qRT-PCR, and the western blot was employed to detect the expression of proteins.
Results
We found that the single-agent jervine or decitabine can significantly inhibit the proliferation rate of MUTZ-1 cell lines, and this inhibitory effect is time-dependent and concentration-dependent. The combined intervention of the jervine and decitabine can more significantly inhibit cell proliferation, induce cell apoptosis, and block the G1 phase of the cell cycle. The combined intervention of the two drugs significantly reduced Smo and G1i-1 mRNA expression in MUTZ-1 cells. Furthermore, after combining both of the drug treatments, the proteins levels of Smo, G1i-1, PI3K, p-AKT, Bcl2, and Cyclin Dl were significantly downregulated, and Caspase-3 is upregulated, indicating that jervine with its combination of decitabine might be effective for controlling the proliferation, apoptosis, and cell cycle.
Conclusion
The Smo inhibitor jervine and its combination with decitabine have a synergistic effect on the proliferation, cell cycle, and apoptosis of MUTZ-1 cells, and its mechanism may be achieved by interfering with the Shh signaling pathway.
1. Background
Myelodysplastic syndrome (MDS) is a group of heterogeneous clonal diseases that originated from hematopoietic stem cells and are characterized by pathological hematopoiesis of blood cells, and it has a high risk for transforming into acute myeloid leukemia (AML) [Citation1]. The pathogenesis of MDS is still unclear and may be related to various factors such as molecular genetics, hematopoietic microenvironment changes, hematopoietic stem/progenitor cell proliferation, and apoptosis disorders, immune deficiency, and tumor suppressor gene methylation [Citation2,Citation3]. Given that the benefits of MDS patients from traditional chemotherapy are still very limited, and most MDS patients are elderly patients, most patients have lost the opportunity for transplantation. Therefore, for the international prognostic scoring system (IPSS) intermediate-risk-2 and high-risk patients who are not suitable for transplantation, hypomethylating agents (HMAs) have become the first choice for clinical treatment. HMAs are specific DNA methyltransferase inhibitors, including azacitidine (AZA) and decitabine (DAC). The overall response rate of the treatment is about 50%, but the complete remission (CR) rate is only 20%. The factors such as severe bone marrow suppression, long effective time, and the need for multiple courses of consolidation therapy cause poor patient compliance during treatment. Also, the passage of treatment time and the efficacy of some patients will gradually decrease, and the drug resistance is widespread [Citation4–6]. Therefore, to further improve the remission rate and survival time of patients with MDS, exploring the combination therapy with HMAs is the main target for MDS treatment.
The Sonic Hedgehog (Shh) signaling pathway is overexpressed in many types of tumors such as gastric cancer, lung cancer, and leukemia, and is closely related to the occurrence of tumors [Citation7–9]. In our previous studies, we found an activation of the Shh signaling pathway in MDS-stem/progenitor cells, and it participates in the occurrence and development of MDS. Survival analysis showed that the median survival time of patients with high expression of Smo and Gli-1 genes was significantly shorter than that of patients with low expression, which is one of the independent adverse factors. Smo inhibitors were used for in-vitro experiments to interfere with MUTZ-1 cells, and the results showed that they could effectively inhibit cell proliferation and promote cell apoptosis [Citation10]. This is consistent with the research results of Zou and Xavier-Ferrucio [Citation11,Citation12]. The Smo inhibitor jervine is used in this study, a natural steroidal alkaloid isolated from Veratrum calfornicum. Mechanistically, jervine inhibits the Hedgehog signaling pathway through binding to the transmembrane domain of Smo [Citation13].
Preclinical studies have shown that Smoothened (Smo) inhibitors can block the development and survival of cancer stem cells by inhibiting the Hedgehog signaling pathway, thereby playing the role of treating AML or MDS [Citation14]. In 2018, Smo inhibitors were approved by the FDA for the treatment of AML or MDS patients who are not suitable for intensive chemotherapy [Citation15]. The complete remission rate of Smo inhibitors in combination therapy (low-dose cytarabine, HAMs, or standard chemotherapy drugs) for AML and MDS is 17% – 54.2% [Citation16,Citation17]. Based on the above-mentioned clinical research, we believe that the combination therapy of Smo inhibitors such as jervine and decitabine may have a potential synergistic effect, and the combined intervention may have positive significance for prolonging the survival of patients and improving the effectiveness of drug treatment. To identify the mechanism of action of the two-drug combination, we used a single/combined drug intervention program to determine the proliferation rate, apoptosis rate, cell cycle changes, and changes in the Shh expression level signaling-pathway related molecules in MUTZ-1 cells. Based on the substantial regulatory functions of the Shh signaling pathway in cell lines, we observed the phenotypic changes of tumor cells, to explore possible molecular mechanisms of cooperative drug intervention.
2. Materials and methods
2.1. Cells, reagents, and instruments
MUTZ-1 cells were purchased from Shanghai Tongpai Biotechnology Co., Ltd. The other reagents are fetal bovine serum (Gibco, USA), Annexin V-FITC/PI cell apoptosis kit, and CCK8 kit (Jiangsu KGI Biotechnology Co., Ltd.) were purchased from the companies indicted in the bracket. The utilized instruments are enzyme-labeled instrument (BioTek, USA), flow cytometer (BD Bioscience, USA), Q-PCR instrument (Roche, Switzerland), CO2 incubator (Thermo Fisher, USA).
