ABSTRACT
Bone marrow microenvironment provides critical regulatory signals for lineage differentiation and maintenance of HSC quiescence, and these signals also contribute to hematological myeloid malignancies. Macrophages exhibit high phenotypic heterogeneity under both physiological and pathological conditions and are mainly divided into proinflammatory M1 and anti-inflammatory M2 macrophages. Furthermore, osteoclasts are multinucleated giant cells that arise by fusion of monocyte/macrophage-like cells, which are commonly known as bone macrophages. Emerging evidence suggests that macrophages and osteoclasts originating from myeloid progenitors lead to two competing differentiation outcomes, and they appear to play an important role in the onset, progression, and bone metastasis of solid cancers. However, little is known about their role in the development of hematological malignancies. In this review, we focus on macrophages and osteoclasts, their role in leukemia, and the potential for targeting these cells in this disease.
KEYWORDS:
Introduction
Hematopoietic stem cells (HSCs) are a group of primitive hematopoietic cells residing in the bone marrow (BM) that have long-term self-renewal ability and have the potential to differentiate into various kinds of mature blood cells[Citation1,Citation2]. The maintenance of ‘stemness’ in HSCs is regulated by a specialized BM microenvironment (BMME)[Citation3]. In recent years, studies have revealed the existence of a complex interaction network among bone marrow cells in the microenvironment, which can regulate the proliferation, differentiation, localization and self-renewal of HSCs and is crucial for maintaining the stability of the intracellular environment and blood regeneration[Citation4,Citation5]. However, abnormal BMME can lead to hematopoietic dysfunction and even bone marrow malignancies such as leukemia[Citation6–9]. Increasing evidence has shown that leukemia cells can transform the normal BMME into a leukemic microenvironment to better support the survival and proliferation of tumor cells[Citation10–12]. More importantly, the leukemia microenvironment protects leukemia cells against chemotherapy-induced death and develops drug resistance[Citation13,Citation14]. In particular, the role of the mononuclear phagocytic system of the BM has been implicated in both leukemia chemoresistance and progression[Citation15,Citation16]. In addition, osteoclasts (OCs) are terminally differentiated cells of the monocyte/macrophage lineage that resorb mineralized bone matrix and degrade the extracellular matrix of the endosteum in trabecular bone. Recently, osteolineage cells, mainly including both osteoblasts (OBs) and OCs, have been reported to be involved in regulating hematopoiesis in the BM niche while an altered osteogenic niche has been reported to lead to myeloid leukemia in BM[Citation17,Citation18].
Understanding of underlying niche-imposed support to leukemic cells will contribute to develop effective drug therapies. This review discusses the role of different niche cells and provides an overview of emerging therapeutic strategies for leukemia.
Relationship between macrophages and osteoclasts
The mononuclear phagocytic system was proposed in 1972 to consist of monocytes, macrophages, dendritic cells (DCs) and their precursor cells. Cells of the mononuclear phagocyte series have similar characteristics in terms of morphology, function, origin, and kinetics of phagocytes[Citation18]. Macrophages and osteoclasts are the two competing differentiation outcomes from myeloid progenitors[Citation19,Citation20]. These two types of polarized cells have been separately reported to have effects on the progression of leukemia cells[Citation16,Citation17]. Due to the complexity of their functions, the dynamic regulation among macrophages, osteoclasts, and leukemia cells is unclear. Here, we detail the biology of monocytes/macrophages and osteoclasts in the context of myeloid malignancy.
Characteristics of macrophages
Macrophages are heterogeneous cell populations originating from monocytes, and they exist in almost all tissues and play crucial roles in the maintenance of tissue homeostasis[Citation21]. In response to different stimuli, macrophages therefore have great plasticity and can differentiate into several functional states[Citation22,Citation23]. Typically, macrophages can be divided into two major phenotypes, M1 and M2. M1 macrophages are classically activated macrophages by cytokines, such as interferon γ (IFN γ), tumor necrosis factor-α (TNF-α), and lipopolysaccharides (LPSs). M1 macrophages are involved in the host defense against different pathogens and play a role in antitumor immunity by the production of numerous inflammatory mediators, such as interleukin 6 (IL-6), reactive oxygen species (ROS) and nitric oxide (NO). In contrast, M2 macrophages, known as alternatively activated macrophages, are stimulated by interleukin-4 (IL-4), or interleukin 13 (IL-13) and associated with anti-inflammatory responses. They secrete transforming growth factor-β (TGFβ), interleukin 10 (IL-10), arginase, and other cytokines that cause immune suppression, angiogenesis and tissue repair. However, most macrophages are polarized in a signal-dependent manner to a continuum of states between M1 and M2 rather than either the M1 or M2 phenotype in vivo[Citation24]. The origin and characterization of three other categories of macrophages remain unclear, including tumor-associated macrophages (TAMs), CD169+ macrophages, and TCR+ macrophages[Citation24]. These macrophage subpopulations have been involved as main players in several human pathologies, such as cancer, infectious diseases, obesity, and asthma [Citation22–24].
Origin and function of osteoclasts
The monocyte/macrophage origin of osteoclasts was confirmed by Udagawa et al. in 1990[Citation25], and they showed that hematopoietic stem cells produce the monocyte-macrophage lineage when stimulated by macrophage colony-stimulating factor (M-CSF). Subsequently, osteoclasts were confirmed to be generated from precursors of the monocyte-macrophage lineage and from mature tissue macrophages. It is now well established that osteoclasts are multinucleated giant cells that arise by fusion of monocyte/macrophage-like cells in the presence of CSF-1 and receptor activator of nuclear factor-kB (RANK) and its ligand (RANKL), and they are commonly known as bone macrophages[Citation26,Citation27]. Moreover, some studies have reported the transdifferentiation of immature dendritic cells (DCs) into osteoclasts[Citation26,Citation28]. These studies have pointed out that immature DCs can also form osteoclasts under the influence of M-CSF and RANKL, mainly in inflammatory bone loss. However, the physiological relevance of DC-derived osteoclasts is not fully understood.
