ABSTRACT
Objective
Our previous study demonstrated that iron overload could lead to haemophilic cartilage destruction by changing chondrocyte phenotype. This change was caused by iron’s effect on chondrocyte expression of FGF23 and SOX9, in addition to iron-induced chondrocyte apoptosis and cartilage extracellular matrix degradation. However, the underlying mechanisms remain unclear. This study aimed to determine the mechanism by which iron influences chondrocyte phenotype in the pathogenesis of haemophilic cartilage destruction.
Methods
The expression of the PTEN/PI3K/AKT/FOXO1 signal pathway in the articular cartilage of patients with haemophilic arthritis (HA) or osteoarthritis (OA) was determined using western blot (WB). Additionally, we quantified the expression of iron-induced PTEN, PI3K, p-PI3K, AKT, p-AKT, FOXO1, and p-FOXO1 in primary human normal chondrocyte cells (HUM-iCell-s018) using WB.
Results
We found that compared to that in patients with OA, the expression of PTEN, PI3K, AKT, and FOXO1 in the articular cartilage of patients with HA was up-regulated, while the expression of p-PI3K, p-AKT, and p-FOXO1 was down-regulated. Additionally, iron increased the expression of PTEN, PI3K, AKT, and FOXO1 and suppressed that of p-PI3K, p-AKT, and p-FOXO1 in chondrocytes in a dose-dependent manner.
Conclusions
Our findings demonstrated that iron was involved in the pathogenesis of haemophilic cartilage destruction by affecting chondrocyte phenotype through the inhibition of the PTEN/PI3K/AKT/FOXO1 pathway.
1. Introduction
Haemophilia is a rare congenital, recessive X-linked disorder caused by deficiency or absence of coagulation factor IX (FIX) or VIII (FVIII) [Citation1]. Hemarthrosis resulting from recurrent joint bleeding due to coagulation factor deficiency can induce the destruction of all structures within the joint, such as the menisci, bone, and cartilage, ultimately leading to haemophilic arthritis (HA) [Citation2].
Articular cartilage destruction is a major feature of HA. It is currently agreed that cartilage destruction in HA is induced by the interaction of joint bleeding, inflammation, and mechanical stress. Iron overload resulting from joint bleeding is considered the most decisive factor in distinguishing HA from osteoarthritis (OA), which is mainly due to abnormal inflammation and mechanical stress [Citation3]. It has been established that iron overload can induce haemophilic cartilage lesions indirectly by destroying cartilage extracellular matrix and directly by inducing chondrocyte apoptosis. However, little attention has been given to the effects of iron on chondrocyte phenotype changes which may be another important cause of HA cartilage destruction. FGF23 and SOX9 are widely studied regulatory factors in cartilage metabolism. Our previous study found higher expression of FGF23 and lower expression of SOX9 in HA cartilage than in OA. Additionally, we showed that iron could regulate the expression of FGF23 and SOX9 in chondrocytes [Citation4]; however, the mechanisms behind this remain unclear.
Forkhead box transcription factor O1 (FOXO1) is an essential regulatory factor in cartilage metabolism [Citation5,Citation6]. Studies have shown that high expression of FOXO1 and low expression of pFOXO1 can lead to higher expression of FGF23 and lower expression of SOX9 in chondrocytes, respectively [Citation7–9]. The PTEN/PI3K/AKT pathway is another crucial pathway for maintaining joint health and is closely related to chondrocyte growth, proliferation, and survival [Citation10–12]. FOXO1 is downstream of the PTEN/PI3K/AKT pathway, and inhibition of this pathway increases FOXO1 expression and suppresses pFOXO1 expression in chondrocytes [Citation5]. Hence, we suspected that iron overload may cause high expression FOXO1, low expression pFOXO1, and subsequent up-regulation of FGF23, and down-regulation of SOX9 expression in HA cartilage by inhibiting the PTEN/PI3K/AKT pathway.
This study aimed to explore the mechanism by which iron overload regulates chondrocyte phenotype in haemophilic cartilage. Firstly, we detected the expression of the PTEN/PI3 K/AKT/FOXO1 signal pathway in HA and OA cartilage. Then, we determined how iron regulates the above signalling pathway in chondrocytes in vitro.
2. Materials and methods
2.1. Reagents
Ferric ammonium citrate (FAC) was purchased from Sigma (1185-57-5). Antibodies for PTEN (UK, Ab170941, diluted 1:500) were purchased from Abcam, while antibodies for PI3K (USA, AF6241, diluted 1:500), p-PI3K (USA, AF3242, diluted 1:500), AKT (USA, AF6261, diluted 1:500), p-AKT (USA, AF0016, diluted 1:500), FOXO1 (USA, AF6416, diluted 1:500), and p-FOXO1 (USA, AF3416, diluted 1:500) were purchased from Affinit.