The qRT-PCR kit and primer synthesis were purchased from Dalian Takara Company. Smo inhibitor (Jervine) and decitabine were purchased from Abcam, UK, and Jiangsu Hausen Pharmaceutical Group Co., Ltd., respectively. Mouse monoclonal antibody β-actin, HRP-labeled Goat anti-Mouse Secondary Antibody, HRP-labeled Goat anti-Rabbit Secondary Antibody (Wuhan Boster Bioengineering Co., Ltd.), rabbit monoclonal antibody Smo, Gli-1 (British Abcam company), rabbit polyclonal antibody Bcl2, murine monoclonal antibody Cyclin D1, murine monoclonal antibody PI3K (Wuhan Sanying Biotechnology Co., Ltd. Company), rabbit monoclonal antibody p-AKT (American Cell signaling company), and rabbit polyclonal antibody Caspase-3 (American Affinity company) were also purchased from the indicated companies in the bracket.
2.2. Cell culture
MUTZ-1 cells were cultured in RPMI 1640 medium containing 10% fetal bovine serum in an incubator at 37°C, and 5% CO2 saturated humidity and passaged every 2–3 days. All experiments were in the logarithmic growth phase of the cell.
2.3. Detection of cell proliferation activity by CCK-8 assay
In this study, the concentration gradient of Jervine and DAC was selected based on relevant literature reports and preliminary test results [Citation18]. The logarithmic growth phase cells were inoculated into 96-well plates at 5 × 104 cells/mL, and 100μl cell suspension was inoculated per well. The experiment was divided into a blank control group, an experimental control group, and jervine (0, 1, 5, 10 μmol/L) and, DAC (0, 2, 5, 10μmol/L) treatment group, each group has set up 3 replicate holes. After culturing for 24, 48, and 72 h, add 10μl CCK8solution to each well, then continue the incubation for 4 h at 37°C, and 5% CO2 saturated humidity was maintained. Then, we used a microplate reader to detect the absorbance at 450 nm wavelength, and perform 3 independent repeat experiments, calculate changes in the cells vitality. Record and draw a histogram: draw a histogram with the drug concentration on the horizontal axis and the proliferation rate on the vertical axis. Take the blank control group without cells and the only medium, add 0 μmol/L jervine, 0 μmol/L DAC as the control group, the proliferation rate was calculated as [(absorbance value of the experimental group-absorbance value of the blank group) / (absorbance value of the control group -the absorbance value of the blank group)] × 100%.
2.4. Measurement of cell cycle and apoptosis by using flow cytometry
MUTZ-1 cells were treated with 5 μmol/L Jervine, 5 μmol/L DAC, and the combination of the two drugs, respectively, with a total volume of 3 ml per well. After aseptically cultured in an incubator at 37°C with 5% CO2 saturated humidity for 24 h, the cells of different treatment groups were collected, washed twice with pre-cooled PBS, and the cell density was adjusted to 1 × 106/L. Annexin V-FITC/ PI kit and cell cycle kit were utilized for determining cell apoptosis and cell cycle by flow cytometry, respectively. The above experiments were repeated 3 times, and the average with standard deviation was taken for statistical analysis.
2.5. Identifying protein expression levels
We extracted the total cell protein with RIPA lysate. Take the 20 μl of total protein each time for SDS-PAGE gel electrophoresis (80 V 40 min, 120 V 60 min), and transfer the protein from the SDS-PAGE gel to the PVDF membrane (100 V 120 min). 5% skimmed milk powder closed for 2 h. Dilute Gli-1 and PI3K at a ratio of 1:500, Smo, Caspase-3, and p-AKT at a ratio of 1:1000, Bcl2 at a ratio of 1:2000, Cyclin D1 at a ratio of 1:5000, and β-actin at a ratio of 1:200. Then, dilute the corresponding HRP-labeled rabbit secondary antibody and mouse secondary antibody according to 1:50000, and incubate at 37°C for 2 h for developing the chromogenic substrate.
2.6. Isolation of total RNA and fluorescence real-time quantitative PCR
Total RNA was extracted from MUTZ-1 cells with TRIzol reagent (Invitgen, CA, USA) and reverse transcribed into cDNA with reverse transcriptase reagent (Takara). The primer sequences are shown in . 25 μLinjected for PCR amplification, reaction conditions are: 50˚C for 2 min, pre-denaturation time is 95 ˚C for 10 min, 95 ˚C 30 s, 60 ˚C for 30 s, fluorescence signal collected at 60°C, the cycle is 40 times. The results were normalized to the expression of the gene and are presented as the fold change (2−ΔΔCT).
Table 1. Sequence and length of target gene.
2.7. Statistical analysis
We used SPSS23.0 statistical software for analyzing the data. The relative expression level of genes was analyzed by the method of 2-△△Ct. Since the results did not obey the normal distribution, we converted the expression value into log2 normalization for performing statistical analysis. The data are expressed as mean ± standard deviation (X ± SD). The comparison of means between multiple groups was analyzed by single-factor analysis of variance. We used the multiple comparisons when the variances are uniform with the LSD-t method, and when the variances are not uniform, we used the multiple comparisons between the groups by using Dunnett’s T3 method. We utilized a two-tailed test, and the significance threshold level was considered P<0.05. The synergistic effect of the combination drug was analyzed by CompuSynsoftware. CI < 1 indicates synergy, CI=1 indicates additive effect, and CI > 1 indicates antagonistic effect.