Osteoclasts play an important role in the balance of bone metabolism under physiological or pathological conditions, and are the only bone resorptive cells in the human body. Bone formation by osteoblasts and bone resorption by osteoclasts occur mainly at the bone surface and are tightly coupled to maintain bone homeostasis[Citation29]. Osteoclast hyperactivation is common in malignant bone tumors, osteoporosis, rheumatoid arthritis (RA) and other bone metabolic diseases[Citation30,Citation31]. On the other hand, dysregulated bone homeostasis generally leads to excessive bone apposition and osteopetrosis[Citation31,Citation32]. Although it was largely considered that osteoclasts only function in bone resorption, it becomes increasingly clear that osteoclasts are involved in multiple pathways beyond bone homeostasis[Citation32]. There are many similarities between osteoclasts and macrophages in terms of cell origin, spatial distribution, function and regulation. At present, it is believed that osteoclasts are innate immune cells residing in bones. Innate immune cells in the tumor microenvironment, such as macrophages, dendritic cells, and mast cells, are often transformed by tumor cells and recruited as accomplices of tumor cells[Citation20,Citation29]. The delicate relationship between osteoclasts and the immune system provides a new perspective on the role of a large number of activated osteoclasts in tumors.
Interaction between macrophages and osteoclasts
Both osteoclasts and macrophages originate from the monocyte precursor cells of hematopoietic stem cells; second, both have tissue degradation function. Finally, osteoclasts express some immune molecules on the surface, which is similar to that of macrophages. Therefore, osteoclasts are currently considered innate immune cells residing in bones.
Bone-resident tissue macrophages are termed osteal macrophages which are predominantly located adjacent to osteoblasts and may support osteoblastogenesis and bone formation[Citation33]. M1 macrophage-related cytokines, such as TNF-α, IL-6, and IL-1β, can induce osteoclastogenesis, while M2 macrophage-related cytokines, such as IL-4 and IL-10, can inhibit osteoclastogenesis through the inhibition of NFATc1. Thus, the polarization of macrophages (M1/M2) is important for the determination of osteoclastogenesis, which makes the interaction between macrophages and osteoclasts even more complex[Citation18,Citation33].
Yang et al. (2019) summarized the recently discovered molecular determinants for the polarization of macrophages and osteoclasts. These determinants include nuclear receptors and transcription factors, such as peroxisome proliferator activated receptor γ (PPARγ), estrogen-related receptor α (ERRα) and PPARγ coactivator 1-β (PGC-1β) [Citation34], and they act as important modulators to promote osteoclast differentiation but suppress the activation of M1 macrophages. Although the interaction between macrophages and osteoclasts has been realized for a long time, the underlying molecular mechanisms are not well understood.
Macrophage and osteoclast link in leukemia
HSCs reside in specific BM microenvironments and compose two predominant niches: the perivascular niche and endosteal niche[Citation2,Citation35]. Both of these niches induce different responses in HSCs, such as self-renewal, quiescence, homing, mobilization, or differentiation. Dysfunction in these microenvironments may lead to abnormal hematopoiesis and tumorigenesis. Leukemic cells have been suggested to interact dynamically and closely with the BMME. It is believed that BMME plays a key role in the maintenance, initiation, or outcome of hematological malignancies[Citation10]. Signals from the environment contribute to protection of leukemic cells against apoptosis and promote their survival or proliferation[Citation36]. Moreover, leukemic cells may influence the BM niche and disrupt normal hematopoiesis. Here, we focus on the role of monocytes/macrophages and osteoclasts in the context of myeloid malignancy.
Macrophages and leukemia
TAMs represent an important inflammatory response cell in the tumor microenvironment (TME), and they are highly plastic and play an important role in the regulation of immune function in tumor tissues[Citation37–39]. TAMs have different phenotypes at different stages of tumor development. In most advanced solid tumors, TAMs are the largest group of infiltrating leukocytes. Extensive TAMs infiltration is associated with poor prognosis in a variety of cancers. TAMs affect almost every aspect of tumor biology, promoting angiogenesis, tumor progression, invasion, and metastasis, and this effect on different stages of tumor development highlights their functional diversity[Citation39]. In the early stage of tumor development, macrophages exhibit an antitumor M1 phenotype due to activated leukocytes producing granulocyte-macrophage colony stimulating factor and interferon γ. Subsequent tumor growth causes monocytes and macrophages to produce signals for tissue repair, resulting in M2-type TAMs with repair functions. TAMs may also have both M1-type and M2-type characteristics. However, the signaling mechanism of TAMs to obtain mixed M1/M2 phenotypes in the TME is still unclear and TAMs cannot always be divided into pure M1 or pure M2 phenotypes[Citation24]. TAMs are involved in tumor-associated inflammatory responses and express a variety of biomarkers that may be potential targets for cancer diagnosis and treatment.
Recent studies have found that TAMs also have a significant impact on leukemia, including AML, chronic lymphocytic leukemia (CLL) and ALL, and can promote the survival, growth and drug resistance of leukemia cells[Citation40–42]. Yahya et al. (2016) found that the proportion of CD163+CD206+M2-like macrophages in the bone marrow of AML patients was significantly higher than that of healthy volunteers. Further studies have confirmed that there are a large number of GFP-CD11b+ Ly6G- macrophages, namely AML-associated macrophages (AAMs), in the bone marrow and spleen of AML mice[Citation40]. AML cells can induce the polarization of BMDMs into AAMs, which mainly exhibit the characteristics of M2-like macrophages, and AAMs derived from AML mice can significantly promote the proliferation of the AML cell line C1498. In addition, TAMs not only promote the immune escape of AML cells through the binding of SIRT1 to the CD47 receptor on the surface of AML cells but also regulate the self-renewal, survival, proliferation and resistance of leukemia stem cells (LSCs).
Studies have reported on leukemia-associated macrophages (LAMs) infiltration in the Notch 1 knockout ALL mouse model, including different parts of the spleen, bone marrow, and liver[Citation43]. Although the LAMs of each part have different gene expression characteristics, they can all promote the proliferation and migration ability of leukemia cells. Chen et al. (Citation2017) further found that peritoneal LAMs expressed both M1- and M2-associated genes, which were also detected in BM and spleen LAMs[Citation44]. These LAMs showed common features when compared with TAMs and can also promote the proliferation of leukemia cells.
A type of nurse-like cells (NLCs) is observed in patients with CLL, which is a special type of TAMs in CLL, that can protect CLL cells by secreting a variety of cytokines, promote their survival in vitro, and inhibit drug-induced apoptosis[Citation41]. Through the chemokines CXCL12, CXCL13 and vascular cell adhesion molecule 1 (VCAM1), TAMs may promote the proliferation of CLL cells. CD14+ cells are able to differentiate into CLL-NLCs when in contact with CLL cells. These cells express very high amounts of CD163 and CD68 and are able to protect CLL cells from apoptosis. However, CD14+ cells derived from healthy donors (HD-NLCs) express very little CD163 and CD68, do not produce CCL4 and are unable to protect CCL cells. This study reveals that CLL-NLCs are the specific TAMs of CLL and are able to rescue CLL cells compared to HD-NLCs[Citation45].
