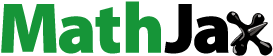
ABSTRACT
Chronic myeloid leukemia (CML) is a clonal myeloproliferative growth of human pluripotent stem cells which is estimated to occur at a rate of 1/100000 populations every year worldwide. A characteristic feature of this disease is the presence of the Philadelphia chromosome genotype, which results from the reciprocal translocation between human chromosomes 9 and 22. Two types of major genotypes are involved, which consequently result in two major types of expressed fusion mRNA transcripts: b3a2 and b2a2, i.e. major breakpoint segments (happening after exon 13 & after exon 14) of the BCR gene on chromosome 22 fuze with the ABL1 gene breakpoint (happening after exon 2) on chromosome 9, forming two genotypes coding for two transcripts: b3a2 (e14a2) and b2a2 (e13a2). The protein ‘p210 BCR–ABL1’, a protein which characteristically exhibits a high tyrosine kinase activity which is followed by the activation of various cellular processes that lead to increased cellular proliferation and cancer, is coded by both major BCR – ABL1 mRNA transcripts. Recent developments in the treatment of CML through molecular monitoring of the disease have managed to reduce patient morbidity and mortality. Advanced molecular techniques are aimed at detecting BCR–ABL1 transcript levels to monitor treatment response. Transcript typing is necessary to detect minimal residual disease and to achieve molecular response by helping to provide selective therapy based on the type of transcript identified, as transcript type is correlated with the disease course.
The purpose of this review is to discuss: the role of the BCR–ABL1 fusion gene in the pathogenesis of CML; the role of BCR–ABL1 transcript characterization in the molecular monitoring of CML therapy; the association of BCR – ABL1 transcript types with different CML phenotypes, molecular responses, and treatment responses; and the laboratory techniques employed to detect and characterize BCR – ABL1 transcripts.
1. Introduction
Chronic myeloid leukemia (CML) is a clonal myeloproliferative tumor of human pluripotent stem cells which is estimated to occur at a rate of 1/100000 populations every year worldwide. Rare studies have been conducted on the incidence of CML in Africa, including Ethiopia; one report in 2017 put the incidence in Africa at a rate of 0.4 cases per 100,000 populations per year. A characteristic feature of this disease is the presence of the Philadelphia chromosome genotype, which results from the reciprocal translocation between human chromosomes 9 and 22. A housekeeping gene of human chromosome 22, which is called the breakpoint cluster region (BCR), fuzes with the Abelson (ABL) gene of human chromosome 9, forming the BCR–ABL1 fusion gene or translocation t(9:22). The ABL1 gene breakpoint usually happens at the second exon (a2), while the BCR gene breakpoint variably happens in either of three regions: the major breakpoint cluster region (M–BCR), the minor breakpoint cluster region (m–BCR), and the micro breakpoint cluster region (μ-BCR) [Citation1–3]. The BCR–ABL1 fusion gene is transcribed into messenger ribonucleic acid (mRNA) and translated into BCR–ABL1 proteins designated as p210, p190 and p230, as well as other rare phenotypes based on the type of break points involved. These BCR–ABL1 proteins exhibit a consequent phenotypic expression of undesired auto – phosphorylation and uncontrolled signaling to downstream proteins, a process which is followed by the activation of undesirable cellular pathways leading to tumor initiation and development. The translated proteins of the BCR–ABL1 fusion gene have different sizes due to the presence of different breakpoints in the BCR gene. These differentially sized proteins possess high tyrosine kinase activity and are involved in the initiation and development of chronic myeloid leukemia with varied phenotypic manifestations. Nearly 90% of CML cases with the Philadelphia chromosome (PH+) involve the major breakpoint cluster region (M – BCR) of the BCR gene.
Two types of major breakpoints are involved, which consequently result in two types of fusion mRNA transcripts: b3a2 and b2a2. The transcript b3a2 (e14a2) is the result of a breakpoint that takes place after the 14th exon of the BCR gene (b3), and the b2a2 (e13a2) transcript is the result of a major breakpoint after the 13th exon of BCR (b2). Both breakpoints fuze with exon 2 (a2) of the ABL1 gene, forming two genotypes coding for two mRNA transcripts: b3a2 (e14a2) and b2a2 (e13a2). The protein ‘p210 BCR – ABL1’ is encoded by both fusion mRNA transcripts, although the protein may also be encoded by other rare genotypes. Besides, p210 BCR – ABL1 protein may also be present in other types of leukemia in rare cases [Citation4–6]. The BCR–ABL1 oncoprotein interacts with many cellular substrates in the cytoplasm of the cell, leading to the initiation of abnormal pathways characterized by high protein kinase activity, decreased apoptosis, and malignant transformation. High BCR–ABL1 expression and activity in chronic myeloid leukemia (CML) is a consequence of alteration of the normal cellular and genetic characteristics, which further leads to transformation and progression to advanced phases of the disease [Citation7]. The major contribution of the BCR–ABL1 protein to CML pathogenesis and progression was not known until the late 1900s, when new therapeutic agents called tyrosine kinase inhibitors that inhibit the tyrosine kinase activity of the BCR – ABL1 oncoprotein and halt the progression of the disease were introduced into the clinical management of CML, among which the drug Imatinib has gained popularity in the treatment of CML. There are also new generations of tyrosine kinase inhibitors available for alternative treatment of CML [Citation8]. CML patients can be diagnosed by either cytogenetic (standard or fluorescence in situ hybridization) or molecular techniques. Polymerase Chain Reaction (PCR) being a rapid and sensitive method for in vitro amplification of specific DNA segments, can especially aid in delivering optimal therapy by providing information regarding treatment success and patient prognosis. The occurrence of BCR–ABL1 transcript types in different CML phenotypes can be detected by multiplex reverse transcriptase polymerase chain reaction (multiplex RT–PCR), which can assist in assessing the prognosis & treatment response of the disease and greatly save time as this assay allows the simultaneous detection of two or more genes in the same reaction. The frequency of different BCR–ABL1 transcript types shows variation among different studies. Besides, the effect of these transcript types on the phenotypic characteristics of CML patients has been an issue of conflict [Citation9, Citation10].
Generally, the incidence of the different types of leukemia varies among people according to differences in ages, sexes, and races. In a study conducted to assess incidence trends of leukemia across different populations between 1990 and 2017, the number of CML cases increased globally from 31.810x103 in 1990 to 34.210x103in 2017, with the highest incidence of CML being found in Ethiopia (1.98/105 populations), followed by Brunei and Honduras in 2017 [Citation11]. This review was prepared from articles published in different scientific journals by using Google Scholar and PubMed search engines. Peer-reviewed, online-available, open-access works submitted by various authors were included. The purpose of this review is to discuss and assess the role of BCR–ABL1 transcript characterization in the molecular monitoring of CML therapy, the association of BCR–ABL1 transcript types with CML phenotypes, molecular response/treatment response according to various studies, and the laboratory techniques employed to detect and characterize BCR – ABL1 transcripts.
2. Philadelphia chromosome and pathogenesis of chronic myeloid leukemia
The Philadelphia chromosome was first described in a 1960 comparative chromosomal analysis study of normal and leukemic cases by Nowell and Hungerford in 1960. Out of 4 cases of granulocytic leukemia cases investigated, definite abnormalities of chromosome morphology were present in 2 leukemic patients who were admitted to Philadelphia General Hospital. In the two cases of chronic leukemia, ideogram analyses revealed chromosomal changes of a similar type involving the presence of a minute chromosome [Citation12]. Later, the small, unusual chromosome described by Nowell and Hungerford was given the name ‘Philadelphia chromosome’ after the city where it was discovered. By using improved Giemsa staining techniques, [Citation13] demonstrated that the shortened chromosome of Nowell and Hungerford was created due to reciprocal translocation of the long arms of human chromosome 9 and 22. The human ABL1 gene, which is a homologue of the Abelson murine leukemia virus oncogene (v–ABL) gene, is located on the band q34 on chromosome 9 and translocates to the band q11 on chromosome 22, where the breakpoint cluster gene (BCR) is located; translocation t(9;22)(q34;q11) thus effects the formation of the fusion gene called BCR–ABL1. Today, we know that the Philadelphia chromosome is the mark of chronic myeloid leukemia (CML), although it is often encountered in some other forms of leukemia [Citation14]. The formation of BCR-ABL1 transcript variants depends on the site of cleavage at the BCR gene.