3. Result
3.1. The effect of different concentrations of Smo inhibitor jervine on the proliferation of MUTZ-1 cells
The proliferation assay showed that different concentrations of jervine have a growth inhibitory effect on MUTZ-1 cells, and it increases with the increase of the dose and the extension of the action time, and it is dose-dependent (r= – 0.977; p = 0.000) and time dependence (r = −0.980, P = 0. 020). After treatment with 0, 1, 5, and 10 μmol/L of jervine for 24 h, the proliferation rates of MUTZ-1 cells were (99.88 ± 0.12)%, (93.39 ± 0.93)%, (87.88 ± 1.72)%, and (78.76 ± 0.75)%, respectively. We found significant differences between the groups when compared with the control (), and when the Jervine concentration is ≥5 μmol/L, the cell proliferation rate is significantly lower than the negative control group (P < 0.01). It is worth noting that the proliferation rates of MUTZ-1 cells were 100%, (87.88 ± 1.72)%, (80.51 ± 0.95)%, and (75.57 ± 1.26)% after treatment with 5 μmol/L Jervine for 0, 24, 48, and 72 h, respectively. There are also significant differences between the groups. Therefore, the use of 5μmol/L of jervine can effectively inhibit the proliferation of MUTZ-1 cells ().
Figure 1. Proliferation rate of MUTZ-1 cells treated with Jervine (X ± SD, n = 3). A. Inhibitory effect of multiple concentrations of jervine on the proliferation of MUTZ-1 cells. B. The representation of the time- and dose-dependent effects of jervine on cell proliferation in MUTZ-1 cells. *P < 0.01.
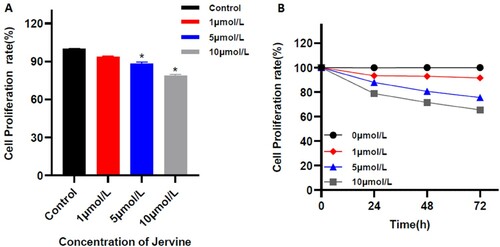
3.2. The effect of different concentrations of DAC on the proliferation of MUTZ-1 cells
The in-vitro study showed that the different concentrations of DAC have inhibitory effects on the proliferation of MUTZ-1 cells. Inhibitory effects increase with increasing the dose and the extension of the action time, and it is dose-dependent (r = −0.990; p = 0.000) and time-dependent (r = −0.963, P = 0. 037). After 24 h of treatment with 0, 2, 5, and 10 μmol/L DAC, the proliferation rates of MUTZ-1 cells were (99.54 ± 0.69)%, (91.52 ± 2.51)%, (84.27 ± 0.66)% and (75.21 ± 1.10)%, respectively. The proliferation rate has significant differences between groups, and when the DAC concentration is ≥5 μmol/L, the cell proliferation rate is significantly lower than the negative control group (P < 0.01). And after using 5 μmol/LDAC for 0, 24, 48, and 72 h, the proliferation rates of MUTZ-1 cells were significantly reduced to 100%, (84.27 ± 0.66)%, (79.21 ± 0.98)% and (72.87 ± 2.58)%, respectively. Therefore, the use of 5 μmol/L of DAC can effectively inhibit the proliferation of MUTZ-1 cells ().
3.3. Jervine and its combination with DAC synergistically inhibits the proliferation of MUTZ-1 cells
The in-vitro CCK8 assay was used to quantifying the proliferation rate of MUTZ-1 cells after combining the Jervine with DAC for 24 h. We used the CompuSyn software to analyze the inhibitory effects of the two drugs on the proliferation of MUTZ-1 cells and the synergy index of the combined effects of the two drugs. We divided the experiment into 4 different groups: blank or control group, J (Jervine 5 μmol/L) group, D (DAC 5 μmol/L) group, and J + D (5 μmol/L Jervine combined with 5 μmol/L DAC) group and found that the significant differences among the groups. The results of this study showed that both single-drug and combined drugs could significantly inhibit the proliferation of the MUTZ-1 cell line (). Interestingly, the J + D (5 μmol/L Jervine combined with 5 μmol/L DAC) group showed a lower proliferation rate of MUTZ-1 cells than that of the single-drug group (P < 0.01) (). The combination index (CI) of the combined two-drug on MUTZ-1 cells is less than 1 (), suggesting that the J + D (5 μmol/L Jervine combined with 5 μmol/L DAC) group has a significant synergistic effect on the lowering of MUTZ-1 cells proliferation.
Figure 3. Comparison of cell Proliferation rate among the multiple groups. Jervine alone (5 μmol/L), DAC alone (5 μmol/L), and jervine and its combination with DAC (X ± SD, n = 3). **P < 0.01.
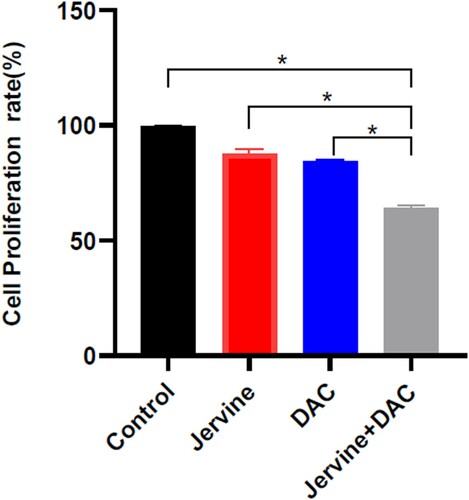
Figure 4. Combination effects of Jervine and DAC in MUTZ-1 cells (X ± SD, n = 3). The combination index of the two drugs on MUTZ-1 cells was less than 1, and the proliferation rate of the combination group was significantly lower than that of the single-drug group. J represents the Jervine intervention group, D represents the decitabine intervention group, J + D represents the combination group of jervine and decitabine.
