ABSTRACT
This retrospective report presents the outcomes and adverse events (AEs) observed in 73 patients aged 60 years or older diagnosed with Philadelphia Chromosome-negative Acute Lymphoblastic Leukemia (Ph-negative ALL) treated with a pediatric-inspired protocol incorporating either Pegylated (PEG-ASP) or Native Asparaginase (EC-ASP). Notably, 61% of patients experienced AEs of Grade III-IV severity. The most prevalent AEs included thrombosis (35.6%), febrile neutropenia (38.4%), and transaminitis (34.2%). AEs did not translate into significant differences concerning overall survival, leukemia-free survival, or early mortality. Furthermore, we observed a reduction in early mortality rates (11% vs. 20%) and an increase in median overall survival (54 vs. 48 months) compared to our previous data. These findings suggest that the utilization of a pediatric-inspired chemotherapy protocol, with ASP, is an effective and well-tolerated therapeutic option for older patients with Ph-negative ALL. However, it emphasizes the importance of diligent monitoring and close follow-up throughout treatment.
Background
The annual incidence of Acute Lymphoblastic leukemia (ALL) in North America is estimated to be between 1.1 and 2.1 per 100,000 person-years, with higher incidences seen in children and older adults. Specifically, nearly 20% of patients with ALL are older than 60 years. Due to the aging population, taken together with improvements in the treatment of prior malignancies, and an increasing life expectancy overall, the proportion of the world population over the age of 55 is projected to reach 20% by 2030. This age increase is expected to be coupled with a greater number of age-related cases of ALL patients in the future [Citation1–3].
Reported outcomes in the elderly ALL population are inferior to those seen in children and young adults due to a combination of factors such as the adverse biology of the disease and the poor tolerance to chemotherapy [Citation4–6]. From a cytogenetic point of view, older patients have higher rates of adverse karyotypes with the BCR::ABL mutation of p190 or p210 present in approximately 50% of patients older than 50 years. Additionally, the incidence of adverse mutations, such as those of TP53 and IKZF1, is also higher in older patients [Citation7–9]. Furthermore, compared to children and young adults, older patients are more likely to have additional comorbidities and poorer performance status with decreased tolerance to intensive chemotherapy, resulting in dose reductions, treatment delays, higher treatment-related mortality, and relapse rates [Citation5, Citation10].
Risk-stratified, multi-agent and multiphase chemotherapy, given along with central nervous system prophylaxis, has significantly improved outcomes for children and young adults with ALL, with reported complete remission (CR) frequencies exceeding 90% and long-term event-free survival rates exceeding 80% [Citation11–14]. Intensive use of asparaginase (ASP) containing regimens has been a key factor contributing to the high survival rates in pediatric and young adults [Citation15–17]. However, the results remain inferior for older patients, with reported lower CR rates (40-81%), shorter long-term survival rates (3-29% at 2 years), and higher rates of mortality and relapse (7-35% and 10-45%, respectively) [Citation18–27].
Studies comparing adult ALL outcomes, using different regimens have shown dissimilar results [Citation4, Citation18–29]. ASP-based therapy has shown acceptable outcomes but also reports of notable adverse events (AEs) and higher early mortality rates (20-29%). The role and importance of ASP, including the type of ASP used, the dose, frequency, and duration, are still topics of discussion. AEs described as hepatotoxicity, thrombosis, pancreatitis, and myelosuppression have been attributed to ASP, particularly in association with increased age, obesity, and myelosuppressive agents [Citation30–32].
The advent of antibody–drug conjugates and bispecific monoclonal antibody–based options have created an entirely new avenue of treatment that is effective and tolerable, with promising results, especially in the older adult setting [Citation33–36]. The current limitation of this approach is low access to these drugs in first-line treatment outside of a clinical trial.
In our center, a pediatric-inspired chemotherapy protocol based on steroids, vinca alkaloids, anthracyclines, and ASP has been used for the past 20 years for patients 60 years of age or older with Ph-negative ALL.
In our study, the primary objective was to delineate the outcomes and AEs observed in older patients treated with ASP-inclusive protocols. This comprehensive analysis aimed to broaden the understanding of treatment implications and safety profiles in older ALL patients, particularly in the context of ASP therapies. While a comparative analysis between EC-ASP and PEG-ASP was conducted, it is important to clarify that this was a secondary aim of our research. The focus on EC-ASP versus PEG-ASP comparisons was not the central objective but rather an adjunct element to our broader investigation. The primary goal remained centered on an exploration of treatment outcomes and the identification of risk factors for AEs in the elderly ALL patient cohort.