There are different breakpoints in the BCR gene which span a DNA length of about 5–6 kb in the middle of the gene; this consequently leads to the formation of three different translated BCR/ABL1 phenotypes: P190, P210, and P230, with the numbers representing the weights of the hybrid proteins in kilo Dalton (kDa). The three proteins are associated with acute lymphocytic leukemia, chronic myeloid leukemia and chronic neutrophilic leukemia, respectively, although it is not well understood how these BCR – ABL1 proteins differ from each other in their signaling properties. A critical and most important aspect of cellular transformation induced by the BCR – ABL1 oncogene is activation of the tyrosine kinase feature of ABL1 in the BCR – ABL1 oncoprotein. CML patients usually have a break point in the major region of the BCR gene, between exons 13 and 15, which fuzes with exon 2 of the ABL gene, resulting in either b2a2 (e13a2) or b3a2 (e14a2) transcripts of the BCR-ABL1 gene encoding an oncoprotein with 210 kDa, i.e. fusion of either exon 13 (b2) or exon 14 (b3) of the BCR gene with the exon 2 (a2) of the ABL1 gene takes place, leading to the formation of either b2a2 (e13a2) or b3a2 (e14a2) oncogenes and resultant transcripts both of which produce a hybrid oncoprotein p210 [Citation15–17]. The Philadelphia chromosome, the break points involved, and the translocation process are indicated in and .
Figure 1. The Philadelphia chromosome [Citation18]. Philadelphia chromosome described by Nowell and Hungerford indicated by an arrow. BCR/ABL breakpoints: m-BCR happens after exon 1, µ-BCR after exon 19, and the M-BCR beyond exon 13 or exon 14; ABL break points happen after the 2nd exon of the gene. Fusion oncogenes/mRNA transcripts which result from the breakpoints.
![Figure 1. The Philadelphia chromosome [Citation18]. Philadelphia chromosome described by Nowell and Hungerford indicated by an arrow. BCR/ABL breakpoints: m-BCR happens after exon 1, µ-BCR after exon 19, and the M-BCR beyond exon 13 or exon 14; ABL break points happen after the 2nd exon of the gene. Fusion oncogenes/mRNA transcripts which result from the breakpoints.](/cms/asset/06c97b41-4539-4201-ad9b-760d8c9bcafb/yhem_a_2284038_f0001_oc.jpg)
Figure 2. Pictorial representation of translocation (9; 22) and the resultant BCR – ABL transcripts associated with CML, AML and ALL [Citation19].
![Figure 2. Pictorial representation of translocation (9; 22) and the resultant BCR – ABL transcripts associated with CML, AML and ALL [Citation19].](/cms/asset/0b063b33-70e8-4178-8659-d3408ac83648/yhem_a_2284038_f0002_oc.jpg)
The Philadelphia chromosome results in a chimeric oncogene in which the BCR and ABL1 genes are fuzed; a subsequent product of this oncogene is the expressed BCR/ABL1 protein which characteristically has elevated ABL1 tyrosine kinase activity. The pathogenesis of chronic myeloid leukemia (CML) is initiated by a reciprocal translocation which involves the long arms of chromosomes 9 and 22, t (9; 22) (q34; q11.2), to produce the Philadelphia chromosome. Blending of the Abelson gene (ABL1) on chromosome 9 with the breakpoint cluster region (BCR) on chromosome 22 leads to the formation of the BCR–ABL1 oncogene which encodes the BCR–ABL1 protein. This protein relocates itself to the cytoplasm of the cell and gets engaged in phosphorylation of multiple cellular substrates.
The protein is involved in the transformation of hematopoietic cells and brings into effect a multitude of cellular progressions such as reduction in growth factor dependence, enhanced viability, and changed adhesive properties of the chronic myelocytic leukemia (CML) cells. Through its enhanced tyrosine kinase activity, the protein activates downstream signal transduction and transformation processes. These processes include substrate phosphorylation induced downstream activation of various molecular pathways such as JAK/STAT, PI3 K/AKT, RAS/MEK, mTOR, Src kinases [Citation16]. A number of receptors of the hematopoietic growth factor are known to be directly linked to gene transcription through temporary activation of the Janus tyrosine kinase/signal transducer and activator of transcription protein (JAK/STAT) pathway, a chain of interactions between proteins in a cell which is involved in such cellular processes as immunity, cell division, cell death, and tumor formation. Janus tyrosine kinases (JAKs) are believed to show tyrosine kinase activity against activated receptors and signaling proteins including the signal transducer and activator of transcription proteins (STATs) [Citation20, Citation21]. Tyrosine phosphorylation of cytoplasmic STAT proteins leads to their multi – dimerization, movement to the nucleus, and transcriptional activation. Constitutive activation of JAK/STAT pathway has been demonstrated in CML cell lines with STAT1 and STAT5 being the major tyrosine – phosphorylated STATs in p210BCR–ABL1 containing cells [Citation22–24]. Another cellular process of importance in CML development and progression is the constitutive activation of the Phosphoinositide 3 – kinase/Serine/threonine kinase (PI3 K/AKT) pathway by BCR–ABL1 protein; this is an intracellular signaling pathway which is particularly involved in regulating the cell cycle there by having an effect on cellular quiescence, proliferation, cancer, and longevity. Phosphoinositide 3–kinases (PI3 K) activation is followed by phosphorylation and activation of the serine/threonine kinase (AKT) and its consequent movement to the plasma membrane. Activation of the anti–apoptotic N–terminal pleckstrin homology (PH) domain of AKT by PI3 K in BCR–ABL1 transformed cells is thought to play a major role in the cellular transformation process during CML development and progression [Citation25, Citation26].
The Ras (a guanosine nucleotide–binding protein) GTPase/Mitogen–activated extracellular signal–regulated kinase (Ras GTPase/MEK) pathway is a cellular pathway which consists of a kinase cascade which is subject to regulation through phosphorylation and dephosphorylation by specific protein molecules, including kinases, phosphatases, and various other proteins. Ras GTPase/MEK kinase pathway activation stimulates cell growth through a membrane receptor–binding network to induce transcription of various growth factor genes; it is known to be a vital cellular pathway which is usually deregulated in different cancers. In the case of CML, the BCR–ABL1 protein activates the Ras/MEK pathway by phosphorylating the growth factor receptor-bound protein 2/GRB2-associated Binding Protein 2 (Grb2/Gab2) pathway thereby stimulating cellular growth. The Ras/MEK pathway is frequently involved in sensitivity or resistance to the treatment of leukemia [Citation27–29]. The Sarcoma oncogene (Src)–family kinases (SFKs), which are none-receptor tyrosine kinases, are additional molecular classes of extensively studied downstream targets of the BCR–ABL1 oncoprotein. With their role mainly being extracellular signal–directed coordination of cell growth, differentiation and motility, they may contribute to disease progression by helping the BCR–ABL1 protein activate STAT5 and AKT proteins in the activation process of the JAK/STAT pathway [Citation30, Citation31]. The mammalian target of rapamycin (mTOR) pathway is another molecular signaling pathway that gets activated by BCR–ABL1 in CML cases. Mammalian target of rapamycin (mTOR) is a serine/threonine kinase that forms two different complexes, mTOR1 & mTOR2 and it has been shown that cells expressing mutated BCR–ABL genes bring about simultaneous inhibitions of mTORC1 and mTORC2, followed by apoptosis of cells, thereby curtailing tumorigenesis [Citation32]. The exhibited tyrosine kinase activity of the protein characteristically happens to be continuously up-regulated so that it can continue to activate these molecular pathways. Activation of these cellular and molecular processes is further accompanied by dysregulation of the adhesive, proliferative, transformational, and apoptotic features of hematopoietic cells [Citation33, Citation16]. The different cellular processes involved in CML pathogenesis are depicted in and .
Figure 3. BCR – ABL fusion gene oncogenesis leading to activation of different cellular processes and CML pathogenesis [Citation34].
![Figure 3. BCR – ABL fusion gene oncogenesis leading to activation of different cellular processes and CML pathogenesis [Citation34].](/cms/asset/329b0e6a-574a-4bc7-b366-422fc5bf8867/yhem_a_2284038_f0003_oc.jpg)
Figure 4. Molecular events leading to the expression of CML disease phenotype [Citation35].
![Figure 4. Molecular events leading to the expression of CML disease phenotype [Citation35].](/cms/asset/8683f49a-c668-4794-bbf3-0ba61475c9ef/yhem_a_2284038_f0004_oc.jpg)
The domains of the BCR-ABL1 oncoprotein that are involved in the development of CML are well identified and are found in the ABL1 and BCR proteins. The ABL1 protein domains are the Src 3-homology (SH3) domain, Src2-homology (SH2) domain, tyrosine kinase domain, DNA-binding domain, and actin-binding domain, while the BCR protein domains are the oligomerization domain and phoposerin/treonin-rich SH2 binding domain. It has been shown that a small binding domain can be used to control subcellular localization (nuclear or cytoplasmic), which may have implications for CML therapy. BCR – ABL1 is involved in causing CML when it is transported to the cytoplasm; its movement back to the nucleus leads to apoptosis. One area of research is to find ways to change the location of critical proteins to alter their function [Citation36, Citation37]. Different domains and amino acid residues of the BCR–ABL1 protein are shown in .