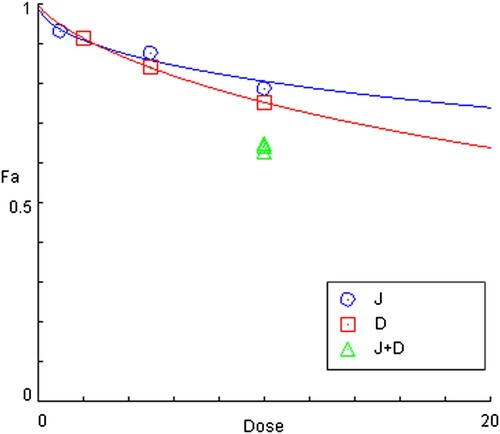
3.4. Cell apoptosis rate is induced by jervine and its combination with decitabine in MUTZ-1 cells
We used Jervine, DAC, and a combination of Jervine and DAC to analyze the apoptosis rate of MUTZ-1 cells for 24 h and compare the apoptosis rate of each group. Our results showed that both the single-drug and combination of drugs could significantly promote cellular apoptosis of the MUTZ-1 cell line (A-B). Interestingly, the apoptosis rate of MUTZ-1 cells is considerably higher for the drug combination intervention group (jervine and DAC) than that of the single-drug group (jervine or DAC) (P < 0.01) ().
Figure 5. Jervine (5 μmol/L), decitabine (5 μmol/L), and their combined effects on cellular apoptosis. A. Comparative cell apoptosis rate after treatment with jervine, decitabine, and their combinations. B. MUTZ-1 cells were exposed to jervine, decitabine, and their combination at indicated concentrations for 24 h and apoptosis was determined by Annexin V-FITC/PI staining.
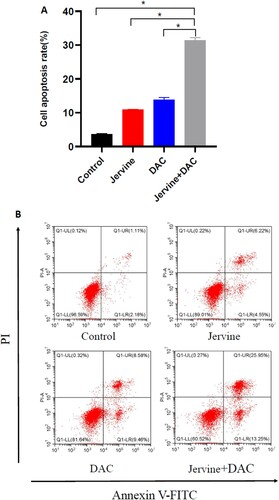
3.5. The effect of Jervine and DAC single drug and combined drug on the cell cycle of MUTZ-1
Since jervine, decitabine, and their combination (Jervine + DAC) significantly inhibited the proliferation rate, we anticipated that the cell cycle arrest could be contributed to their synergistic effects on MDS. So, we applied the single-drug Jervine and DAC as well as the combination of both drugs (Jervine + DAC) on MUTZ-1 cells for 24 h. Then we detected the cell cycle changes among all groups. The results showed that both single-drug and their combination of drugs could significantly increase the number of cells in the G1 phase but significantly reduced the cells in the S-phase (A-E). Interestingly, the combined intervention of drugs (Jervine + DAC) can change the cell cycle more considerably than the single drugs (Jervine or DAC) (P < 0.01) (A-E). This finding indicates that the combination of both drugs (Jervine + DAC) is associated with the regulation of the cell cycle in the MDS cell line.
Figure 6. Comparison of the cell cycle after the intervention of jervine, decitabine, and their combination (Jervine 5 μmol/L, DAC 5 μmol/L) A. Cell cycle rate is increased in G1 phase but decreased in the S phase. B-E. MUTZ-1 cells were treated for 24 h and then flow cytometry was utilized to analyze the cell cycle.
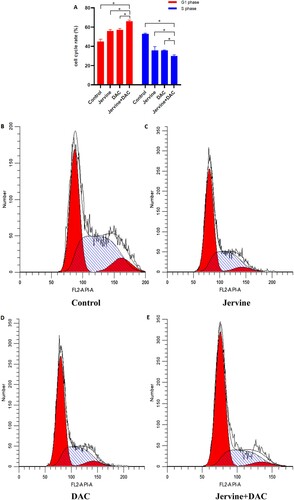
3.6. The effect of single drug jervine or DAC and their combining intervention on Smo and Gli-1 gene expression
Since Smo and Gli-1 are key regulatory downstream effector molecules of the Shh signaling pathway [Citation19], we quantified the expression level of Smo and Gli-1 gene expression in MUTZ-1 cells after 24 h treatment. The qRT-PCR results showed that the combination group (Jervine + DAC) significantly (P < 0.01) downregulated the mRNA expression of Smo and Gli-1in MUTZ-1 cells when compared with the control group (). Furthermore, the pairwise comparison showed that the reduction of Smo and Gli-1 gene expression levels was more significant (P < 0.05) in the combination group of drugs (Jervine + DAC) than that of the Jervine and DAC single-drug groups (). Altogether, this result indicates that the combination of both drugs (Jervine + DAC) is associated with the downregulation of the Shh signaling pathway by reducing the Smo and Gli-1 gene expression.