Other hematological tumors, such as multiple myeloma and classic Hodgkin’s lymphoma, both have a large number of infiltrated macrophages[Citation46,Citation47]. At present, there are few studies on the role of macrophages in leukemia, and more studies are needed in the future.
OCs and leukemia
Over the years, leukemia is considered to be a series of genetic attacks on hematopoietic stem/progenitor cells (HSPCs), which convert them into preleukemic and eventually into leukemic cells. Recent findings suggest that genetic changes in niche cells, particularly in osteogenic cells, may also result in leukemia in a mouse model[Citation17,Citation48]. The osteogenic niche is mainly composed of OBs, osteocytes and OCs, and plays a key role in the maintenance and expansion of HSPCs as well as in their transformation into leukemia cells. Bone is one of most common sites for metastasis, often leading to mortality. Matrix metalloproteinases (MMPs) expressed at the interface between tumor and stromal cells play an important role in osteolysis and dissemination into bone tissue. MMP-7 expressed by osteoclasts at the tumor-bone interface triggers osteolysis and subsequent bone metastasis in a rodent model of prostate cancer[Citation49]. An increasing number of studies have proven that bone is also an immune organ with important immune regulation functions. Bone not only provides the structural framework of the body but also acts as a reservoir for hematopoietic cells and immune cells[Citation31,Citation48]. Hematopoietic cells, immune cells, osteoblasts, osteoclasts and other cells share the BMME. In recent years, a better understanding has been reached on the interaction mechanism between bone and the immune system, and a new cross-disciplinary osteoimmunology has been developed. Bone immunological studies have shown that T cells play an important role in the mediation of osteoclasts. On the one hand, activated T lymphocytes are an important source of RANKL and IL-1β, which are important activating factors for osteoclasts, thereby activating osteoclasts, which has also been observed in rheumatoid arthritis; on the other hand, activated T lymphocytes can also secrete IFN-γ, IL-4, IL-10 and other cytokines to inhibit osteoclasts[Citation31]. Therefore, normally functioning T cells play an important role in regulating the balance of bone metabolism.
An G et al. (2016) showed that the number and activity of OCs are strongly enhanced by myeloma cells, leading to significant bone lesions in patients with multiple myeloma (MM)[Citation50]. OCs significantly protect MM cells against T-cell–mediated cytotoxicity via direct inhibition of proliferating CD4+ and CD8+ T cells. Inhibitory immune molecules programmed death ligand 1 (PD-L1), galectin-9, herpesvirus entry mediator (HVEM), and CD200 as well as T-cell metabolism regulators indoleamine 2,3-dioxygenase (IDO), and CD38 are significantly upregulated during osteoclastogenesis. Recent studies have also demonstrated that OCs express a number of immune receptors and share phenotypic features with macrophages, such as a high number of lysosomes. Autophagy is an evolutionarily highly conservative lysosomal pathway involved in oncogenesis and resistance in leukemia[Citation51]. Recent studies suggest that inhibiting autophagy may be an efficient approach to improve the chemotherapeutic antileukemic regimens. Through suppressing autophagy in ETV6-RXNX1 gene positive B-ALL could severely downregulate cell proliferation and survival[Citation52]. For example, an autophagy inhibitor, choloroquine, could increase the response of patients with B-ALL to the chemotherapy[Citation53]. Furthermore, hydroxychloroquine, an autophagy inhibitor, reduces proliferation and survival of leukemic blasts in ALL[Citation54]. Targeting suppressing autophagy in T-ALL was also effective and could be used to support further clinical trials in the future[Citation52]. However, how secretory lysosomes in osteoclasts work still needs to be clarified. TAMs in the MM microenvironment protect MM cells from chemotherapy-induced apoptosis. Based on these observations, they confirmed that OCs in the MM microenvironment play a similar role as TAMs[Citation50,Citation55,Citation56]. Importantly, they also showed that an anti-CD38 mAb can restore the proliferative activity of CD4 and CD8 T cells partially by downregulating the expression of HVEM and IDO in OCs. These results further support targeting these checkpoint molecules may restore anti-MM immunity and enhance immunotherapeutic activity. Furthermore, their team demonstrated that high expression of NEK2, one of the chromosomal instability (CIN) genes, is associated with drug resistance and poor prognosis. NEK2 can induce osteoclast differentiation and bone destruction via heparanase in MM, again emphasizing the importance of OC in MM[Citation56].
A greater number of studies have focused on osteoclasts in MM than on osteoclasts in leukemia, and they are mainly concentrated in chronic lymphocytic leukemia. Recently, OCs have been shown to play a key role in the mobilization of hematopoietic stem and progenitor cells[Citation17]. It appears that OCs are involved in the maintenance of dormant leukemic cells in the BMME through the release of TGF-β[Citation48]. Quiescence is important for the survival of leukemia-initiating cells (LICs) or LSCs, which may be the cause of drug resistance and could become effective targets for the treatment of leukemia. RANK, osteoprotegerin (OPG) and their ligand (RANKL) are key regulators of bone remodeling. RANKL, a specific osteoclast differentiation factor, is a type II transmembrane protein of the TNF superfamily, with its N-terminus constituting the intracellular region and C-terminus containing the receptor-binding domain, which may further influence the progression of B cell-derived malignancies such as CLL or MM[Citation50,Citation56,Citation57]. In CLL, RANKL mediates the release of IL-8, which contributes to disease pathophysiology. In MM, the balance of RANKL and OPG is disrupted, thus causing activation of osteoclasts and bone destruction, and RANKL neutralization delays MM progression in mice. Signaling via RANKL into MM and CLL cells induced the release of cytokines involved in disease pathophysiology. The effects of RANKL on osteoclastogenesis and cytokine production by malignant cells could be blocked by disruption of the RANK-RANKL interaction via denosumab.