Materials and methods
Patients
All newly diagnosed patients aged ≥ 60 with Ph-negative ALL diagnosed between January 2002 and January 2022, who received chemotherapy with a modified pediatric-inspired regimen (PM-DFCI) at our center, were included. Patients with Ph-negative B-ALL, T-ALL, or mixed-phenotype acute leukemia (MPAL) were included in this analysis. A total of 73 patients were identified for whom detailed clinical information and follow-up information were available retrospectively. Treatment was chosen and managed by the patient’s leukemia staff most responsible physician (MRP). This study was approved by the PMH institutional review board.
Treatment protocol
The treatment regimen used was a modified Dana Farber Cancer Institute Protocol (PM-DFCI, ≥ 60, Ph-ve; [a derivative of DFCI 10-175/NCIC CTG AL.4]) consisting of an induction phase, central nervous system (CNS) prophylaxis phase, seven intensification cycles, and 24 maintenance cycles, as summarized in Supplementary Table 1. In our previous work, we detailed modifications made to the DFCI protocol. These adaptations included a series of progressive changes aimed at optimizing therapeutic efficacy while minimizing toxicity in patients. Key alterations were: Induction Phase: The induction phase saw the replacement of prednisone with dexamethasone, a reduction in methotrexate dosage from 4 g/m2 to 40 mg/m2, and the elimination of one vincristine dose. These changes were driven by the goal of achieving a balance between treatment efficacy and the management of side effects. CNS Prophylaxis: In the central nervous system (CNS) prophylaxis phase, cranial radiation, traditionally a standard component, was omitted. This modification was based on emerging evidence suggesting potential long-term neurotoxicity associated with cranial irradiation. Intensification Phase: The intensification phase was adjusted from the standard ten cycles to seven cycles. This reduction was aimed at decreasing the cumulative exposure to chemotherapeutic agents, thereby potentially reducing the risk of long-term treatment-related complications. Maintenance Phase: Modifications in the maintenance phase included switching from parenteral to oral methotrexate and reducing the dose of dexamethasone. These changes were implemented to enhance patient tolerability and compliance with long-term therapy. Asparaginase Formulation Changes: Over the course of the 20-year accrual period, there was also a notable shift in the formulation of ASP used in treatment protocols [Citation20]. EC-ASP was used from 2002 until late 2017, when a pilot PEG-ASP program (PEG-ASP on a PM-DFCI backbone) started. This pilot program ended enrollment in November 2018, and previously enrolled patients continued until early 2019. Subsequently, patients again received EC-ASP, until mid-January 2020, at which time EC-ASP became unavailable. From that time onwards, all patients have received PEG-ASP. The implemented PEG-ASP dose was based on an iterative assessment of asparaginase activity level (AAL) on days 15 and 22 after each PEG-ASP dose, to achieve a serum AAL ASP level of 0.1-0.3 IU/ml [Citation25, Citation37]. Supportive therapy has also evolved. Antimicrobial prophylaxis now consists of oral fluconazole during induction and pneumocystis jirovecii (PJP) prophylaxis. Patients receive bisphosphonates, calcium, and Vitamin D for the prevention of corticosteroid-induced osteopenia. From 2011, all patients began receiving low molecular weight heparin for the prevention of thrombosis, the dosage being gradually adjusted based on the evolving clinical knowledge at our institution.
Toxicity Criteria and Response
Intention-to-treat analysis was used to analyze all endpoints. Toxicity was assessed according to the Common Terminology Criteria for Adverse Events version 4.0 (CAE4). All AEs grades 3 or greater were registered. Thrombosis was considered any deep vein thrombosis that required anticoagulation therapy according to the choice of MRP that occurred from day 1 of therapy up to 3 months after the end of the maintenance phase. Hypofibrinogenemia was considered as such when fibrinogen was less than 150 mg/dL. A multivariable analysis was performed that included all variables associated with thrombosis in the univariate analysis with a p-value <0.10 was conducted. Induction mortality was defined as death within 6 weeks of day 1 of beginning induction chemotherapy and prior to the start of the CNS phase.