3.7. The effect of jervine alone and its combination with DAC on the expression levels of critical regulatory proteins in MUTZ-1 cells
The Smo, Gli-1, PI3K, p-AKT, Bcl-2, Cyclin D1, and caspases-3 proteins are the essential regulators for controlling the Shh pathway, PI3K/AKT signaling pathway, cellular proliferation, cell cycle, and apoptosis. To understand the molecular mechanism underlying synergistic effects of Jervine and DAC on the MDS, we explored the expression levels of Smo, Gli-1, PI3K, p-AKT, Bcl-2, Cyclin D1, and caspases-3 proteins in MUTZ-1 cells for 24 h treatment of these compounds alone or combination. Western blotting explored that the single-drug group and the combination group downregulated the expression levels of Smo, Gli-1, PI3K, p-AKT, Bcl-2, and Cyclin D1 protein than those in the control group. There was a significant (P < 0.01) downregulation of the pro-apoptotic protein, Caspase-3, which is associated with remarkable induction of apoptosis in tumor cells (A-B). Furthermore, we compared the expression levels of all key regulatory proteins in different groups and found that the expression level of Smo, Gli-1, PI3K, p-AKT, Bcl2, and Cyclin D1 proteins are significantly downregulated in the two-drug combination group than that of Jervine and DAC single-drug group. The expression level of apoptosis-inducing protein Caspase-3 showed the opposite trend, significantly (P < 0.05) upregulated in the two-drug combination group than in the single–drug group (). Since Smo and Gli-1 are associated with Shh signaling pathway, PI3K and p-AKT are regulating PI3K/AKT signaling pathway, Bcl2 and Caspase-3 are linked with apoptosis, and Cyclin D1 controlling the cell cycle, our findings indicated that the combination of these two drugs (jervine + DAC) is associated with the upregulation or downregulation of these signaling molecules in MDS. Altogether, Jervine and its combination with DAC contributed to regulate the expression levels of critical regulatory proteins including Smo, Gli-1, PI3K, p-AKT, Bcl-2, CyclinD1, and Caspase-3, and this combined therapy (jervine + DAC) ultimately associated with the downregulation of Shh signaling pathway, inhibition of cellular proliferation, arresting cell cycle, and inducing apoptosis in MUTZ-1 cells.
Figure 8. The relative expression levels of Smo, Gli-1, PI3 K, P-AKT, Bcl-2, Caspase-3, and Cyclin D1 protein after the intervention of jervine (5 μmol/L) and its combination with DAC (5 μmol/L) (n = 3). A. The Smo, Gli-1, PI3K, p-AKT, Bcl-2, and Cyclin D1 proteins are downregulated, and caspase-3 is upregulated after the treatment of jervine and DAC. B. Western blotting of Smo, Gli-1, PI3K, p-AKT, Bcl-2, Cyclin, and caspase-3 proteins indicating the differences among the different groups.
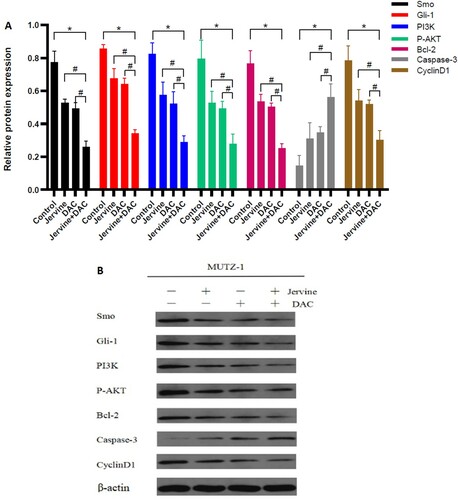
4. Discussion
Epigenetic changes are the hotspot and main entry point for studying the pathogenesis of MDS and exploring treatment methods. Among them, DNA methylation is the most common, which can change the stability of the genome and the expression of tumor suppressor genes, and this change can be reversed [Citation20]. In MDS patients, many essential tumor suppressor genes (P15, P16/CDKNA2, RASSFlA, FHIT, etc.) are abnormally hypermethylated in the CpG island DNA in their promoters, and their expression will be silent, which ultimately become an essential factor in the development of MDS [Citation21]. Therefore, HMAs have become one of the critical targets for the treatment of MDS. DAC is a natural analog of deoxycytidine adenosine, with specific sensitivity to the S phase of the cell cycle. Low-dose of DAC can specifically bind irreversibly with DNA methyltransferases (DNMTs), inhibit the activity of DNMTs, and reduce DNA methylation. Increasing expression levels or re-activation of silent tumor suppressor genes could promote tumor cell differentiation or apoptosis, and ultimately improve the condition of some patients with MDS, delay the transformation to AML, to achieve the goal of prolonging survival [Citation22,Citation23]. However, because DAC cannot completely block the synthesis of DNMTs, and the CR rate of MDS is low, continuous administration is generally required in clinical practice to maintain the drug's therapeutic effect, and researchers are trying to combine other drugs with improving its efficacy.