Adult T-cell leukemia/lymphoma (ATL) is etiologically linked to infection with human T-cell leukemia/lymphoma virus type 1 (HTLV-I). One of the most serious and frequent complications arising in ATL patients is hypercalcemia as a result of increased osteolytic bone lesions. Recent studies suggest that leukemic cells from ATL patients overexpress Wnt5a, RANKL, and PTHLH. Among these pro-osteoclastic genes, Wnt5a secreted by ATL cells can enhance osteoclastogenesis and may contribute to osteolytic bone lesions and hypercalcemia, and this effect could be prevented by the addition of Wnt5a antibodies[Citation58].
B cell acute lymphoblastic leukemia (B-ALL) is the most common cancer in children and also occurs in adults. Leukemia-associated bone destruction is a significant source of acute and long-term morbidity in children and adolescents. Moreover, RANKL-RANK interactions play an important role in early B cell development as well in the progression of hematological malignancies[Citation59]. Sujeetha et al. demonstrated that leukemic cells from a spontaneous mouse model of B-ALL are positive for RANKL, suggesting an underlying mechanism for fragile bones. Similarly, in multiple subtypes of patient-derived xenograft (PDX) models, B-ALL cells can produce high levels of RANKL in BMME where osteoclasts are localized, thereby promoting osteoclast differentiation and driving the destruction of trabecular bone and long bone growth plates.
Acute myeloid leukemia (AML) is one of the most aggressive hematologic malignancies and is characterized by an increased number of myeloid precursors in BM that fail to differentiate into mature myeloid cells. Numerous AML studies have emphasized the relationship between leukemic cells and niches[Citation11–13]. AML cells have been confirmed to alter BM niches, and alterations in BM niches lead to the progression of leukemia. BM mesenchymal stromal cells (BMSCs) have been identified as essential HSC niche components. BMSCs represent a group of heterogeneous cell populations with self-renewal ability that exist in a large number of highly vascularized areas and highly express CXCL12, SCF, IL7, Wnts, OPN and other proteins[Citation14,Citation60]. They have the role of supporting and regulating hematopoiesis both in vivo and in vitro, can be distributed in a variety of tissues and organs in the body and have the potential to differentiate into osteoblasts, fibroblasts, reticulocytes, adipocytes and endothelial cells. Previously, several studies have shown abnormal differentiation in BMSCs of patients with myeloid malignancies accompanied by altered osteogenic and osteolytic activity. Frisch et al. reported that the function of osteoblastic cells is inhibited in myeloid leukemia[Citation11]. The reduced bone mineralization could have resulted from increased osteolytic activity. A short-lived increase in osteoclasts was also found in a murine model of the blast-crisis chronic myeloid leukemia (CML) phenotype[Citation61]. These reports suggest that leukemic cells remodel the endosteal bone marrow niche into a self-reinforcing leukemic niche. However, the extent to which osteoblasts and osteoclasts work together to reconstruct a hospitable microenvironment for leukemic cells needs further investigation.
Target of macrophages and osteoclasts for leukemia therapy
As mentioned above, the BMME promotes the survival, differentiation, proliferation and migration of HSPCs and their offspring[Citation14]. However, this microenvironment is not only a shelter for normal hematopoiesis, but also provides a shelter for the proliferation of LSCs. It also protects malignant cells from chemotherapy. Blocking the interaction between leukemia cells and their environment, may prevent chemotherapy resistance and drug side effects, and provide a new direction for the treatment of leukemia.
Targeting TAMs
Recently, the protection of leukemia cells by macrophages has been a hot research topic. TAMs play an important role in the tumor microenvironment. An increasing number of in vivo and in vitro experiments have confirmed that targeted TAMs can inhibit tumor cell activity, promote tumor cell death, and delay disease progression[Citation37–39]. Antitumor strategies targeting TAMs are gradually increasing. For example, targeted knockout of hypoxia-inducible factors can inhibit macrophage activity and recruitment[Citation62] and inducing TAM polarization to the M1 type can enhance the phagocytic function of CLL cells[Citation63]. The function of TAMs depends on the receptor tyrosine kinase CSF1R, which is a member of the type III receptor tyrosine kinase family and is activated by binding to its ligand CSF-1. CSF-1R is mainly expressed on monocytes and tissue macrophages and plays a key role in the proliferation, differentiation and chemotaxis of TAMs[Citation64,Citation65]. The CSF-1/CSF-1R signaling pathway plays an important role between leukemia cells and macrophages. Inhibiting the activation of CSF-1R can significantly reduce the number of TAMs in animals and significantly improve the prognosis of leukemia[Citation40,Citation41,Citation16]. Experimental results show that the specific CSF-1R inhibitor GW2580 or ARRY-382 can act on CD14+ monocytes in the CLL microenvironment and reduce the latter’s support for leukemia cells, and the combined application of GW2580 or ARRY-382 and ibrutinib can destroy the activation of B cell receptor signals in leukemia cells and has a synergistic antitumor effect. The use of CSF-1R inhibitors in AML patients can inhibit the secretion of multiple cytokines in support cells and block their protective effects on AML cells. Similarly, our research also shows that targeting macrophages can delay the progression of ALL in mice[Citation42]. Therefore, targeting CSF-1R combined with chemotherapeutic drugs can simultaneously kill leukemia cells, destroy the bone marrow microenvironment that they depend on for survival and enhance the antitumor effect. In-depth research on TAMs may open up new directions for the treatment of leukemia.
In addition to CSF1R inhibitors, another important target is CD47, a key ‘don’t eat me’ molecule that is upregulated by a variety of cancers[Citation66,Citation67]. CD47 renders malignant cells resistant to classic immune surveillance machinery and is now recognized as a fundamental driver of tumor growth. Under normal physiological conditions, the CD47 protein exists on the surface of many healthy cells. By combining with SIRPα (signal regulatory protein-α) on the surface of macrophages, CD47 tells macrophages not to ‘eat’ them, thereby protecting healthy cells from being eliminated. When cells age or become diseased, CD47 is gradually lost on the cell surface, and macrophages can recognize and process senescent or diseased cells. Unfortunately, many tumor cells also overexpress CD47; subsequently, TAMs not only coexist peacefully with tumor cells but also promote the proliferation of blood vessels in tumors, inhibit effector T cells, and promote tumor cell expansion and growth. At present, there are two main strategies for targeting the CD47-SIRPα pathway: via the CD47 antibody or via the SIRPα-Fc recombinant protein. Both methods have been validated by preclinical efficacy[Citation68]. Tumor cells can promote the migration of MDSCs and macrophages to the tumor area by expressing chemokines CCL2, CCL5, CXCL12, etc. By blocking these recruitment pathways, the number of MDSCs and tumor-promoting macrophages in the tumor infiltration area can be reduced (). However, whether targeting these pathways is useful for hematological tumors needs more research.