Figure 5. BCR–ABL1 domains and the targeting regions of the binding domains [Citation37]. Numbering indicates amino acid residue location of each domain in BCR–ABL1 (asterisk indicates approximate location).
![Figure 5. BCR–ABL1 domains and the targeting regions of the binding domains [Citation37]. Numbering indicates amino acid residue location of each domain in BCR–ABL1 (asterisk indicates approximate location).](/cms/asset/2c2838b7-2d6a-4b1c-aa03-6a592f85ed4f/yhem_a_2284038_f0005_oc.jpg)
Different experiments have been performed to assess the involvement of the BCR–ABL1 protein domains in cellular transformation processes which lead to the development of CML, and it has been shown that the transformation ability of these essential targets depends on the experimental system. For example, SH2 deletion mutants of BCR–ABL1 are defective for fibroblast transformation, but they retain the capacity to transform cell lines to factor independence and are leukemogenic in animals. Deletion or positional alteration of the ABL1 SH3 domain induces kinase activity. Abi-1 and Abi-2 (ABL interactor proteins 1 and 2) are two proteins which activate the inhibitory function of this SH3 domain. Another candidate inhibitor of ABL1 is the macrophage 23-kD stress protein (Msp23), which is a product of the pregnancy-associated glycoprotein (PAG) gene. When cells are exposed to conditions of oxidative stress, such as ionizing radiation, this small protein is oxidized and dissociates from ABL1. The kinase activity of ABL1 will then be turned on. Sometimes, the SH3 domain may bind internally to the proline–rich region in the center of the ABL1 protein, causing a conformational change that inhibits interaction with substrates. The merging of BCR sequences with the ABL1 SH3 domain nullifies the physiologic suppression of the kinase, which is thought to be the consequence of homo dimer formation; the N–terminal dimerization domain is, therefore, a crucial characteristic feature of the BCR–ABL1 protein but can be functionally replaced by other sequences that allow for dimer formation. It can then reasonably be deduced that deregulated tyrosine kinase activity is a unifying feature of chronic myeloproliferative disorders such as CML [Citation15]. Clinical management of CML involves the use of tyrosine kinase inhibitors (TKI). Imatinib mesylate was such a tyrosine kinase inhibitor which was approved for the first time to be utilized in the management of CML, with acceptable responses being reported in about 60% of patients, intolerance in nearly 20%, and drug resistance in nearly 20%. Newer TKIs’ with multiple options for patients in terms of enhanced potency and altered side effect profiles with relevant landmarks such as early molecular responses (3–6 months) and optimal molecular responses (12 months) have been reported recently [Citation34]. [Citation38] has reported that the response of CML patients to Imatinib Mesylate treatment varies according to BCR–ABL1 transcript type, with b2a2 transcript variant carriers having a higher response compared to b3a2 transcript variant carriers. Therefore, the identification of transcript type is essential to monitoring CML therapy.
3. Molecular monitoring of chronic myeloid leukemia, BCR – ABL1 test standardization & transcript quantitation
More than two decades have elapsed since previous CML management approaches such as the use of interferon–alfa (IFN–a), hydroxyurea, busulfan and allogenic hematopoietic stem cell transplantation (allo–HSCT) have been replaced by newer treatment options. Enhanced understanding of the molecular processes of CML pathogenesis has resulted in the innovation of these newer treatment options known as tyrosine kinase inhibitors (TKIs) which target different stages of the cellular transformation and cancer development process. The three TKIs that have been approved for the treatment of chronic-phase CML are imatinib, dasatinib, and nilotinib. The era of TKIs has brought the hope of long-term control of illness with simple oral therapy into reality. TKIs have thus been established as the gold standard treatment options for CML in the twenty-first century, with a reasonable number of patients who receive TKI therapy achieving promising molecular or treatment responses; untraceable or remarkably low levels of disease during treatment withdrawal have been reported in some patients. Allogenic hematopoietic stem cell transplantation (allo-HSCT) may be indicated when a satisfactory treatment response cannot be achieved with modern TKIs or in patients who do not respond well to TKIs in which case HSCT can be relied on for the hope of long–term survival. Among the novel therapeutic agents (TKIs), the treatment of CML with the tyrosine kinase inhibitor Imatinib has revolutionized targeted cancer therapy by altering the devastating malignancy of CML into a chronic condition which can be managed to a reasonable degree. Through this treatment option, it was possible to prove that a particular inhibition of the BCR–ABL1 fusion gene can effectively curtail the progression of CML, consequently resulting in pronounced early cytogenetic and molecular responses in most patients. Therefore, imatinib was established as an effective first – line therapy for CML during the revolutionary clinical trial ‘International Randomized Study of Interferon versus STI571 (IRIS)’ in which the prognostic importance of utilizing BCR–ABL1 mRNA transcript level measurement to assess therapeutic responses to CML was also verified. Hematologic, cytogenetic, and molecular tests can be used to monitor therapeutic responses in CML patients besides evaluating their clinical conditions [Citation39–41]. Hematology tests usually performed are an evaluation of the number of blood cells and a blood smear to determine the type of differentiation of white blood cells.
Patients are categorized into the complete hematologic response (CHR) group if the number of white blood cells & platelets is normal and no immature granulocyte cells are found in the blood smear [Citation42]. In CHR, CML could not be detected through hematologic tests but only through cytogenetic and molecular tests. Cytogenetic tests are performed to determine the number of white blood cells that carry the Philadelphia chromosome and would be done with a conventional cytogenetic test, i.e. karyotyping, or a more advanced cytogenetic test, which is fluorescence in situ hybridization (FISH) [Citation35]. Patients are considered to be in the minimal cytogenetic response group when the number of white blood cells carrying the Philadelphia chromosome is in the range of 66–95%; minor cytogenetic response if the number of white blood cells carrying the Philadelphia chromosome is in the range of 36–65%; partial cytogenetic response (PCyR) if the number of white blood cells carrying the Philadelphia chromosome is in the range of 1–35%; and complete cytogenetic response (CCyR) if no cells carrying the Philadelphia chromosome are found. Partial cytogenetic response (PCyR) and complete cytogenetic response (CCyR) are together called major cytogenetic response (MCyR). In CCyR, leukemic cells are not detected by using cytogenetic tests but by using molecular tests, which are performed to quantify the number of BCR–ABL1 mRNA transcripts by quantitative reverse transcriptase PCR (RT–qPCR). A BCR–ABL1 transcript level of <0.1% classifies patients as having a major molecular response (MMR), and if no BCR–ABL1 transcripts can be detected, patients are classified as having a complete molecular response (CMR) [Citation35, Citation42]. CML has three phases based on the pathogenesis process: the chronic phase, the acceleration phase, and the blast phase, or crisis phase. Most CML patients in the developed world are diagnosed in the chronic phase, with the median age at diagnosis being about 65 years, while CML is usually diagnosed during its blast phase in low – income countries, with the median age at diagnosis being 39 years. Patients diagnosed in the chronic phase are normally asymptomatic and are accidentally diagnosed with CML when they undergo usual blood testing for other purposes [Citation43, Citation44]. shows how response to treatment and minimal residual disease can be defined for patients diagnosed with chronic phase CML and treated with Imatinib.
Figure 6. Defining response to treatment and minimal residual disease for patients diagnosed with chronic phase CML, treated with Imatinib [Citation35].
![Figure 6. Defining response to treatment and minimal residual disease for patients diagnosed with chronic phase CML, treated with Imatinib [Citation35].](/cms/asset/601a2cae-fe41-4479-a0a5-65d058227e35/yhem_a_2284038_f0006_oc.jpg)
The molecular determination of BCR – ABL1 transcripts with real-time quantitative reverse transcriptase PCR (RT–qPCR, or RQ–PCR) can essentially be utilized to assess patient response, monitor minimal residual disease, and detect relapse in CML cases. Unexplained leukocytosis, which shows regularity, and Philadelphia chromosome detection by cytogenetic and molecular techniques settle the diagnosis of characteristic CML. Nevertheless, real-time quantitative reverse transcriptase polymerase chain reaction (RT–qPCR), which detects BCR–ABL1 transcript levels by amplifying the region around the fusion point between BCR and ABL1 genes, is an extremely sensitive test for minimal residual disease detection, and it is now being used as a standard method for molecular monitoring of CML [Citation45, Citation8, Citation6].