Studies have found that the Shh signaling pathway is involved in the self-renewal of stem cells. Excessive activation of the Shh pathway can induce normal stem cells to transform into cancer stem cells and promote AML and MDS-associated cancer stem cells [Citation24,Citation25]. Zou et al. [Citation11] performed Shh, Smo, and Gli-1 gene detection on bone marrow CD34+ cells in 32 MDS patients and 9 MDS-transformed AML patients. The study results showed that Shh signaling pathway activation exists in MDS patients, and there is a high risk of MDS and leukemia. The expression levels of Shh, Smo, and Gli-1 genes in high-risk patients were significantly higher than those in low-risk patients, and the above-mentioned gene expression levels were related to the prognostic group of IPSS. Xavier-Ferrucio et al. [Citation12] performed Ptch, Smo, Gli1, and Gli2 gene detection in the bone marrow CD34+ cells of 63 low-risk and high-risk MDS patients, and the results showed that Smo is highly expressed in MDS patients. Smo expression is associated with the classification system of WHO, and a higher expression level of Smo is significantly correlated with shortened Patients’ EFS and OS. Our research also confirmed the above view. Therefore, blocking this pathway may affect cancer stem cells’ development and survival, providing a novel option for MDS treatment.
The MUTZ-1 cells used in this study are tumor cells isolated and constructed from MDS-RAEB patients. The preliminary test results show that Shh signaling pathway-related genes are highly expressed in MUTZ-1 cells. Jervine and DAC single-drugs are effective against MUTZ- 1 cells. Both drugs have inhibitory effects on the cellular proliferation rate, and these effects are time-dependent and dose-dependent. The combination of Jervine and DAC at low concentrations can effectively inhibit the proliferation of MUTZ-1 cells, promote cell apoptosis, and block the G1 phase of the cell cycle. The combined drug effect is better than the single-agent group, indicating that the two-drug combination can synergistically inhibit the proliferation of MUTZ-1 cells and promotes apoptosis [Citation11]. Also, in this study, we found that the Jervine and DAC single-drug treatment and the combination of the two drugs can significantly reduce the expression levels of Smo, Gli-1, PI3K, p-AKT, Bcl2, and Cyclin D1 in MUTZ-1 cells, while the expression of Caspase-3 protein is upregulated. It is worth noting that the changes in the above-mentioned proteins in the combination group are more remarkable, which further shows that the combination therapy may have a more significant inhibitory effect on the Shh signaling pathway, and also significantly affect the activation of the PI3K/AKT signaling pathway and mediate downstream protein expression. Raoul Tibes et al. [Citation26] used RNAi interference technology to screen four types of myeloid cells (TF1, THP1, MDS-L, and HEL) for identifying differentially expressed genes. The results showed that the Shh signaling pathway might be an important signaling pathway for MDS and AML. After intervention with the above four cell lines and RNAi screening, the expression levels of Shh, Smo, and Gli-3 genes in the Shh signaling pathway were significantly down-regulated. After further processing 7 myeloid cell samples with Smo inhibitor combined with AZA, the results also showed that combining the two drugs could synergistically inhibit the Shh signaling pathway's expression and inhibit cell colony formation. Zou et al. [Citation18] silenced the expression of Gli-1 in MUTZ-1 cells by shRNA, and the results showed that the proportion of cells in the G0/G1 phase increased, and the rate of apoptosis also increased while silencing the expression of Gli-1 reduced the expression of DNMT1 in the cells. They found that the higher expression of tumor suppressor gene p15, and blocking of the Shh signaling pathway to treat MUTZ-1 cells is associated with inhibition of cell proliferation, reduced expression of DNMT1, and the higher expression of p15, indicating that the Gli-1 silencing is associated with the blocking of the Shh signaling pathway. It proves that the mechanism of Shh signaling downregulation may be associated with DNA hypomethylation of key regulatory genes. Our study results showed that the expression of PI3K and p-AKT was remarkably downregulated after the treatment of combined drugs (jervine and DAC). Decitabine is a cytosine analog inhibiting DNA methylation and has dual effects on neoplastic cells. Decitabine is associated with the reactivation of silenced genes and differentiation at low doses. On the other hand, decitabine is associated with cytotoxicity at high doses [Citation27]. In MDS, decitabine is a hypomethylating agent that is incorporated into the DNA and forms irreversible covalent adducts with DNA-methyltransferases [Citation28]. At lower concentrations of decitabine, it is considered to exert its anticancer effects by inducing DNA hypomethylation [Citation28]. In addition, decitabine is effective in the management of refractory or relapsed AML. Decitabine is used with chemotherapy or molecular therapy. However the efficacy of its combinational use is much variable [Citation29]. Decitabine in combination with chidamide, showing synergistic cytotoxicity in acute myeloid leukemia (AML) and myelodysplastic syndrome (MDS) [Citation30].
Collectively, our data indicating that there may be substantial crosstalk between the Shh signaling pathway and the PI3K/AKT signaling pathway in the MDS cells. The PI3K/AKT signaling pathway is one of the important signaling pathways in cells, involved in the regulation of cell proliferation, differentiation, and apoptosis [Citation31]. In high-risk MDS patients, there is continuous activation of the PI3K/AKT signaling pathway, which plays an important role in promoting the MDS cell proliferation and anti-apoptosis [Citation32,Citation33]. Several studies have shown that the activation of the Shh signaling pathway has a specific regulatory effect on the PI3K/AKT signaling pathway, and the combination of these two deregulated pathways can promote tumor formation [Citation34–36]. We investigated the deregulatory status of the expression levels of downstream proteins Bcl-2, Cyclin Dl, and Caspase-3 in this study. Therefore, through this study, we found that the Shh signaling pathway in MUTZ-1 cells is likely to mediate the expression of downstream proteins through changes in the P13 K/AKT pathway, thereby affecting the phenotypic standardization of cell proliferation and apoptosis. It will be warranted to further explore the possible molecular mechanisms of the crosstalk between the PI3K/AKT pathway and the SHH pathway in MDS patients.