2.2. Clinical specimens
Eight knee articular cartilages (OA (4); HA (4)) were collected from male patients who underwent total knee arthroplasty at our hospital between January and April 2022. The OA group consisted of patients with a mean age of 77.25 ± 3.86 years (range, 73–81 years; Kellgren-Lawrence grade IV), while the HA group had a mean age of 24.75 ± 2.63 years (range, 21–27 years; Modified Arnold-Hilgartner system grade IV). All patients with HA were type A (1 for moderate; 3 for severe) without alloantibodies to FVIII. The Institutional Ethical Review Board of the Second Affiliated Hospital of Anhui Medical University approved this study, and informed consent was obtained from all participants.
2.3. Cell culture
Primary human normal chondrocyte cells (HUM-iCell-s018) were purchased from iCell Bioscience Inc. The cells were cultured in the ICell Primary Chondrocyte Culture System (PriMed-iCell-020) under a humidified, 5% CO2 atmosphere at 37°C. For all assays, primary chondrocytes were used without subculturing to preserve the chondrocyte phenotype. Chondrocytes were initially seeded in 6-well plates at a density of 5 × 105 cells/well. After 16 h, chondrocytes were treated with FAC (1, 5, 10, 25, 50, 75 ng/mL) for 24 h, and then the cells were collected for subsequent detection.
2.4. Western blot (WB)
Protein samples were extracted from knee articular cartilage and prepared using RIPA lysis buffer (Biosharp, China, BL504A). Similarly, chondrocytes were washed three times with PBS and then prepared using RIPA lysis buffer (Biosharp, China, BL504A). The proteins were separated using sodium dodecyl sulfate-polyacrylamide gel electrophoresis (SDS-PAGE) and transferred to a polyvinylidene difluoride membrane (Millipore, United States). Protein bands were visualized using ECL Chemiluminescent Substrate Reagent Kit (Thermo, China) and JS-M6P (Peiqing Technology Co., Ltd., Shanghai, China), and the density of each band was quantified using Image J version 1.52.
2.5. Statistical analysis
All analyses were performed using SPSS software version 24.0 (IBM Corp., Chicago, IL, USA). Statistical significance between the two tissue groups was determined using covariance analysis to eliminate the effect of age differences. One-way ANOVA was performed to analyse the significant differences in protein expression among treatment groups, followed by Bonferroni’s post-hoc test. Results are plotted as mean ± SD. A p-value < 0.05 was considered statistically significant.
3. Results
3.1. PTEN/PI3 K/AKT/FOXO1 signalling pathway expression
The protein expression of PTEN, PI3 K, p-PI3 K, AKT, p-AKT, FOXO1, and p-FOXO1 in OA and HA articular cartilage was determined using WB ((A–C)). PTEN, PI3 K, AKT, and FOXO1 expression were significantly up-regulated, while p-PI3 K, p-AKT and p-FOXO1 were significantly down-regulated in HA compared with OA.
3.2. Effect of FAC on protein expression of PTEN/PI3 K/AKT/FOXO1 signalling pathway in chondrocytes
As shown in (D–K), the protein expression of PTEN, PI3 K, AKT, and FOXO1 was up-regulated, while that of p-PI3 K, p-AKT, and p-FOXO1 were down-regulated in a dose-dependent manner when stimulated by a gradient concentration of FAC.
Figure 2. Effects of iron on PTEN, PI3K, p-PI3K, AKT, p-AKT, FOXO1, and p-FOXO1 expression in HUM-iCell-s018 chondrocyte cells. Cells were treated with an increasing gradient concentration of ferric ammonium citrate (0, 1, 5, 10, 25, 50, and 75 mM) for 24 h, then protein expression of PTEN, PI3K, p-PI3K, AKT, p-AKT, FOXO1, and p-FOXO1 in chondrocytes was determined using western blotting (D). Data are expressed with mean ± SD; (E-K) *p < 0.05, **p < 0.01 and ***p < 0.001. All experiments were repeated three times independently.