Targeting OCs
Few studies have focused on osteoclasts in leukemia. Numerous studies have characterized an immunosuppressive role of OCs in MM by upregulating various inhibitory checkpoint molecules and immune-suppressive cytokines[Citation50,Citation55,Citation56]. Moreover, CD38 is significantly upregulated during osteoclastogenesis in MM and targeting with an anti-CD38 mAb alleviates the immunosuppressive function of OCs, thereby improving anti-MM immunity. Whether this underlying mechanism is also present in leukemia needs further study.
Accordingly, the present data suggest that RANK/RANKL loop activation promoted by either leukemic cells or MM cells might extend its role to represent a factor enhancing clonal proliferating potential[Citation17,Citation69,Citation70]. Secchiero et al. (Citation2006) reported that the RANK/RANKL interaction potently promoted clone proliferation and resistance to apoptotic signals in CLL and that CLL cell proliferation was impaired by denosumab-mediated disruption of the RANK/RANKL loop[Citation71]. Antagonizing RANKL with denosumab markedly reduced the number of osteoclasts and attenuated femur bone erosion, thus emphasizing the role of the RANK/RANKL axis in osteoclast activation. Moreover, RANKL is the target of MMP-7[Citation49]. The MMPs represent the most prominent family of proteinases associated with tumorigenesis[Citation49]. Research on drugs called small-molecule metalloproteinase inhibitor (MPI) were started about three decades ago and markedly advanced our understanding of MMPs as modulators of the tumor microenvironment[Citation72]. Due to its complexity, we are only beginning to understand functions of MMPs and that further studies are required to evaluate the role of MPI under in vivo conditions.
Giannakoulas et al. (Citation2011) reported on a patient with AML who presented with hypercalcemia due to high bone turnover at diagnosis and confirmed that increased bone resorption is at least partially due to increased RANKL and IL-6 levels[Citation73]. Abnormalities in the RANKL/OPG pathway remained after antileukemia therapy. Unfortunately, further studies have not focused on the pathway during the progression of leukemia.
Previous research documented that OCs might contribute to the maintenance of quiescent leukemia stem cells by releasing TGF-β to the BM niche ()[Citation48]. Zoledronic acid (ZOL), a third-generation bisphosphonate, has been used as an adjuvant treatment for treating advanced cancers with bone metastases and has been well tolerated when administered to children with ALL-induced osteonecrosis[Citation74–76]. ZOL has previously been reported to exert antitumor effects in a CML mouse model by reducing the number of mature OCs in the BMME. Collectively, ZOL suppresses the progression of leukemia not only by directly inducing apoptosis in leukemic cells but also by inhibiting the activity of osteoclasts and restoring bone loss. These findings indicate that the interaction between leukemic cells and the microenvironment might play a significant role in the development of leukemia and that the interaction may represent a novel therapeutic target. Further studies evaluating the efficacy of ZOL in combination with standard chemotherapy for leukemia are now warranted ( and ).
Figure 1. Interaction between leukemic cells and macrophages in the microenvironment (A) Secreted factors by leukemic cells can recruit and polarize monocytes/macrophages toward a M2 pro-tumoral phenotype promoting tumor growth. (B) Interference with TAM Survival or inhibition of macrophage recruitment. Clodronate liposomes (Clod. Lip.) can selectively inhibit proliferation, migration and invasion of macrophages. Peripheral monocytes are recruited to tumor sites via tumor derived chemokines CCL2, CCL5 and CSF1. Targeting these signaling molecules can potentially inhibit the accumulation of TAM in the microenvironment, thereby improving treatment efficiency.
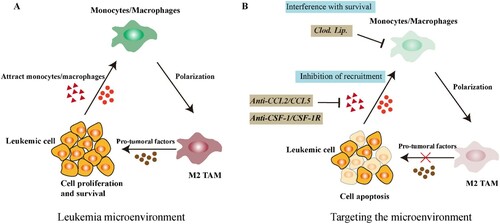
Figure 2. Schematic representations of mechanisms of interactions between tumor cells and OCs in leukemia and myeloma. Tumor cells overexpressed the osteolytic-associated genes-Wnt5a, PTHLH, M-CSF, RANKL and NEK2, etc. These molecules favor osteoclast differentiation and promote the progression of tumor. When targeted by specific antibody can block the interaction between tumor cells and OCs and improve therapy outcome.
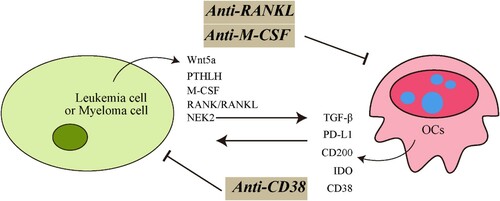
Conclusions
The BM microenvironment includes a variety of cells with special functions as well as an extracellular matrix, growth factors and chemokines. The structure and function are very complex. Major research progress in recent years has revealed the important role of different components in the bone marrow microenvironment in the pathogenesis of bone marrow malignant tumors, and clarified that they interact with normal HSCs or LSCs in different ways. However, the potential mechanism of BMME is still in the preliminary research stage, and its complexity is becoming increasingly obvious due to different effects in different diseases or different stages of the same disease. In the future, we hope that in-depth research on the microenvironment of leukemia will lead to the development of safe and effective drugs targeting the microenvironment to reduce leukemia occurrence and inbibit disease progression.
Author contributions
KL and XYW chose the topic. KL is responsible for writing the article. RMJ and XYW contributed a lot in the revision and provided scientific advice. All authors read and approved the final manuscript.
Disclosure statement
No potential conflict of interest was reported by the author(s).
Additional information
Funding
References
- Orkin SH. Diversification of haematopoietic stem cells to specific lineages. Nat Rev Genet. 2000;1(1):57–64. doi:https://doi.org/10.1038/35049577.
- Galan-Diez M, Cuesta-Dominguez A, Kousteni S. The bone marrow microenvironment in health and myeloid malignancy. Cold Spring Harb Perspect Med. 2017;8(7):a031328, doi:https://doi.org/10.1101/cshperspect.a031328.
- Schofield R. The relationship between the spleen colony-forming cell and the haemopoietic stem cell. Blood Cells. 1978;4(1-2):7–25.