5. Conclusions
In summary, the combination of Smo inhibitor jervine and DAC can synergistically inhibit the proliferation, arrest cell cycle, and promotes cellular apoptosis of MDS cells. The mechanism of action may be mediated by regulating the Shh signaling pathway, P13 K/AKT signaling pathway, and other key regulatory downstream proteins associated with MDS. Combining with the relevant clinical trial data of existing Smo inhibitors, the combination of Smo inhibitors and HMAs may provide a novel therapeutic intervention for high-risk MDS patients.
Authors’ contributions
All authors have contributed to the research concept, design, and interpretation of the data. All authors review and approved the final version of the document.
Disclosure statement
No potential conflict of interest was reported by the author(s).
Data availability
All data utilized, produce, and analyzed during this study are included in this article.
Additional information
Funding
References
- Cazzola M. Myelodysplastic syndromes. N Engl J Med. 2020;383(14):1358–1374. doi:https://doi.org/10.1056/NEJMra1904794.
- Greenberg PL, Stone RM, Al-Kali A, et al. Myelodysplastic Syndromes, version 2.2017, NCCN clinical practice guidelines in oncology. J Natl Compr Canc Netw. 2017;15(1):60–87. doi:https://doi.org/10.6004/jnccn.2017.0007.
- Joshi K, Zhang L, Breslin SJP, et al. Advances in experimental medicine and biology. Adv Exp Med Biol. 2019;1143:95–128. doi:https://doi.org/10.1007/978-981-13-7342-8_5.
- Komrokji RS. Treatment of higher-risk myelodysplastic syndromes after failure of Hypomethylating agents. Clin Lymphoma Myeloma Leuk. 2015;15 Suppl:S56–S59. doi:https://doi.org/10.1016/j.clml.2015.03.010.
- Flotho C, Sommer S, Lübbert M. DNA-Hypomethylating agents as Epigenetic therapy before and after allogeneic hematopoietic stem cell transplantation in myelodysplastic syndromes and Juvenile Myelomonocytic Leukemia. Semin Cancer Biol. 2018;51:68–79. doi:https://doi.org/10.1016/j.semcancer.2017.10.011.
- Prébet T, Gore SD, Esterni B, et al. Outcome of high-risk myelodysplastic syndrome after Azacitidine treatment failure. J Clin Oncol. 2011;29(24):3322–3327. doi:https://doi.org/10.1200/JCO.2011.35.8135.
- Lee SJ, Do I-G, Lee J, et al. Gastric Cancer (GC) patients with Hedgehog Pathway activation: PTCH1 and GLI2 as independent prognostic factors. Target Oncol. 2013;8(4):271–280. doi:https://doi.org/10.1007/s11523-013-0253-1.
- Li H, Yue D, Jin JQ, et al. Gli promotes Epithelial-Mesenchymal Transition in human lung adenocarcinomas. Oncotarget. 2016;7(49):80415–80425. doi:https://doi.org/10.18632/oncotarget.11246.
- Terao T, Minami Y. Targeting Hedgehog (Hh) pathway for the Acute Myeloid Leukemia treatment. Cells. 2019;8(4):312, doi:https://doi.org/10.3390/cells8040312.
- Qin Y, Jiang M, Tuerxung N, et al. Sonic Hedgehog signaling pathway in myelodysplastic Syndrome: Abnormal activation and Jervine intervention. Gene. 2020;754:144881, doi:https://doi.org/10.1016/j.gene.2020.144881.
- Zou J, Hong Y, Tong Y, et al. Sonic Hedgehog produced by Bone Marrow-Derived Mesenchymal stromal cells supports cell survival in myelodysplastic syndrome. Stem Cells Int 2015. 2015: 957502, doi:https://doi.org/10.1155/2015/957502.
- Xavier-Ferrucio JM, Pericole FV, Lopes MR, et al. Abnormal Hedgehog pathway in myelodysplastic syndrome and Its impact on Patients’ outcome. Haematologica. 2015;100(12):e491–e493. doi:https://doi.org/10.3324/haematol.2015.124040.
- Szeliga M, Ciura J, Tyrka M. Representational difference analysis of transcripts involved in Jervine biosynthesis. Life (Basel). 2020;10; doi:https://doi.org/10.3390/life10060088.
- Irvine DA, Copland M. Targeting Hedgehog in hematologic malignancy. Blood. 2012;119(10):2196–2204. doi:https://doi.org/10.1182/blood-2011-10-383752.
- Hoy SM. Glasdegib: first global approval. Drugs. 2019;79(2):207–213. doi:https://doi.org/10.1007/s40265-018-1047-7.
- Cortes JE, Heidel FH, Hellmann A, et al. Randomized comparison of low dose Cytarabine with or without Glasdegib in patients with newly diagnosed Acute Myeloid Leukemia or high-risk myelodysplastic syndrome. Leukemia. 2019;33(2):379–389. doi:https://doi.org/10.1038/s41375-018-0312-9.