Complete remission (CR1) was evaluated after day 21 if there was a hematologic recovery or at 5–6 weeks in cases where hematological recovery did not occur. CR was defined as <5% blasts in the bone marrow without evidence of extramedullary disease (EMD), transfusion independence, neutrophil >1.0 10*9/L, and platelets >100 10*9/L. Quantitative flow cytometry-based measurable residual disease (MRD) assessment was initially performed sporadically after induction until early 2014 when it transitioned to become the standard of care for all cases. Time to overall survival (OS) was calculated in months from the date of initial diagnosis to death from any cause. Patients were censored at the last date of follow-up if they were alive at the end of the study period. Leukemia-free survival (LFS) was limited to patients achieving CR1 and was defined as the time from the achievement of CR1 to relapse as defined above, or until death from any cause. Patients were censored at the last follow-up date when alive in CR1.
Measurement of Effect and Analysis of Endpoints
Categorical variables were presented as counts and percentages. Continuous variables were presented as medians with ranges. Chi-square association test or Fisher’s exact test (as appropriate) was used to assess the impact of categorical covariates of interest on ASP type (PEG-ASP vs. EC-ASP). The Kruskal–Wallis test was used to assess the impact of continuous covariates of interest on ASP type. The primary outcome of interest was OS, as defined above. The association of baseline variables with OS was assessed using log-rank test. Multivariable Cox proportional models were conducted to obtain the hazard ratio (HR). Variables with p < 0.10 in the univariable analysis and clinically important variables were included in the multivariable models to assess the joint effect and the final reduced model included significant predictors such as Asparaginase. The secondary outcome was leukemia-free survival which was analyzed similarly using time-to-event analysis and Cox proportional hazard models in obtaining hazard ratios. To assess the potential associations between AEs with variables such as age, sex, type of ASP used, accumulated dose of ASP, and BMI, a logistic regression model was performed. Statistical analyses were performed using Stata 13 (StataCorp LP, College Station, TX, USA) and SAS version 9.4 of the SAS system for Windows (Copyright © 2002–2012 SAS Institute, Inc., Cary, NC. USA).
Results
From January 2002 to January 2022, a total of 73 patients ≥ 60 years with Ph-negative ALL received treatment with the PM-DFCI >60 Ph-ve protocol. Of these patients, 47.9% were male and the median age was 67 years (range 60-86). describes the main characteristics of the patients. Most of the patients (82%, n = 60) completed the induction, CNS, intensification, and maintenance phases of therapy, while a small number discontinued treatment during or after each phase (8/73, 2/65, 3/60, and 4/57, respectively). In all cases, ASP was administered, with 52 patients receiving EC-ASP and 21 receiving PEG-ASP. The median dose of EC-ASP and PEG-ASP was 6205 IU/m2 weekly (range 5608–12825 IU/m2) and 937 IU/m2 every three weeks (range 666–1087 IU/m2), respectively, with a median number of total cycles of 7 (range 1-8) and 6 (range 1-8), respectively. The characteristics of the patients were similar in those who received EC-ASP and PEG-ASP, except WBC . Post-induction MRD was assessed in 52 patients, with a median time from diagnosis to assessment of 39 days (range 29-46).
Table 1. Patients’ characteristics.
Adverse events and their predictive factors
Grade III/IV adverse events (AEs) experienced by the patients are described in and . Febrile neutropenia (FN), grade 3–4 transaminitis, and thrombosis were the most common AEs. FN was reported in 28 patients (38.4%). Thrombosis was seen at any time during treatment in 26 patients (35.6%), of which 38% were line-related, 50% occurred during induction, and 27% during intensification. Transaminitis was observed in 25 patients (34.2%) and fully resolved in all cases. Hypofibrinogenemia, defined as <1 g/L, was found in 23 patients (31.5%), but the incidence of clinically significant bleeding between these patients was not significantly different from those without low fibrinogen. Based on biochemical or imaging criteria, pancreatitis was diagnosed in 4 patients, with all cases being considered severe, 2 cases occurred during induction and 2 during the intensification phase.
Table 2. Adverse event rates at any phase during therapy.