Molecular monitoring of BCR–ABL1 transcript levels in peripheral blood is the gold–standard monitoring strategy and a preferred method to the cytogenetic approach because of its higher sensitivity and less invasiveness. Molecular monitoring aids in rapid treatment intervention in treatment failure cases [Citation46]. BCR–ABL1 mRNA transcript quantitation by real-time PCR methods in blood specimens has been the principal molecular monitoring technique for CML phenotypes. Recent reports have hypothesized that early reduction of BCR–ABL1 transcript levels is predictive of cytogenetic response in chronic–phase CML cases. Testing laboratories are required to define their own criteria regarding the acceptable quality of RNA, test sensitivity, test precision, and significant transcript levels as RT–PCR techniques for BCR–ABL1 transcript quantitation are not standardized internationally; nonetheless, these methods can provide reliable and sensitive data if pertinently developed and if carried out with thorough attention to quality control (QC) issues. Such data are thought to be helpful in defining the levels of molecular response to therapy and identifying the patterns of response that provide an early indication of relapse and treatment resistance [Citation47]. Sequential measurement of BCR–ABL1 mRNA titers has been found to be reliable sensitivity- and specificity-wise to monitor the degree of response to therapy in CML patients and is used to define time – bound clinical markers and to assess the eligibility of cases for which treatment can be discontinued. An international scale (IS) for BCR–ABL1 transcript level determination has been implemented by testing laboratories worldwide to improve inter-laboratory comparability of test results. This can be done by making use of laboratory–specific conversion factors; kits and reagents calibrated to the First World Health Organization International Genetic Reference Panel for quantitation of BCR–ABL1 mRNA can also be used. BCR–ABL1 test standardization is an ongoing process as accurate and sensitive BCR–ABL1 transcript level determination remains technically challenging, although there has been undeniable progress over the past years [Citation48]. The international scale (IS) for BCR–ABL1 reverse transcriptase quantitative polymerase chain reaction (RT – q PCR) quantification was suggested in a CML meeting at the National Institutes of Health in Bethesda in October 2005, along with a number of endorsements for the harmonization of minimal residual disease (MRD) assessment.
The international scale (IS) is in essence identical to the measurement scale used in the International Randomized Study of Interferon versus STI571 (IRIS) study, with the IRIS standardized baseline being defined as 100% and the major molecular response (MMR) being a 3-log reduction relative to the standardized baseline defined as 0.1%
. A procedure for developing and endorsing laboratory-specific conversion factors (CFs) which can be used for the purpose of converting local values to IS values was initiated by the Adelaide Laboratory to enable testing laboratories to gain access to the IS scale. This enables testing laboratories to continue using their present assay conditions and expressing results according to local preferences besides expressing results on the IS. To guarantee the accuracy of BCR – ABL1 testing, testing laboratories are required to participate in standardization and external quality assessment programs in addition to establishing conversion factors or using calibrated kits for reporting on the IS. Testing laboratories are also required to determine how their assay results vary at high and low levels of illness thereby validating that their assay is adept to detecting molecular response (MR) in reasonable number of patient samples. As mentioned above, molecular detection of BCR – ABL1 transcript levels has been the most sensitive routine test which is utilized in monitoring CML treatment response. The transcript detection method makes use of reverse transcriptase quantitative polymerase chain reaction (RT–qPCR) to approximate the amount of BCR–ABL1 mRNA relative to an internal reference gene usually ABL1, GUSB, or BCR. Quantification of transcripts can be done by quantitative real–time PCR (qRT–PCR). Results are communicated on the International Scale (IS) as a percentage relative to the standardized baseline used in the fundamental IRIS (International Randomized Study of Interferon versus STI571) trial in which the tyrosine kinase inhibitor (TKI) imatinib was evaluated in CML patients. BCR – ABL1 testing is utilized to define molecular response (MR) to TKIs with a major molecular response (MMR) being defined as a 3 – log reduction from the standardized baseline which is
or 0.1%
. There is also a response category called deep molecular response, with the deep molecular responses (DMRs) of
,
and
being respectively defined as ≤0.01%, ≤0.0032%, and ≤0.001%
[Citation49, Citation50, Citation51]. Some CML patients may be Philadelphia chromosome (Ph)-positive by standard cytogenetic techniques, but typical b3a2 or b2a2 transcripts may not be detected, abnormally low levels of e13a2 or e14a2 transcript expression may be observed in other Ph-positive cases.
A search for an atypical BCR–ABL1 mRNA transcript variant should follow such clinical observations with specially designed PCR protocols, as atypical BCR–ABL1 fusion transcripts may not be efficiently detected by standard RT–qPCR methods. A multiplex PCR assay whereby BCR–ABL1 fusion genes are detected by resultant PCR product sizes generated based on BCR/ABL gene breakpoints which can be employed for the identification of atypical BCR–ABL1 fusion mRNA transcripts was described by [Citation52] for the first time. BCR–ABL1 mRNA values cannot be reported on the IS, nor can time–bound molecular indicators or treatment monitoring guidelines be applied for follow–up or treatment discontinuation in such cases. Nevertheless, molecular response rhythms can be defined as distinct molecular response (IMR) levels in relation to baseline BCR – ABL1 mRNA transcript expressions before the start of TKI therapy [Citation53].
4. Progresses made in molecular monitoring of chronic myeloid leukemia
Molecular monitoring plays a vital role in the clinical management of chronic myeloid leukemia (CML) patients, and it is being used as a tool to guide clinical decision-making. Quantitative reverse transcriptase polymerase chain reaction (RT–qPCR) assessment of BCR–ABL1 transcript levels has become a standard practice in CML management. Further developments have been found to be mandatory to judge the burden of leukemia more proficiently, to monitor minimal residual disease (MRD), to detect mutations that bring about resistance to tyrosine kinase inhibitor (TKI) therapy, and to identify clinical predictors of response to TKI therapy. Cartridge – based BCR – ABL1 quantitation, digital PCR and next-generation sequencing (NGS) are examples of technologies which have recently been assessed, evaluated and incorporated into clinical practice. Molecular tests for the evaluation of CML patients are made at diagnosis and at intervals of 3, 6 and 12 months after commencing TKI therapy. BCR–ABL1 transcript levels at these landmark time points are used by clinicians to define the degree of molecular response (MR). The European LeukemiaNet2013 recommendations define an optimal molecular response according to BCR–ABL1 transcript levels measured in IS as: <10% at 3 months, <1% at 6 months, and <0.1% from 12 months onwards. Patients with BCR–ABL1 transcript levels >10% at 6 months and >1% from 12 months on are categorized as being in the treatment failure group. BCR–ABL1 transcript levels between the optimal molecular response and treatment failure indicate a warning zone where more frequent molecular monitoring is recommended [Citation54, Citation55].
Modern developments in molecular monitoring of BCR–ABL1 transcript levels in CML cases are dedicated to addressing ongoing clinical challenges in CML, which are: (i) the development of efficient methods which facilitate fast, inexpensive and sensitive BCR–ABL1 transcript quantitation in emerging economic regions; (ii) the quantitation of low levels of BCR–ABL1 transcripts to assist in the detection and monitoring of minimal residual disease (MRD); (iii) the sensitive and accurate detection of mutations that bring about resistance to TKI therapy; and (iv) the discovery of molecular markers that predict response to therapy or disease progression. Advances in BCR–ABL1 transcript quantification, through which inexpensive and sensitive results on the IS can be efficiently obtained from various laboratories, will be advantageous to the molecular monitoring of CML in emerging economic regions. A cartridge–based automated RT–qPCR system, the Xpert BCR–ABL1 Monitor Assay can measure BCR–ABL1 p210 transcripts and has been developed by Cepheid (California, USA). The GeneXpert system utilizes microfluidics to directly process peripheral blood samples in a cartridge for RNA extraction, real-time RT-qPCR, and fluorescence detection in an ‘all-in-one’ self–contained instrument. The system has adopted reporting BCR-ABL1 transcript levels on the IS, whereby a conversion factor specific to the GeneXpert assay was developed and used to adjust the BCR–ABL/ABL1 ratio to the IS [Citation56, Citation57], but this test is still expensive and not affordable by many patients in low–income countries. Recently developed molecular tests for the detection of BCR–ABL1 transcripts in CML patients include digital PCR (d PCR), DNA PCR and droplet digital PCR (dd PCR). d PCR is a very sensitive method and has the characteristic benefit of avoiding certain limitations encountered in conventional RT-qPCR. Therefore, its value for monitoring BCR–ABL1 mRNA transcript levels in CML patients undergoing TKI therapy has been appreciated [Citation58].
5. Assessment of BCR – ABL transcript variants detection, occurrence, and association with CML phenotypes/hematological features
The majority of CML patients express either the b3a2 or b2a2 BCR–ABL1 transcript types; both transcripts can be expressed in about 5–10% of patients due to alternative splicing. There are varied arguments derived from various studies on the effect of BCR – ABL transcript types on disease characteristics; it is believed that much of the disagreement between the findings of such studies may be attributed to differences in sample selection [Citation59].