- Zeidan AM, Schuster MW, Krauter J, et al. Clinical benefit of Glasdegib in combination with Azacitidine or low-dose Cytarabine in patients with Acute Myeloid Leukemia. Blood. 2019;134:3916–3916. doi:https://doi.org/10.1182/blood-2019-124034.
- Zou J, Zhou Z, Wan L, et al. Targeting the Sonic Hedgehog-Gli1 pathway as a potential new therapeutic strategy for myelodysplastic syndromes. PLoS One. 2015;10:e0136843, doi:https://doi.org/10.1371/journal.pone.0136843.
- Rimkus TK, Carpenter RL, Qasem S, et al. Targeting the Sonic Hedgehog signaling pathway: review of Smoothened and GLI inhibitors. Cancers. 2016;8(2):22, doi:https://doi.org/10.3390/cancers8020022.
- Will B, Zhou L, Vogler TO, et al. Stem and progenitor cells in myelodysplastic syndromes show aberrant stage-specific expansion and harbor genetic and Epigenetic alterations. Blood. 2012;120(10):2076–2086. doi:https://doi.org/10.1182/blood-2011-12-399683.
- Khan H, Vale C, Bhagat T, et al. Role of DNA methylation in the pathogenesis and treatment of myelodysplastic syndromes. Semin Hematol. 2013;50(1):16–37. doi:https://doi.org/10.1053/j.seminhematol.2013.01.001.
- Hackanson B, Daskalakis M. Decitabine. Recent Results Cancer Res. 2014;201:269–297. doi:https://doi.org/10.1007/978-3-642-54490-3_18.
- Ganetsky A. The Role of Decitabine for the treatment of Acute Myeloid leukemia. Ann Pharmacother. 2012;46(11):1511–1517. doi:https://doi.org/10.1345/aph.1R151.
- Li Z, Mao S, Jin J. Activation of Hedgehog pathway in Acute Myeloid Leukemia patients. Int J Clin Exp Pathol. 2017;10:8605–8609.
- Merchant AA, Matsui W. Targeting hedgehog–a Cancer stem cell pathway. Clin Cancer Res. 2010;16(12):3130–3140. doi:https://doi.org/10.1158/1078-0432.CCR-09-2846.
- Tibes R, Al-Kali A, Oliver GR, et al. The Hedgehog pathway as targetable vulnerability with 5-Azacytidine in myelodysplastic syndrome and Acute Myeloid Leukemia. J Hematol Oncol. 2015;8(1):114, doi:https://doi.org/10.1186/s13045-015-0211-8.
- Jabbour E, Kantarjian HM, Garcia-Manero G, et al. Decitabine in myelodysplastic syndromes. Therapy. 2005;2:835–842. doi:https://doi.org/10.2217/14750708.2.6.835.
- Atallah E, Kantarjian H, Garcia-Manero G. The role of Decitabine in the treatment of myelodysplastic syndromes. Expert Opin Pharmacother. 2007;8(1):65–73. doi:https://doi.org/10.1517/14656566.8.1.65.
- Ma Y-Y, Zhao M, Liu Y, et al. Use of decitabine for patients with refractory or relapsed Acute Myeloid Leukemia: A systematic review and meta-analysis. Hematology. 2019;24(1):507–515. doi:https://doi.org/10.1080/16078454.2019.1632407.
- Mao J, Li S, Zhao H, et al. Effects of chidamide and Its combination with decitabine on proliferation and apoptosis of Leukemia cell lines. Am J Transl Res. 2018;10:2567–2578.
- Dey N, De P, Leyland-Jones B. PI3K-AKT-MTOR inhibitors in breast cancers: from tumor cell signaling to clinical trials. Pharmacol Ther. 2017;175:91–106. doi:https://doi.org/10.1016/j.pharmthera.2017.02.037.
- Lin S, Li J, Zhou W, et al. BIIB021, an Hsp90 inhibitor, effectively kills a myelodysplastic syndrome Cell Line via the activation of caspases and inhibition of PI3K/Akt and NF-ΚB pathway proteins. Exp Ther Med. 2014;7:1539–1544. doi:https://doi.org/10.3892/etm.2014.1651.
- Yin J, Li X, Zhang Z, et al. SPAG6 silencing induces apoptosis in the myelodysplastic syndrome Cell Line SKM-1 via the PTEN/PI3 K/AKT signaling pathway in vitro and in vivo. Int J Oncol. 2018;53:297–306. doi:https://doi.org/10.3892/ijo.2018.4390.
- Riobó NA, Lu K, Ai X, et al. Phosphoinositide 3-Kinase and Akt are essential for Sonic Hedgehog signaling. Proc Natl Acad Sci U S A. 2006;103(12):4505–4510. doi:https://doi.org/10.1073/pnas.0504337103.
- Yoo YA, Kang MH, Lee HJ, et al. Sonic Hedgehog pathway Promotes Metastasis and Lymphangiogenesis via activation of Akt, EMT, and MMP-9 pathway in Gastric cancer. Cancer Res. 2011;71(22):7061–7070. doi:https://doi.org/10.1158/0008-5472.CAN-11-1338.
- Ke Z, Caiping S, Qing Z, et al. Sonic Hedgehog-Gli1 signals promote Epithelial-Mesenchymal Transition in ovarian Cancer by mediating PI3K/AKT pathway. Med Oncol. 2015;32:368. doi:https://doi.org/10.1007/s12032-014-0368-y.