- Taichman RS. Blood and bone: two tissues whose fates are intertwined to create the hematopoietic stem-cell niche. Blood. 2005;105(7):2631–2639. doi:https://doi.org/10.1182/blood-2004-06-2480.
- Kim S, Lin L, Brown GAJ, et al. Extended time-lapse in vivo imaging of tibia bone marrow to visualize dynamic hematopoietic stem cell engraftment. Leukemia. 2017;31(7):1582–1592. doi:https://doi.org/10.1038/leu.2016.354.
- Huntly BJ, Gilliland DG. Leukaemia stem cells and the evolution of cancer-stem-cell research. Nat Rev Cancer. 2005;5(4):311–321. doi:https://doi.org/10.1038/nrc1592.
- Lane SW, Wang YJ, Lo Celso C, et al. Differential niche and Wnt requirements during acute myeloid leukemia progression. Blood. 2011;118(10):2849–2856. doi:https://doi.org/10.1182/blood-2011-03-345165.
- Krause DS, Fulzele K, Catic A, et al. Differential regulation of myeloid leukemias by the bone marrow microenvironment. Nat Med. 2013;19(11):1513–1517. doi:https://doi.org/10.1038/nm.3364.
- Walkley CR, Olsen GH, Dworkin S, et al. A microenvironment-induced myeloproliferative syndrome caused by retinoic acid receptor gamma deficiency. Cell. 2007;129(6):1097–1110. doi:https://doi.org/10.1016/j.cell.2007.05.014.
- Medyouf H, Mossner M, Jann JC, et al. Myelodysplastic cells in patients reprogram mesenchymal stromal cells to establish a transplantable stem cell niche disease unit. Cell Stem Cell. 2014;14(6):824–837. doi:https://doi.org/10.1016/j.stem.2014.02.014.
- Frisch BJ, Ashton JM, Xing L, et al. Functional inhibition of osteoblastic cells in an in vivo mouse model of myeloid leukemia. Blood. 2012;119(2):540–550. doi:https://doi.org/10.1182/blood-2011-04-348151.
- Kode A, Manavalan JS, Mosialou I, et al. Leukaemogenesis induced by an activating β-catenin mutation in osteoblasts. Nature. 2014;506(7487):240–244. doi:https://doi.org/10.1038/nature12883.
- Hussong JW, Rodgers GM, Shami PJ. Evidence of increased angiogenesis in patients with acute myeloid leukemia. Blood. 2000;95(1):309–313.
- Korn C, Méndez-Ferrer S. Myeloid malignancies and the microenvironment. Blood. 2017;129(7):811–822. doi:https://doi.org/10.1182/blood-2016-09-670224.
- Boissard F, Laurent C, Ramsay AG, et al. Nurse-like cells impact on disease progression in chronic lymphocytic leukemia. Blood Cancer J. 2016;6(1):e381, doi:https://doi.org/10.1038/bcj.2015.108.
- Edwards DK, Watanabe-Smith K, Rofelty A, Damnernsawad A, Laderas T, Lamble A, Lind EF, Kaempf A, Mori M, Rosenberg M, d'Almeida A, Long N, Agarwal A, Sweeney DT, Loriaux M, McWeeney SK, Tyner JW (2019) CSF1R inhibitors exhibit antitumor activity in acute myeloid leukemia by blocking paracrine signals from support cells. Blood 133 (6):588-599. doi:https://doi.org/10.1182/blood-2018-03-838946
- Le PM, Andreeff M, Battula VL. Osteogenic niche in the regulation of normal hematopoiesis and leukemogenesis. Haematologica. 2018;103(12):1945–1955. doi:https://doi.org/10.3324/haematol.2018.197004.
- Ono T, Nakashima T. Recent advances in osteoclast biology. Histochem Cell Biol. 2018;149(4):325–341. doi:https://doi.org/10.1007/s00418-018-1636-2.
- Pereira M, Petretto E, Gordon S, et al. Common signalling pathways in macrophage and osteoclast multinucleation. J Cell Sci. 2018;131(11). doi:https://doi.org/10.1242/jcs.216267.
- Xiao Y, Palomero J, Grabowska J, et al. Macrophages and osteoclasts stem from a bipotent progenitor downstream of a macrophage/osteoclast/dendritic cell progenitor. Blood Adv. 2017;1(23):1993–2006. doi:https://doi.org/10.1182/bloodadvances.2017008540.
- Biswas SK, Mantovani A. Macrophage plasticity and interaction with lymphocyte subsets: cancer as a paradigm. Nat Immunol. 2010;11(10):889–896. doi:https://doi.org/10.1038/ni.1937.
- Gordon S, Martinez FO. Alternative activation of macrophages: mechanism and functions. Immunity. 2010;32(5):593–604. doi:https://doi.org/10.1016/j.immuni.2010.05.007.
- Geissmann F, Gordon S, Hume DA, et al. Unravelling mononuclear phagocyte heterogeneity. Nat Rev Immunol. 2010;10(6):453–460. doi:https://doi.org/10.1038/nri2784.
- Chávez-Galán L, Olleros ML, Vesin D, et al. Much More than M1 and M2 macrophages, there are also CD169(+) and TCR(+) macrophages. Front Immunol. 2015;6:263, doi:https://doi.org/10.3389/fimmu.2015.00263.
- Udagawa N, Takahashi N, Akatsu T, et al. Origin of osteoclasts: mature monocytes and macrophages are capable of differentiating into osteoclasts under a suitable microenvironment prepared by bone marrow-derived stromal cells. Proc Natl Acad Sci USA. 1990;87(18):7260–7264. doi:https://doi.org/10.1073/pnas.87.18.7260.
- Speziani C, Rivollier A, Gallois A, et al. Murine dendritic cell transdifferentiation into osteoclasts is differentially regulated by innate and adaptive cytokines. Eur J Immunol. 2007;37(3):747–757. doi:https://doi.org/10.1002/eji.200636534.
- de Vries TJ, Schoenmaker T, Hooibrink B, et al. Myeloid blasts are the mouse bone marrow cells prone to differentiate into osteoclasts. J Leukoc Biol. 2009;85(6):919–927. doi:https://doi.org/10.1189/jlb.0708402.
- Miyamoto T, Ohneda O, Arai F, et al. Bifurcation of osteoclasts and dendritic cells from common progenitors. Blood. 2001;98(8):2544–2554. doi:https://doi.org/10.1182/blood.v98.8.2544.