Generally, it can reasonably be argued that assessment of the effect of BCR–ABL1 transcript types on CML phenotypes is an issue of concern, as data obtained from such associations may enrich the clinical knowledge of the disease, consequently aiding in its proper or better management. A study conducted at Ahmed Ben Bella University of Oran, Algeria, in 2020 assessed the impact of the major BCR–ABL1 transcript types on clinical and biological parameters in patients with chronic myeloid leukemia. The occurrence of major BCR–ABL1 transcripts and the clinical or hematological characteristics obtained in each type of major transcript in patients with newly diagnosed chronic phase (CP)-CML were assessed in this study. In this study, molecular transcript typing was performed by multiplex reverse transcriptase polymerase chain reaction, followed by quantification of transcripts by real–time quantitative polymerase chain reaction. Among the 67 patients included in the study, 41 (61%) patients expressed the b3a2 transcript, 24 (35.82%) patients expressed the b2a2 transcript, and two (2.98%) patients expressed a rare transcript type, e19a2. The b3a2 transcript was found to be significantly more frequent in women than the b2a2 transcript, and patients expressing b2a2 appeared to have a higher mean leukocyte count, indicating a greater tumor charge than those expressing b3a2. No difference was observed in the average number of platelets or hemoglobin concentration among patients expressing different transcripts [Citation51]. Another study was also conducted in the same year at King Edward Medical University, Lahore, Pakistan, on CML patients to measure the occurrence of b3a2, b2a2 and e1a2 transcripts and to determine their relationship with clinical and biological variables like blood count, age and gender. The study also made a comparison of whole blood and plasma samples for BCR–ABL1 mRNA extraction and detection. Blood cell counts, including total leukocyte count (TLC), hemoglobin (Hgb) and platelet count (PC), were recorded. Real-time PCR was employed in this study to detect BCR–ABL1 transcript types and separate amplification reactions for b2a2, b3a2 and e1a2 transcripts were carried out. Specific primers with the same PCR components were used in these reactions. The transcript b3a2 was detected in 32 (66.66%) of 48 patients, and b2a2 was detected in 10 (32.10%) of the patients. No co-expression of transcripts was observed in all patients, and no e1a2 transcript was detected. No significant correlation was found between transcript type and any of the hematological parameters. There was no significant correlation between transcript type and gender or age. BCR-ABL/G6PD ratios in peripheral blood cells were higher than those in plasma.
This study concluded that plasma can be used as an alternative to blood cells for BCR – ABL1 quantification and that transcript types cannot be easily explained by clinical factors or CML phenotypes [Citation6]. BCR–ABL1 transcript types of CML patients in Dr. Sardjito General Hospital, Yogyakarta, Indonesia, were analyzed using RT – PCR in 2020. 185 patients were enrolled in this study, out of which (99%) were BCR-ABL1 positive, with the most common transcript type being b3a2 (136/183; 74.3%), followed by b2a2 (41/183; 22.4%). Two samples (1.1%) showed co-expression of b3a2 and b2a2, and three samples showed uncommon fragments. Contrasting results were reported by a study made in Ecuador, indicating that nearly 95% of CML patients with BCR–ABL1 express the b2a2 transcript type and only 5.4% are positive for the b3a2 transcript type. These differences in the occurrence of transcript types may be attributed to different genetic backgrounds in the different populations. Assessment of the BCR – ABL1 transcript variants and their frequencies in different ethnic groups is needed since the BCR–ABL1 transcript type in CML is highly correlated to the clinical course and outcome of the patients [Citation60, Citation61]. A cross-sectional study was conducted in 2021 on patients from a tertiary care hospital in Rawalpindi in the laboratory settings of Riphah International University in Pakistan with the aim of detecting and analyzing the demographic and hematological characteristics according to the variants of the BCR-ABL1 transcripts in CML patients. The study used reverse transcriptase (RT)-PCR by choosing a primer specific for exon b1 (e12) on the BCR gene and a reverse primer on ABL1 exon 2 for the detection of various transcripts. This approach gives the advantage of simplifying and shortening the procedure of RT–PCR for detection of these transcripts in a single reaction by eliminating the need for independent sequence–specific primers, which increases the complexity, workload, and cost of PCR. According to this study, the predominant transcript in CML patients was e14a2 (b3a2) . The co-expression of e13a2/e14a2 transcripts was demonstrated in only two patients (3%) of the 70 patients analyzed, and the clinical features and phenotypes of the patients with the two groups of typical transcripts were not found to be plainly different. All the CML patients (70) included in this study possessed characteristic BCR-ABL1 transcripts, with the dominant e14a2 (b3a2) transcript variant. The group of patients with the e13a2 (b2a2) transcript had a significantly higher mean leukocyte count compared to those with the e14a2 (b3a2) variant. Considering the gender of patients, male prevalence was observed in e14a2 (b3a2) and female preponderance in e13a2 (b2a2) transcripts.
The difference in leukocyte counts and gender association in patient groups possessing the two transcripts might suggest a distinct pathobiology and phenotype of disease, according to this study [Citation62]. In another study conducted in 2020, a multiplex PCR assay was employed to detect BCR–ABL1 transcripts in a retrospective descriptive and analytical study of adult CML patients in Srinagarind hospital, KhonKaen University, Northeastern Thailand with the aim of reporting BCR–ABL1 fusion transcript types in different CML phenotypes. Data were collected from medical records, and peripheral blood or bone marrow samples were analyzed by multiplex PCR from 177 adult CML patients. All patients involved in the study were positive for some type of BCR–ABL1 transcript, with the majority of the patients (93.79%) expressing one of the typical BCR–ABL1 transcripts (b3a2/b2a2). b3a2 and b2a2 transcript types were detected in 58.19% and 35.59%, respectively, and co-expression of b3a2/e1a2 was detected in 0.56%. The expression of an e1a2 transcript type, which codes for a p190 protein, was found to be 4.52%, and a 1.14% expression rate was observed for the e19a2 transcript type. Co-expression of p210/p190 was not detected. Multiplex RT–PCR is useful and saves time in the detection of BCR–ABL1 fusion transcript types. The primer combinations in multiplex RT–PCR allow simultaneous detection of all known types of BCR/ABL and BCR transcripts in one reaction. This study was able to reliably detect the typical p210 transcripts, b2a2 or b3a2 with the more prevalent transcript in CML patients being b3a2 and atypical ones such as transcripts lacking ABL exon a2 (b2a3 and b3a3), or p190 BCR–ABL1 transcripts, such as e1a2 [Citation10]. A study was conducted in Tunisia to report the frequencies of major BCR–ABL1 fusion mRNA transcripts in 44 CML patients and to assess the association between the type of transcripts and biological or hematological features. The BCR-ABL fusion transcript determination was made by reverse transcriptase polymerase chain reaction (RT–PCR). 64% of the patients expressed the transcript b3a2, while the rest expressed the b2a2 transcript. No co-expression of b3a2/b2a2 transcripts was observed. Analysis of the association between transcript type and biological variables showed that the identified major transcripts were not statistically related to the sex of patients; the b3a2 transcript type was expressed in older patients compared with the b2a2 transcript; and the major BRC–ABL1 transcripts were not related to white blood cell (WBC) and hemoglobin level measurements.
On the contrary, the number of platelets was found to be significantly higher in patients expressing the b3a2 transcript compared with those expressing the b2a2 transcript (P = 0.001) [Citation63]. P-glycoprotein, also called P-gp is an efflux transporter protein which is coded by the multidrug resistance protein 1 (MDR1) gene. This protein functions by limiting the dispersal of drug substrates into various organs. Enhanced expression of the MDR1 gene leads to resistance of cancer cells to the cytotoxic effects of several anti-cancer agents such as anthracyclines, epipodo-phyllotoxins, vinca alkaloids, etc., and it has been reported as the commonest cause of multi-drug resistance in different cancers, probably leading to a poor prognosis. [Citation64] examined the expression of the MDR1 gene in 98 untreated chronic phase-CML patients bearing different BCR-ABL1 mRNA transcript types and associated the results with various hematologic features of the patients. Transcript typing and MDR1 gene expression status were performed by RT–PCR. 53 (54%) patients expressed the b3a2 transcript, and the b2a2 transcript was expressed in 44 (45%) of the patients. The MDR1 gene amplification pattern exhibited variable intensity in the CML patients, ranging from negative to strongly positive PCR reactions: over–expression of the MDR1 gene (MDR1+) indicated by moderate to strong positive reactions was found in 48 (49.5%) patients, and a normal level of MDR1 expression (MDR1−) indicated by no product of MDR1 amplification in RT–PCR was found in 49 (50.5%) patients. Wilcoxon Mann–Whitney test was used to associate biological or hematological features in patients expressing b3a2 & b2a2 transcript types, as well as between categories of patients with normal & raised levels of MDR1 gene expression. Higher platelet counts in patients expressing b3a2 transcripts compared to those expressing b2a2 transcripts were observed only in the category of patients exhibiting over-expression of the MDR1 gene. Besides, patients exhibiting over-expression of the MDR1 gene and expressing the b3a2 transcript had lower white blood cell (WBC) counts compared to those expressing b2a2 transcripts and exhibiting MDR1 gene over–expression. More than 95% of CML patients are diagnosed with the e13a2 or e14a2 BCR–ABL1 fusion transcripts. The rest, about 5% of CML patients, are diagnosed with rare, atypical BCR-ABL1 fusion transcripts such as e19a2, e8a2, e13a3, e14a3, e1a3 and e6a2, which result from chromosome breakpoints happening outside the commonly encountered ABL or BCR breakpoint regions. Such atypical BCR–ABL1 mRNA transcripts may not be detected by PCR protocols that are optimized to detect the typical transcripts.