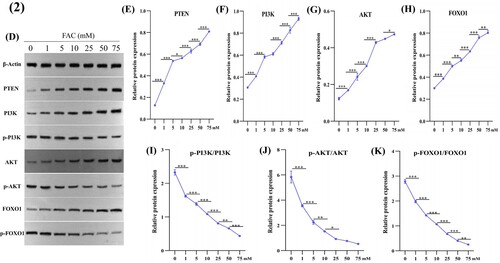
4. Discussion
Although the role of iron overload in the pathogenesis of haemophilic cartilage destruction has been given much concern and study, little attention has been paid to the association between iron overload and chondrocyte phenotype changes. Our previous study demonstrated that iron overload may also cause haemophilic cartilage destruction by increasing the FGF23 expression and decreasing the SOX9 expression in chondrocytes; however, the mechanism remained unclear [Citation4].
We have found, for the first time, that HA articular cartilage had higher levels of PTEN, PI3 K, AKT, and FOXO1 and lower levels of p-PI3 K, p-AKT, p-FOXO1 than OA. Histological examination results verified that the PTEN/PI3 K/AKT/FOXO1 pathway was down-regulated in HA articular cartilage compared with OA. Studies have reported that the PTEN/PI3 K/AKT/FOXO1 pathway is inhibited in OA compared with normal articular cartilage [Citation13]. Some proinflammatory cytokines, such as tumour necrosis factor alpha (TNF-α) and interleukin (IL)−1β, have been shown to suppress the PI3 K/AKT/FOXO1 pathway by elevating PTEN in chondrocytes [Citation14,Citation15]. HA articular cartilage showed a more potent inhibiting effect on the PI3 K/AKT/FOXO1 pathway than OA did, probably because of the higher level of intra-articular TNF-α and IL-1β in HA. Iron overload may be another important contributing factor.
Additionally, we found that iron overload inhibited the PI3 K/AKT/FOXO1 pathway in a dose-dependent manner by inducing PTEN expression in chondrocytes (). However, Jing et al. showed that inhibition of DMT1-mediated iron inflow suppresses IL-1β induced PI3 K/AKT pathway activation in chondrocytes, which indicated that iron may activate the PI3 K/AKT pathway, which is inconsistent with our findings [Citation16]. This is the first time that we have observed that iron overload induced PTEN expression, which acts as an upstream inhibitory regulator of the PI3K/AKT/FOXO1 pathway and can prevent the progression of AKT-mediated downstream signalling by preventing the formation of PIP3 from PIP2 [Citation17]. Controlled regulation of the PI3 K/AKT/FOXO1 signalling pathway is essential for maintaining the normal physiological state of chondrocyte cells, and this is orchestrated by PTEN [Citation18]. Tumour suppressor p53, a modulator of the apoptosis and proliferation processes, which acts as an important upstream inducible factor of PTEN, was found in HA cartilage at a high level in another ongoing research [Citation19]. Yang et al. found that iron overload could induce the expressions of p53 in bone marrow mesenchymal stem cells [Citation20]. Iron overload has been shown to increase p53 expression in RAW264.7 macrophages by elevating ROS production [Citation21]. Additionally, iron has been reported to up-regulate the p53 expression in C2C12 myoblasts, C2C12 myotubes, and the murine preosteoblast cell line MC3T3-E1.2 [Citation22,Citation23]. Therefore, iron may up-regulate PTEN levels in chondrocytes by inducing p53 expression.
Figure 3. PTEN is up-regulated by iron stimulation and acts as a repressor of the PI3K/AKT pathway, which increases the expression of FOXO1, leading to highly expressed FGF23 and lowly expressed SOX9 in HA chondrocytes. HA, haemophilic arthritis.
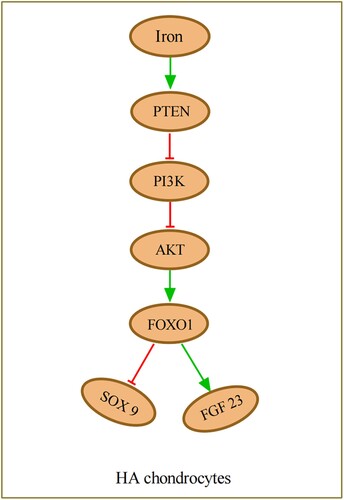
Peroxisome proliferator-activated receptor gamma (PPARγ) is another upstream inducible factor of PTEN and is an important regulatory factor of cartilage metabolism [Citation24,Citation25]. It has been reported that iron can up-regulate PPARγ expression in intestinal epithelial cells (IECs) [Citation26]. Studies have also demonstrated that iron overload leads to high levels of PPARγ in the hepatic tissues of male Wistar rats [Citation27,Citation28]. Hence, it was also possible that iron up-regulates PTEN levels in chondrocytes by inducing PPARγ expression. Additionally, iron may regulate PTEN expression through two other upstream inducible factors, human sprout homolog 2 (SPRY2) and activating transcription factor 2 (ATF2) [Citation29,Citation30]. Further research is needed to explore new possible mechanisms of iron elevation of PTEN expression or verify the abovementioned speculation.