- Kelleher FC, O'Sullivan H. Monocytes, macrophages, and osteoclasts in osteosarcoma. J Adolesc Young Adult Oncol. 2017;6(3):396–405. doi:https://doi.org/10.1089/jayao.2016.0078.
- Jacome-Galarza CE, Percin GI, Muller JT, et al. Developmental origin, functional maintenance and genetic rescue of osteoclasts. Nature. 2019;568(7753):541–545. doi:https://doi.org/10.1038/s41586-019-1105-7.
- Blangy A, Bompard G, Guerit D, et al. The osteoclast cytoskeleton - current understanding and therapeutic perspectives for osteoporosis. J Cell Sci. 2020;133(13), doi:https://doi.org/10.1242/jcs.244798.
- Llorente I, García-Castañeda N, Valero C, et al. Osteoporosis in rheumatoid arthritis: dangerous liaisons. Front Med (Lausanne). 2020;7:601618, doi:https://doi.org/10.3389/fmed.2020.601618.
- Fuji H, Ohmae S, Noma N, et al. Necrostatin-7 suppresses RANK-NFATc1 signaling and attenuates macrophage to osteoclast differentiation. Biochem Biophys Res Commun. 2018;503(2):544–549. doi:https://doi.org/10.1016/j.bbrc.2018.05.153.
- Yang D, Wan Y. Molecular determinants for the polarization of macrophage and osteoclast. Semin Immunopathol. 2019;41(5):551–563. doi:https://doi.org/10.1007/s00281-019-00754-3.
- Ghobrial IM, Detappe A, Anderson KC, et al. The bone-marrow niche in MDS and MGUS: implications for AML and MM. Nat Rev Clin Oncol. 2018;15(4):219–233. doi:https://doi.org/10.1038/nrclinonc.2017.197.
- Ishikawa F, Yoshida S, Saito Y, et al. Chemotherapy-resistant human AML stem cells home to and engraft within the bone-marrow endosteal region. Nat Biotechnol. 2007;25(11):1315–1321. doi:https://doi.org/10.1038/nbt1350.
- Pollard JW. Tumour-educated macrophages promote tumour progression and metastasis. Nat Rev Cancer. 2004;4(1):71–78. doi:https://doi.org/10.1038/nrc1256.
- Noy R, Pollard JW. Tumor-associated macrophages: from mechanisms to therapy. Immunity. 2014;41(1):49–61. doi:https://doi.org/10.1016/j.immuni.2014.06.010.
- Qian BZ, Pollard JW. Macrophage diversity enhances tumor progression and metastasis. Cell. 2010;141(1):39–51. doi:https://doi.org/10.1016/j.cell.2010.03.014.
- Al-Matary YS, Botezatu L, Opalka B, et al. Acute myeloid leukemia cells polarize macrophages towards a leukemia supporting state in a growth factor independence 1 dependent manner. Haematologica. 2016;101(10):1216–1227. doi:https://doi.org/10.3324/haematol.2016.143180.
- Polk A, Lu Y, Wang T, et al. Colony-Stimulating factor-1 receptor Is required for nurse-like Cell Survival in chronic lymphocytic leukemia. Clin Cancer Res: An Official J Am Assoc Cancer Res. 2016;22(24):6118–6128. doi:https://doi.org/10.1158/1078-0432.ccr-15-3099.
- Li K, Xu W, Lu K, et al. CSF-1R inhibition disrupts the dialog between leukaemia cells and macrophages and delays leukaemia progression. J Cell Mol Med. 2020;24(22):13115–13128. doi:https://doi.org/10.1111/jcmm.15916.
- Chen SY, Yang X, Feng WL, et al. Organ-specific microenvironment modifies diverse functional and phenotypic characteristics of leukemia-associated macrophages in mouse T cell acute lymphoblastic leukemia. J Immunol. 2015;194(6):2919–2929. doi:https://doi.org/10.4049/jimmunol.1400451.
- Chen S, Yang X, Feng W, et al. Characterization of peritoneal leukemia-associated macrophages in Notch1-induced mouse T cell acute lymphoblastic leukemia. Mol Immunol. 2017;81:35–41. doi:https://doi.org/10.1016/j.molimm.2016.11.014.
- Chen YCE, Mapp S, Blumenthal A, et al. The duality of macrophage function in chronic lymphocytic leukaemia. Biochim Biophys Acta Rev Cancer. 2017;1868(1):176–182. doi:https://doi.org/10.1016/j.bbcan.2017.03.006.
- Gutiérrez-González A, Martínez-Moreno M, Samaniego R, et al. Evaluation of the potential therapeutic benefits of macrophage reprogramming in multiple myeloma. Blood. 2016;128(18):2241–2252. doi:https://doi.org/10.1182/blood-2016-01-695395.
- Werner L, Dreyer JH, Hartmann D, et al. Tumor-associated macrophages in classical Hodgkin lymphoma: hormetic relationship to outcome. Sci Rep. 2020;10(1):9410, doi:https://doi.org/10.1038/s41598-020-66010-z.
- Yokota A, Kimura S, Tanaka R, et al. Osteoclasts are involved in the maintenance of dormant leukemic cells. Leuk Res. 2010;34(6):793–799. doi:https://doi.org/10.1016/j.leukres.2009.08.034.
- Kessenbrock K, Plaks V, Werb Z. Matrix metalloproteinases: regulators of the tumor microenvironment. Cell. 2010;141(1):52–67. doi:https://doi.org/10.1016/j.cell.2010.03.015.
- An G, Acharya C, Feng X, et al. Osteoclasts promote immune suppressive microenvironment in multiple myeloma: therapeutic implication. Blood. 2016;128(12):1590–1603. doi:https://doi.org/10.1182/blood-2016-03-707547.
- Auberger P, Puissant A. Autophagy, a key mechanism of oncogenesis and resistance in leukemia. Blood. 2017;129(5):547–552. doi:https://doi.org/10.1182/blood-2016-07-692707.
- Huang FL, Yu SJ, Li CL. Role of autophagy and apoptosis in acute lymphoblastic leukemia. Cancer Control. 2021;28:10732748211019138, doi:https://doi.org/10.1177/10732748211019138.
- Maes H, Kuchnio A, Peric A, et al. Tumor vessel normalization by chloroquine independent of autophagy. Cancer Cell. 2014;26(2):190–206. doi:https://doi.org/10.1016/j.ccr.2014.06.025.