Multiplex PCR assays which can detect typical and atypical BCR-ABL1 mRNA transcripts may have to be developed in such cases. The prevalence of such uncommon BCR –ABL1 fusion mRNA transcripts in patients with CML was analyzed in a study conducted in China. BCR–ABL1 transcripts were detected by multiplex RT-qPCR and RT–qPCR from peripheral blood (PB) or bone marrow (BM) samples. A total of 4750 CML patients underwent BCR–ABL1 fusion transcript detection, of which 4667 (98.3%) patients had e13a2/e14a2 (P210) BCR–ABL1 fusion transcripts. 83 (17%) of patients in this study had uncommon BCR–ABL1 transcripts, of which the three most frequent transcript types were e19a2, e13a3/e14a3, and e1a2, which accounted for 39.8%, 20.5%, and 16.9% of cases with uncommon BCR–ABL1 transcripts, respectively. These generally accounted for 3/4 of the cases with uncommon BCR–ABL1 transcripts. Sequence characteristics of uncommon BCR–ABL1 transcripts using Sanger sequencing showed the presence of 19 types of uncommon BCR–ABL1 fusion transcripts in total, all of which were in–frame, and 13 of these had never been reported before [Citation65, Citation66, Citation67]. BCR–ABL1 transcripts which are commonly detected from CML patients are b2a3 and b2a2 although other transcripts rarely detected. The above study reports on BCR–ABL1 transcript types and associated biological/hematological features, summarized in .
Table 1. Summary of study reports on BCR – ABL transcript types and their association with hematological phenotypes/biological features.
6. The impact of different transcript variants on achieving different types of response in patients taking various tyrosine kinase inhibitors
Most laboratories do not discriminate between b2a3 and b2a2 BCR–ABL1 mRNA transcripts when performing minimal residual disease (MRD) studies, as they use the same set of PCR primers to detect both fusion mRNA transcripts. Evolving studies have conjectured that molecular response variations among CML patients may partly be attributed to variances in the amplification efficiency of the two major transcripts by standard RT-qPCR techniques, which use the same primer sets; larger e14a2 (b3a2) mRNA transcripts may be amplified with less efficiency, resulting in a seemingly superior response [Citation53]. Identification of BCR–ABL1 transcript variants is beneficial both to monitor disease carefully and to select additional treatment regimens with specific transcripts when needed. The achievement of a major molecular response (MMR), a 3-log reduction in BCR-ABL1 mRNA transcripts (0.1% BCR–ABLIS) during TKI therapy, is a vital breakthrough in CML management and signifies a good patient prognosis. A 4 log reduction of BCR–ABL1 mRNA transcripts (MR4 or < 0.01 BCR–ABLIS) is referred to as deep molecular response (DMR) and is usually considered the minimum depth of response required to ponder a trial of ‘treatment-free remission’ (TFR), a response category where a patient who has discontinued TKI therapy maintains a major molecular response (MMR) and does not need to restart treatment [Citation62, Citation68]. Several investigations have assessed the impact of BCR – ABL1 major transcript types on achieving different types of molecular responses in patients who are under TKI therapy, besides their effect on the CML phenotypes, and have come up with varied results. The impact of the major BCR – ABL1 transcript type on achieving various molecular/treatment responses in patients with chronic myeloid leukemia treated with imatinib as first–line therapy was assessed at Ahmed Ben Bella University of Oran, Algeria, in 2020. The major molecular response (MMR) and deep molecular response (MRD) obtained in each type of major transcript in patients with CML treated with imatinib (IM) as frontline therapy were evaluated. Statistical association with molecular responses showed that patients with a b3a2 transcript exhibited a better major molecular response (MMR) than patients with a b2a2 transcript at 18 months post-imatinib therapy, and the cumulative probability of achieving a deep molecular response (DMR) at 5 years was higher in patients with a b3a2 type but not statistically significant.
This study concluded that patients with the b3a2 transcript may have a better response to imatinib therapy [Citation51]. An analysis of patients diagnosed with CP–CML & treated with different TKIs was conducted to assess the degree of molecular response to treatment among different BCR–ABL transcripts. The complete cytogenetic response (CCyR) and major molecular response (MMR) achievements were similar among the different transcripts (P = 0.921 and P = 0.489, respectively) in this study, while the deep molecular response (DMR) and sustained deep molecular response (sDMR) were found to be significantly lower for the transcript e13a2 (b2a2) (P = 0.008 and P = 0.003, respectively). A DMR (MR4.0) was achieved by 82.2% of patients harboring the e14a2 (b3a2) transcript and by 52.4% of patients harboring the e13a2 (b2a2) transcript; an sDMR was achieved by 47.2% of patients harboring the e14a2 (b3a2) transcript and by only 26.9% of those harboring the e13a2 (b2a2) transcript at 60 months. The effect of various first–line TKIs on disease response was also assessed in the patients with the different transcripts. The achievement of an sDMR was found to be significantly slower in patients who were treated with imatinib as frontline therapy compared with those who received frontline second–generation TKIs (2GTKIs). Sustained deep molecular response (sDMR) is defined as a stable deep molecular response (MR4.0) for a minimum of 2 years. Expression of the e14a2 transcript was observed in 82% of patients, and e14a2/e13a2 co-expression was observed in 18% of patients who discontinued TKI therapy. This study concluded that the e13a2 (b2a2) transcript impedes the achievement of deep molecular responses and the prospect of stopping TKI treatment in CML patients [Citation69]. A study was conducted at the MD Anderson Cancer Center, Madrid, Spain, in patients with chronic phase CML recruited for clinical trials using TKI as front-line therapy from July 31, 2000, to September 10, 2013, to assess the impact of BCR-ABL1 transcript types on treatment outcome and the wellness predictive pertinence of frequently expressed BCR–ABL1 transcripts in patients treated with 4 TKI agents. 481 patients expressing e13a2, e14a2, and co-expressing both (e13a2/e14a2) transcripts were included in the study and treated with imatinib 400 mg daily (n = 69), imatinib 800 mg daily (n = 199), dasatinib 50 mg twice daily or 100 mg daily (n = 105), or nilotinib 400 mg twice daily (n = 108); the cytogenetic and molecular responses according to transcript type and the TKI agent administered were assessed.
CCyR rate was 89% for e13a2 transcript, 94% for e14a2 transcript, and b3a2/b2a2 co-expression, but the association was not statistically significant; MMR rate was 79% for e13a2 transcript, 91% for e14a2 transcript, and 95% for b3a2/b2a2 co-expression (P = 0.0001); and MR4.5 was 57% for e13a2, 79% for e14a2, and 80% for b3a2/b2a2 co-expression (P = 0.00001). Patients expressing e13a2 transcript and treated with imatinib 400 mg daily exhibited a lower CCyR rate (77%) compared with those who received other TKIs (90–95%); the CCyR rate with imatinib 400 mg daily was similar to other TKI regimens (93–96%) in patients with e14a2 or with co-expressed b3a2/b2a2 transcript carriers. Similarly, patients expressing the e13a2 transcript and treated with imatinib 400 mg daily exhibited lower MMR and MR4.5 rates compared with those who were treated with other TKI regimens. Similar MMR and MR4.5 rates were observed in all TKI regimens for patients with e14a2 transcripts. Nilotinib therapy was associated with inferior MR4.5 rates in either e13a2 or e14a2 transcript carriers compared with imatinib 800 mg or dasatinib therapy. The CCyR and MCyR rates for the e13a2 transcript carriers persisted at 60 months in this study [Citation70]. Twenty–four transcripts were found in 21 patients in a study made in Syria with the aim of identifying the frequency of different BCR–ABL1 transcripts in CML patients and assessing their impact on monitoring and treatment procedures. The typical BCR–ABL1 transcript b3a2 was found to be statistically significantly associated with warning molecular response to imatinib therapy (P = 0.047) in the study compared with b2a2, b3a3, and e1a3 transcripts; the b3a2 transcript is usually observed in patients who have not reached MMR [Citation71]. In addition to p210 transcripts, clinicians may have to address atypical, rare BCR–ABL1 transcripts while treating CML patients, although there is no adequate evidence of these infrequent oncogenic variants regarding their clinical and prognostic roles on which inferences can be built for the management of CML patients enrolled in tyrosine kinase inhibitors (TKIs). The association between such uncommon transcript variants and molecular responses was analyzed in CML patients treated with Imatinib in China, and it was shown that CML patients with e19a2 and e1a2 uncommon transcript types had significantly reduced probabilities of a 1–year complete cytogenetic response (CCyR) and major molecular response (MMR). Patients with e13a3 and e14a3 uncommon transcript variants had significantly increased probabilities of 1–year CCyR and MMR compared with CML patients in chronic phase with common e13a2/e14a2 transcripts receiving frontline imatinib therapy.