In this study, we first demonstrated the inhibition of the PTEN/PI3 K/AKT/FOXO1 pathway in the articular cartilage of HA compared with OA. Additionally, we found a positive dose–response relationship between iron and PTEN/PI3 K/AKT/FOXO1 pathway inhibition. Our findings suggest that iron was involved in haemophilic cartilage destruction pathogenesis by changing chondrocyte phenotype via PI3 K/AKT/FOXO1 pathway inhibition by inducing PTEN. Although possible theoretical underlying mechanisms for iron regulation of PTEN in chondrocytes were highlighted, the exact mechanisms are still unclear. Therefore, identifying the pathways through which iron regulates chondrocyte expression of PTEN will be crucial in fully understanding the effects of iron in chondrocyte phenotype changes. This will be the focus of our next study.
Acknowledgements
The study was designed by Zheng and Yao. The entire experiment was performed by Zheng, Luo, Han, Pang and Ding. Zhang, Ye and Zhu analysed the data. The manuscript was written and revised by Zheng and Yi. The progressive drafts of the manuscript were reviewed critically, and the final version was approved by all authors.
Disclosure statement
No potential conflict of interest was reported by the author(s).
Data availability statement
The data that support the findings of this study are available from the corresponding author, [W.Y], upon reasonable request.
Additional information
Funding
References
- Bolton-Maggs PHB, Pasi KJ. Haemophilias A and B. Lancet. 2003;361(9371):1801–1809. doi:10.1016/S0140-6736(03)13405-8
- Zhu H, Meng Y, Tong P, et al. Pathological mechanism of joint destruction in haemophilic arthropathy. Mol Biol Rep. 2021;48(1):969–974. doi:10.1007/s11033-020-06052-8
- Pulles AE, Mastbergen SC, Schutgens REG, et al. Pathophysiology of hemophilic arthropathy and potential targets for therapy. Pharmacol Res. 2017;115:192–199. doi:10.1016/j.phrs.2016.11.032
- Zheng L, Han Z, Luo D, et al. FGF23 and SOX9 expression in haemophilic cartilage: in vitro studies of the effects of iron. Haemophilia. 2022;28(6):1062–1068. doi:10.1111/hae.14623
- Yue J, Aobulikasimu A, Sun W, et al. Targeted regulation of FoxO1 in chondrocytes prevents age-related osteoarthritis via autophagy mechanism. J Cell Mol Med. 2022;26(11):3075–3082. doi:10.1111/jcmm.17319
- Graves DT, Milovanova TN. Mucosal immunity and the FOXO1 transcription factors. Front Immunol. 2019;10:2530. doi:10.3389/fimmu.2019.02530
- Liang C, Xing H, Wang C, et al. Resveratrol protection against IL-1β-induced chondrocyte damage via the SIRT1/FOXO1 signaling pathway. J Orthop Surg Res. 2022;17(1):406. doi:10.1186/s13018-022-03306-y
- Bär L, Feger M, Fajol A, et al. Insulin suppresses the production of fibroblast growth factor 23 (FGF23). Proc Natl Acad Sci USA. 2018;115(22):5804–5809. doi:10.1073/pnas.1800160115
- Tsitsipatis D, Gopal K, Steinbrenner H, et al. FOXO1 cysteine-612 mediates stimulatory effects of the coregulators CBP and PGC1α on FOXO1 basal transcriptional activity. Free Radic Biol Med. 2018;118:98–107. doi:10.1016/j.freeradbiomed.2018.02.034
- Litherland GJ, Dixon C, Lakey RL, et al. Synergistic collagenase expression and cartilage collagenolysis are phosphatidylinositol 3-kinase/Akt signaling-dependent. J Biol Chem. 2008;283(21):14221–14229. doi:10.1074/jbc.M710136200
- Wymann MP, Zvelebil M, Laffargue M. Phosphoinositide 3-kinase signalling – which way to target? Trends Pharmacol Sci. 2003;24(7):366–376. doi:10.1016/S0165-6147(03)00163-9