- Polak R, Bierings MB, van der Leije CS, et al. Autophagy inhibition as a potential future targeted therapy for ETV6-RUNX1-driven B-cell precursor acute lymphoblastic leukemia. Haematologica. 2019;104(4):738–748. doi:https://doi.org/10.3324/haematol.2018.193631.
- Hao M, Franqui-Machin R, Xu H, et al. NEK2 induces osteoclast differentiation and bone destruction via heparanase in multiple myeloma. Leukemia. 2017;31(7):1648–1650. doi:https://doi.org/10.1038/leu.2017.115.
- Franqui-Machin R, Hao M, Bai H, et al. Destabilizing NEK2 overcomes resistance to proteasome inhibition in multiple myeloma. J Clin Invest. 2018;128(7):2877–2893. doi:https://doi.org/10.1172/jci98765.
- Borge M, Delpino MV, Podaza E, et al. Soluble RANKL production by leukemic cells in a case of chronic lymphocytic leukemia with bone destruction. Leuk Lymphoma. 2016;57(10):2468–2471. doi:https://doi.org/10.3109/10428194.2016.1151506.
- Bellon M, Ko NL, Lee MJ, et al. Adult T-cell leukemia cells overexpress Wnt5a and promote osteoclast differentiation. Blood. 2013;121(25):5045–5054. doi:https://doi.org/10.1182/blood-2012-07-439109.
- Rajakumar SA, Papp E, Lee KK, et al. B cell acute lymphoblastic leukemia cells mediate RANK-RANKL-dependent bone destruction. Sci Transl Med. 2020;12(561):eaba5942, doi:https://doi.org/10.1126/scitranslmed.aba5942.
- Lopez-Villar O, Garcia JL, Sanchez-Guijo FM, et al. Both expanded and uncultured mesenchymal stem cells from MDS patients are genomically abnormal, showing a specific genetic profile for the 5q- syndrome. Leukemia. 2009;23(4):664–672. doi:https://doi.org/10.1038/leu.2008.361.
- Baba T, Mukaida N. Role of macrophage inflammatory protein (MIP)-1α/CCL3 in leukemogenesis. Mol Cell Oncol. 2014;1(1):e29899, doi:https://doi.org/10.4161/mco.29899.
- Sica A, Schioppa T, Mantovani A, et al. Tumour-associated macrophages are a distinct M2 polarised population promoting tumour progression: potential targets of anti-cancer therapy. Eur J Cancer. 2006;42(6):717–727. doi:https://doi.org/10.1016/j.ejca.2006.01.003.
- Gautam S, Fatehchand K, Elavazhagan S, et al. Reprogramming nurse-like cells with interferon γ to interrupt chronic lymphocytic leukemia cell survival. J Biol Chem. 2016;291(27):14356–14362. doi:https://doi.org/10.1074/jbc.M116.723551.
- Papin A, Tessoulin B, Bellanger C, et al. CSF1R and BTK inhibitions as novel strategies to disrupt the dialog between mantle cell lymphoma and macrophages. Leukemia. 2019;33(10):2442–2453. doi:https://doi.org/10.1038/s41375-019-0463-3.
- Wang Q, Lu Y, Li R, et al. Therapeutic effects of CSF1R-blocking antibodies in multiple myeloma. Leukemia. 2018;32(1):176–183. doi:https://doi.org/10.1038/leu.2017.193.
- Kojima Y, Volkmer JP, McKenna K, et al. CD47-blocking antibodies restore phagocytosis and prevent atherosclerosis. Nature. 2016;536(7614):86–90. doi:https://doi.org/10.1038/nature18935.
- Vonderheide RH. CD47 blockade as another immune checkpoint therapy for cancer. Nat Med. 2015;21(10):1122–1123. doi:https://doi.org/10.1038/nm.3965.
- Liu J, Wang L, Zhao F, et al. Pre-Clinical development of a humanized anti-CD47 antibody with anti-cancer therapeutic potential. PloS one. 2015;10(9):e0137345, doi:https://doi.org/10.1371/journal.pone.0137345.
- Marini C, Bruno S, Fiz F, et al. Functional activation of osteoclast commitment in chronic lymphocytic leukaemia: a possible role for RANK/RANKL pathway. Sci Rep. 2017;7(1):14159, doi:https://doi.org/10.1038/s41598-017-12761-1.
- Schmiedel BJ, Scheible CA, Nuebling T, et al. RANKL expression, function, and therapeutic targeting in multiple myeloma and chronic lymphocytic leukemia. Cancer Res. 2013;73(2):683–694. doi:https://doi.org/10.1158/0008-5472.can-12-2280.
- Secchiero P, Corallini F, Barbarotto E, et al. Role of the RANKL/RANK system in the induction of interleukin-8 (IL-8) in B chronic lymphocytic leukemia (B-CLL) cells. J Cell Physiol. 2006;207(1):158–164. doi:https://doi.org/10.1002/jcp.20547.
- Fields GB. The rebirth of matrix metalloproteinase inhibitors: moving beyond the dogma. Cells. 2019;8(9), doi:https://doi.org/10.3390/cells8090984.
- Giannakoulas NC, Terpos E, Palassopoulou M, et al. Increased RANKL and IL-6 levels might result in high bone turnover in a case of a CD34+/CD117+/myeloperoxidase(+dim) acute myeloid leukemia presenting with severe hypercalcemia and lumbar spine fractures. Leuk Res. 2011;35(11):e188–e189. doi:https://doi.org/10.1016/j.leukres.2011.07.006.
- Padhye B, Dalla-Pozza L, Little DG, et al. Use of zoledronic acid for treatment of chemotherapy related osteonecrosis in children and adolescents: a retrospective analysis. Pediatr Blood Cancer. 2013;60(9):1539–1545. doi:https://doi.org/10.1002/pbc.24563.
- Segawa H, Kimura S, Kuroda J, et al. Zoledronate synergises with imatinib mesylate to inhibit Ph primary leukaemic cell growth. Br J Haematol. 2005;130(4):558–560. doi:https://doi.org/10.1111/j.1365-2141.2005.05648.x.
- Kuroda J, Kimura S, Segawa H, et al. The third-generation bisphosphonate zoledronate synergistically augments the anti-Ph+ leukemia activity of imatinib mesylate. Blood. 2003;102(6):2229–2235. doi:https://doi.org/10.1182/blood-2003-01-0305.