It was also shown that patients with the e19a2 transcript had low probabilities of 2–year event–free survival (EFS) and progression–free survival (PFS); patients with the e1a2 transcript also had low probabilities of 2–year EFS compared with CML patients with common transcript variants receiving imatinib (IM) therapy. Generally, uncommon BCR–ABL1 fusion transcripts are rare and diverse in patients with CML and may be relevant for TKI therapy outcomes. Event–free survival (EFS) is defined as the time elapsed between the commencement of TKI therapy and the appearance of one of the following events: the absence of hematological response at 3 months; the loss of previously achieved complete hematological response (CHR), major cytogenetic response (MCyR) or complete cytogenetic response (CCyR); progression to accelerated phase (AP) or blast phase (BP); death from any cause; or the end of follow–up; while progression–free survival (PFS) is defined as the time elapsed between the commencement of TKI therapy and progression to AP or BP; death from any cause; or the end of follow – up in the study [Citation66, Citation67]. The above study reports on BCR–ABL1 transcript types and their impact on achieving different types of responses in patients receiving TKIs, as summarized in .
Table 2. Summary of study reports on BCR – ABL transcript variants and their impact on achieving different types of response in patients taking various tyrosine kinase inhibitors.
7. Conclusion
CML is a devastating disease which predisposes patients to unwanted medical costs, a low quality of life, and a shortened life expectancy. The etiologic agent of the disease is mainly the oncogene BCR-ABL1, which is a result of chromosomal translocation between segments of chromosomes 22 & 9. This oncogene codes for a BCR–ABL1 oncoprotein which has high tyrosine kinase activity, thereby activating many malignant cellular pathways. Recent developments in the treatment of CML through molecular monitoring of the disease have managed to reduce morbidity and mortality. Advanced molecular techniques are aimed at detecting BCR–ABL transcript levels to monitor treatment response (molecular responses) with the transcript levels. Transcript typing is necessary to detect minimal residual disease and achieve a molecular response by helping to provide selective therapy. CML diagnosis and follow–up are beyond the edge in the developed world but by far untouched in developing countries like Ethiopia. Therefore, cheaper genetic tests for BCR–ABL1 transcript profiling of CML patients are required to aid in the diagnosis and management of CML cases, as clinical management alone may not be effective.
Disclosure statement
No potential conflict of interest was reported by the author(s).
References
- Apperley JF. Chronic myeloid leukaemia. Lancet. 2015;385(9976):1447–1459.
- Tadwalkar S. The global incidence and prevalence of chronic myeloid leukemia over the next ten years (2017–2027). J Blood DisordTransfus. 2017;8:2155–2164.
- Khazaal MS, Hamdan FB, Al-Mayah QS. Association of BCR/ABL transcript variants with different blood parameters and demographic features in Iraqi chronic myeloid leukemia patients. Mol Genet Genomic Med. 2019;7(8):e809.
- Davis RL, Konopka JB, Witte ON. Activation of the C – ABL oncogene by viral transduction or chromosomal translocation generates altered C – ABL proteins with similar in vitro kinase properties. Mol Cell Biol. 1985;5(1):204–213.
- Bauer S, Romvari E. Interpreting molecular monitoring results and international standardization in chronic myeloid leukemia. J Adv Pract Oncol. 2012;3(3):151.
- Javed A, Mukhtar H, Kubra K, et al. Detection of BCR–ABL fusion gene and its transcript variants in chronic myeloid leukaemia patients–a multi – comparison study. JPMA. 2020;70(1748):10–5455.
- Bavaro L, Martelli M, Cavo M, et al. Mechanisms of disease progression and resistance to tyrosine kinase inhibitor therapy in chronic myeloid leukemia: an update. Int J Mol Sci. 2019;20(24):6141.
- Bennour A, Saad A, Sennana H. Chronic myeloid leukemia: relevance of cytogenetic and molecular assays. Crit Rev Oncol Hematol. 2016;97:263–274.
- Sholikah, T.A. (2017). Fusion gene BCR-ABL: from etiopathogenesis to the management of chronic myeloid leukemia. JKKI: Jurnal Kedokteran dan Kesehatan Indonesia: 29-37.
- Chootawiriyasakul K, Chansung K. Multiplex PCR for identifying BCR-ABL fusion transcript types in Northeastern Thailand chronic myeloid leukemia patients. Srinagarind Med J. 2020;35(6):720–725.
- Dong Y, Shi O, Zeng Q, et al. Leukemia incidence trends at the global, regional, and national level between 1990 and 2017. Exp Hematol Oncol. 2020;9(1):1–11.
- Nowell PC, Hungerford DA. Chromosome studies on normal and leukemic human leukocytes. J Natl Cancer Inst. 1960;25(1):85–109.
- Rowley JD. A story of swapped ends. Science. 2013;340(6139):1412–1413.
- Haider MZ, Anwer F. Genetics, Philadelphia chromosome [Updated 2023 July 17]. In: Statpearls [internet]. Treasure Island (FL): StatPearls Publishing; 2023 January. Available from: https://www.ncbi.gov/books/NBK560689.
- Deininger MW, Goldman JM, Melo JV. The molecular biology of chronic myeloid leukemia. Blood J Am Soc Hematol. 2000;96(10):3343–3356.
- Sattler M, Griffin JD. Mechanisms of transformation by the BCR/ABL oncogene. Int J Hematol. 2001;73(3):278–291.
- Kang ZJ, Liu YF, Xu LZ, et al. The Philadelphia chromosome in leukemogenesis. Chin J Cancer. 2016;35(1):1–15.
- Radich JP. Another Philadelphia story. Haematologica. 2022;107(3):566.
- Avelino KY, Silva RR, da Silva Junior AG, et al. Smart applications of bionanosensors for BCR/ABL fusion gene detection in leukemia. J King Saud Univ-Sci. 2017;29(4):413–423.
- Ihle JN, Thierfelder W, Teglund S, et al. Signaling by the cytokine receptor superfamily a. Ann N Y Acad Sci. 1998;865(1):1–9.
- Aaronson DS. Horvath CM. A road map for those who don’t know JAK-STAT. Science. 2002;296:1653–1655.
- Carlesso N, Frank DA, Griffin JD. Tyrosyl phosphorylation and DNA binding activity of signal transducers and activators of transcription (STAT) proteins in hematopoietic cell lines transformed by BCR/ABL. J Exp Med. 1996;183(3):811–820.
- Shuai K, Halpern J, Ten Hoeve J, et al. Constitutive activation of STAT5 by the BCR-ABL oncogene in chronic myelogenous leukemia. Oncogene. 1996;13(2):247–254.
- Bromberg J, Darnell JE. The role of STATs in transcriptional control and their impact on cellular function. Oncogene. 2000;19(21):2468–2473.
- Skorski T, Bellacosa A, Nieborowska-Skorska M, et al. Transformation of hematopoietic cells by BCR/ABL requires activation of a PI-3k/AKT-dependent pathway. EMBO J. 1997;16(20):6151–6161.
- King D, Yeomanson D, Bryant HE. PI3king the lock: targeting the PI3 K/AKT/mTOR pathway as a novel therapeutic strategy in neuroblastoma. J Pediatr Hematol Oncol. 2015;37(4):245–251.
- Puil L, Liu JIAXIN, Gish G, et al. BCR-ABL oncoproteins bind directly to activators of the Ras signalling pathway. EMBO J. 1994;13(4):764–773.
- Chu S, Li L, Singh H, et al. BCR-tyrosine 177 plays an essential role in Ras and AKT activation and in human hematopoietic progenitor transformation in chronic myelogenous leukemia. Cancer Res. 2007;67(14):7045–7053.