- Kurakazu I, Akasaki Y, Hayashida M, et al. FOXO1 transcription factor regulates chondrogenic differentiation through transforming growth factor β1 signaling. J Biol Chem. 2019;294(46):17555–17569. doi:10.1074/jbc.RA119.009409
- Sun K, Luo J, Guo J, et al. The PI3K/AKT/mTOR signaling pathway in osteoarthritis: a narrative review. Osteoarthritis Cartilage. 2020;28(4):400–409. doi:10.1016/j.joca.2020.02.027
- Jiang J-M, Mo M-L, Long X-P, et al. MiR-144-3p induced by SP1 promotes IL-1β-induced pyroptosis in chondrocytes via PTEN/PINK1/Parkin axis. Autoimmunity. 2022;55(1):21–31. doi:10.1080/08916934.2021.1983802
- Chen Y, Zhang L, Li E, et al. Long-chain non-coding RNA HOTAIR promotes the progression of osteoarthritis via sponging miR-20b/PTEN axis. Life Sci. 2020;253:117685. doi:10.1016/j.lfs.2020.117685
- Jing X, Lin J, Du T, et al. Iron overload is associated with accelerated progression of osteoarthritis: the role of DMT1 mediated iron homeostasis. Front Cell Dev Biol. 2021;8:594509. doi:10.3389/fcell.2020.594509
- Chen C-Y, Chen J, He L, et al. Pten: tumor suppressor and metabolic regulator. Front Endocrinol (Lausanne). 2018;9:338. doi:10.3389/fendo.2018.00338
- Boosani CS, Gunasekar P, Agrawal DK. An update on PTEN modulators – a patent review. Expert Opin Ther Pat. 2019;29(11):881–889. doi:10.1080/13543776.2019.1669562
- Stambolic V, Woodgett JR. Functional distinctions of protein kinase B/Akt isoforms defined by their influence on cell migration. Trends Cell Biol. 2006;16(9):461–466. doi:10.1016/j.tcb.2006.07.001
- Yang F, Yang L, Li Y, et al. Melatonin protects bone marrow mesenchymal stem cells against iron overload-induced aberrant differentiation and senescence. J Pineal Res. 2017;63(3):e12422. doi:10.1111/jpi.12422
- Zhou Y, Que K-T, Zhang Z, et al. Iron overloaded polarizes macrophage to proinflammation phenotype through ROS/acetyl-p53 pathway. Cancer Med. 2018;7(8):4012–4022. doi:10.1002/cam4.1670
- Huang Y, Wu B, Shen D, et al. Ferroptosis in a sarcopenia model of senescence accelerated mouse prone 8 (SAMP8). Int J Biol Sci. 2021;17(1):151–162. doi:10.7150/ijbs.53126
- Cen W-J, Feng Y, Li S-S, et al. Iron overload induces G1 phase arrest and autophagy in murine preosteoblast cells. J Cell Physiol. 2018;233(9):6779–6789. doi:10.1002/jcp.26405
- Patel L, Pass I, Coxon P, et al. Tumor suppressor and anti-inflammatory actions of PPARγ agonists are mediated via upregulation of PTEN. Curr Biol. 2001;11(10):764–768. doi:10.1016/S0960-9822(01)00225-1
- Zhao X, Petursson F, Viollet B, et al. Peroxisome proliferator-activated receptor γ coactivator 1α and FoxO3A mediate chondroprotection by AMP-activated protein kinase. Arthritis Rheumatol. 2014;66(11):3073–3082. doi:10.1002/art.38791
- Song C-C, Pantopoulos K, Chen G-H, et al. Iron increases lipid deposition via oxidative stress-mediated mitochondrial dysfunction and the HIF1α-PPARγ pathway. Cell Mol Life Sci. 2022;79(7):394. doi:10.1007/s00018-022-04423-x
- Salama SA, Elshafey MM. Galangin mitigates iron overload-triggered liver injury: up-regulation of PPARγ and Nrf2 signaling, and abrogation of the inflammatory responses. Life Sci. 2021;283:119856. doi:10.1016/j.lfs.2021.119856
- Salama SA, Elshafey MM. Cross-talk between PPARγ, NF-κB, and p38 MAPK signaling mediates the ameliorating effects of bergenin against the iron overload-induced hepatotoxicity. Chem Biol Interact. 2022;368:110207. doi:10.1016/j.cbi.2022.110207
- Worby CA, Dixon JE. PTEN. Annu Rev Biochem. 2014;83:641–669. doi:10.1146/annurev-biochem-082411-113907
- Brito B, Goulielmaki M, Papakonstanti E, et al. Focus on PTEN regulation. Front Oncol. 2015;5:166. doi:10.3389/fonc.2015.00166.