- Steelman LS, Franklin RA, Abrams SL, et al. Roles of the Ras/Raf/MEK/ERK pathway in leukemia therapy. Leukemia. 2011;25(7):1080–1094.
- Kim LC, Song L, Haura EB. Src kinases as therapeutic targets for cancer. Nat Rev Clin Oncol. 2009;6(10):587–595.
- Chereda B, Melo JV. Natural course and biology of CML. Ann Hematol. 2015;94(2):107–121.
- Airiau K, Mahon FX, Josselin M, et al. Pi3 K/mTOR pathway inhibitors sensitize chronic myeloid leukemia stem cells to nilotinib and restore the response of progenitors to nilotinib in the presence of stem cell factor. Cell Death Dis. 2013;4(10):e827–e827.
- Bedi A, Zehnbauer BA, Barber JP, et al. Inhibition of apoptosis by BCR –ABL in chronic myeloid leukemia. Blood. 1994;83(8):2038–2044.
- Pophali PA, Patnaik MM. The role of new tyrosine kinase inhibitors in chronic myeloid leukemia. Cancer J (Sudbury, Mass.). 2016;22(1):40.
- Frazer R, Irvine AE, McMullin MF. Chronic myeloid leukaemia in the 21st century. Ulster Med J. 2007;76(1):8.
- Salesse S, Verfaillie CM. BCR/ABL: from molecular mechanisms of leukemia induction to treatment of chronic myelogenous leukemia. Oncogene. 2002;21(56):8547–8559.
- Dixon AS, Constance JE, Tanaka T, et al. Changing the subcellular location of the oncoprotein BCR-ABL using rationally designed capture motifs. Pharm Res. 2012;29(4):1098–1109.
- Sharma P, Kumar L, Mohanty S, et al. Response to imatinib mesylate in chronic myeloid leukemia patients with variant BCR –ABL fusion transcripts. Ann Hematol. 2010;89(3):241–247.
- Valent P, Lion T, Wolf D, et al. Diagnostic algorithms, monitoring, prognostication, and therapy in chronic myeloid leukemia (CML): a proposal of the Austrian CML platform. Wiener KlinischeWochenschrift. 2008;120(21):697–709.
- Tanaka MF, Kantarjian H, Cortes J, et al. Treatment options for chronic myeloid leukemia. Expert Opin Pharmacother. 2012;13(6):815–828.
- Innes AJ, Milojkovic D, Apperley JF. Allogeneic transplantation for CML in the TKI era: striking the right balance. Nat Rev Clin Oncol. 2016;13(2):79–91.
- Goldman JM. How I treat chronic myeloid leukemia in the imatinib era. Blood J Am Soc Hematol. 2007;110(8):2828–2837.
- Perrotti D, Jamieson C, Goldman J, et al. Chronic myeloid leukemia: mechanisms of blastic transformation. J Clin Invest. 2010;120(7):2254–2264.
- Mendizabal AM, Garcia-Gonzalez P, Levine PH. Regional variations in age at diagnosis and overall survival among patients with chronic myeloid leukemia from low- and middle-income countries. Cancer Epidemiol. 2013;37(3):247–254.
- Zhen C, Wang YL. Molecular monitoring of chronic myeloid leukemia: international standardization of BCR–ABL1 quantitation. J Mol Diagn. 2013;15(5):556–564.
- Fernandes A, Shanmuganathan N, Branford S. Genomic mechanisms influencing outcome in chronic myeloid leukemia. Cancers (Basel). 2022;14(3):620.
- Hughes T, Branford S. Molecular monitoring of chronic myeloid leukemia. In: Seminars in hematology. WB Saunders; 2003. 40: p. 62–68.
- Salmon M, White HE, Cross NC, et al. Standardization of molecular monitoring for chronic myeloid leukemia: 2021 update. In: Chronic myeloid leukemia. Cham: Springer; 2021. p. 105–117.
- White HE, Matejtschuk P, Rigsby P, et al. Establishment of the first World Health Organization international genetic reference panel for quantitation of BCR-ABL mRNA. Blood J Am Soc Hematol. 2010;116(22):e111–e117.
- Cross NC, White HE, Evans PA, et al. Consensus on BCR–ABL 1 reporting in chronic myeloid leukaemia in the UK. Br J Haematol. 2018;182(6):777–788.
- Nachi M, Kihel I, Entasoltane B, et al. Impact of the major BCR–ABL1 transcript type on clinical and biological parameters and molecular response in patients with chronic myeloid leukemia. Hematology/Oncology and Stem Cell Therapy. 2022.15:Iss.2, Article 9.
- Cross NC, Melo JV, Feng L, et al. An optimized multiplex polymerase chain reaction (PCR) for detection of BCR-ABL fusion mRNAs in haematological disorders. Leukemia. 1994;8(1):186–189.
- Cross NC. Importance of BCR/ABL1 transcript type in CML. Clin Lymphoma Myeloma Leukemia. 2021;21:S20–S22.
- Baccarani M, Deininger MW, Rosti G, et al. European LeukemiaNet recommendations for the management of chronic myeloid leukemia: 2013. Blood, J Am Soc Hematol. 2013;122(6):872–884.
- Marum JE, Branford S. Current developments in molecular monitoring in chronic myeloid leukemia. Ther Adv Hematol. 2016;7(5):237–251.
- Dufresne SD, Belloni DR, Levy NB, et al. Quantitative assessment of the BCR –ABL transcript using the Cepheid Xpert BCR –ABL monitor assay. Arch Pathol Lab Med. 2007;131(6):947–950.
- Enjeti A, Granter N, Ashraf A, et al. A longitudinal evaluation of performance of automated BCR –ABL1 quantitation using cartridge-based detection system. Pathology. 2015;47(6):570–574.
- Kongruang A, Limsuwanachot N, Magmuang S, et al. Committed change of real-time quantitative PCR to droplet digital PCR for monitoring BCR–ABL1 transcripts in tyrosine kinase inhibitor treated CML. Hematology. 2023;28(1):2256199.
- Melo JV. 2 BCR-ABL gene variants. Baillière’s Clin Haematol. 1997;10(2):203–222.
- Hanfstein B, Lauseker M, Hehlmann R, et al. Distinct characteristics of e13a2 versus e14a2 BCR-ABL1 driven chronic myeloid leukemia under first-line therapy with imatinib. Haematologica. 2014;99(9):1441.
- Paramita DK, Hutajulu SH, Syifarahmah A, et al. BCR-ABL gene transcript types of patients with chronic myelogenous leukemia in Yogyakarta, Indonesia. Asian Pac J Cancer Prev: APJCP. 2020;21(6):1545.
- Amin H, Ahmed S. Characteristics of BCR–ABL gene variants in patients of chronic myeloid leukemia. Open Med. 2021;16(1):904–912.
- Bennour A, Ouahchi I, Achour B, et al. Analysis of the clinico-hematological relevance of the breakpoint location within M-BCR in chronic myeloid leukemia. Med Oncol. 2013;30:1–6.
- Balatzenko G, Vundinti BR, Margarita G. Correlation between the type of BCR-ABL transcripts and blood cell counts in chronic myeloid leukemia – a possible influence of MDR1 gene expression. Hematol Rep. 2011;3(1):e3.
- Burmeister T, Reinhardt R. A multiplex PCR for improved detection of typical and atypical BCR–ABL fusion transcripts. Leuk Res. 2008;32(4):579–585.
- Qin YZ, Jiang Q, Jiang H, et al. Prevalence and outcomes of uncommon BCR-ABL 1 fusion transcripts in patients with chronic myeloid leukaemia: data from a single centre. Br J Haematol. 2018;182(5):693–700.
- Stella S, Massimino M, Tirro E, et al. Detection and clinical implications of a novel BCR–ABL1 e12a2 insertion/deletion in a cml patient expressing the e13a2 isoform. Anticancer Res. 2019;39(12):6965–6971.
- Osman AEG, Deininger MW. Chronic myeloid leukemia: modern therapies, current challenges and future directions. Blood Rev. 2021;49:100825.
- D’Adda M, Farina M, Schieppati F, et al. The e13a2 BCR-ABL transcript negatively affects sustained deep molecular response and the achievement of treatment-free remission in patients with chronic myeloid leukemia who receive tyrosine kinase inhibitors. Cancer. 2019;125(10):1674–1682.
- Jain P, Kantarjian H, Patel KP, et al. Impact of BCR-ABL transcript type on outcome in patients with chronic-phase CML treated with tyrosine kinase inhibitors. Blood, J Am Soc Hematol. 2016;127(10):1269–1275.
- Farhat-Maghribi S, Habbal W, Monem F. Frequency of BCR-ABL transcript types in Syrian CML patients. J Oncol. 2016;2016:8420853.