Relevant findings related to age, increasing age was associated with a higher FN rate, with an odds ratio (OR) of 1.11 (95% CI: 1.01-1.21, p = 0.027); Sex: thrombosis was more frequent in male patients (48.6%) compared to female patients (23.7%), OR 3.043 (95% CI 1.12–8.27, p = 0.029); type of ASP used: patients who received PEG-ASP experienced higher rates of elevated transaminases 52.4% vs 26.9%, OR 2.99 (95% CI 1.04–8.56, p = 0.041); hypofibrinogenemia 52.4% vs 23.1%, OR 3.67 (95% CI 1.26–10.71 p = 0.0175), and pancreatitis (19% vs. 1.3%, p = 0.005). There was a higher trend towards developing thrombosis in the PEG-ASP group, 52.4% vs. 28.8% for EC-ASP, OR 2.71 (95% CI 0.95–7.72, p = 0.061). The AEs of EC-ASP vs. PEG-ASP are summarized in .
Thrombosis and associated characteristics
As mentioned above, male sex was associated with a higher rate of thrombosis while PEG-ASP showed a trend. We found that the accumulated dose of PEG-ASP was associated with higher rates of thrombosis. Specifically, patients who received PEG-ASP with a cumulative dose ≥4500 IU/m2 had a 69.2% rate of thrombosis compared to 30.8% for those with a cumulative dose <4500 UI/m2, p = 0.0487. In a multivariable analysis, sex and PEG-ASP used at a higher dose remained as independent significant prognostic factors for thrombosis. Male patients had an OR of 3.68 (95% CI 1.23-11.04, p = 0.0199), and those who received PEG-ASP ≥ 4500 IU/m2 had an OR of 6.99 (95%CI 1.73-28.28, p = 0.006).
Induction Mortality, Response, Survival, and Relapse
The induction mortality rate was 11% (N = 8), while CR was achieved in 79.45% of cases (n = 58). Allogeneic hematopoietic stem cell transplantation (ASCT) in CR1 was carried out for 8.2% (N = 6). The median follow-up time, calculated using the reverse Kaplan-Meier method, was 6 years (95% CI: 4.1–6.75), and the median overall survival (OS) was 54 months (95% CI: > 23.7), as shown in (a). The 1-year and 4-year OS were 74% (95% CI 62.2–82.5) and 52% (95% CI 39.4–63.6), respectively. The leukemia-free survival at 4 years was 48% (95% CI 33.7–60.6), (b).
Factors associated with survival and relapse
In the univariate Cox proportional analysis, variables found to be significantly associated with worse OS were higher age, increased white blood cell count (WBC) at presentation, previous diagnosis of malignancy, and positive flow cytometric MRD status post-induction therapy, (a–d). However, the type of ASP used (), sex, phenotype, karyotype, and extramedullary disease (EMD) were not significantly associated with OS or LFS. Patients ≥70 years had significantly lower 2-year OS (42% [95% CI: 23.4–59.8]) compared to those < 70 years (73% [95% CI: 56.6–84.2]), p = 0.0134. A higher WBC count (>11 × 10e9/L) was also associated with a shorter 2-year OS (39% [95% CI: 18.6−59.7]) vs. (70% [95% CI: 55.1–80.8]), p = 0.0069. Patients with a previous diagnosis of malignancy had a lower OS at 2 years: 44% (95% CI 17.9–67.9) compared to those without 65% (95% CI 51-76.3), p = 0.0353. Finally, the MRD status was found to be significantly associated with OS: patients with post-induction negative MRD had OS at 1 year of 85% (95% CI: 64.0-93.9) compared to those with positive MRD with OS at 1 year of 57% (95% CI: 36.4-73.8), p = 0.0056. To note, patients who received PEG-ASP had an OS at 2 years: 66% (95% CI: 37.2–84.1) while patients who received EC-ASP: 59% (95% CI: 44.4–71.1), p = 0.5703. LFS at 2 years was 65% (95% CI: 33.1-84.7) and 59% (95% CI: 42.0-71.8) for patients who received PEG-ASP vs EC-ASP, p = 0.5950. In the construction of the multivariable model, we included variables that demonstrated statistical significance in the univariate analysis – specifically, age, elevated WBC count, prior diagnosis of malignancy, and positive MRD status following induction therapy. Additionally, we incorporated a variable of particular interest to our study, the specific type of ASP utilized. This selection was guided by both their demonstrated or theoretical relevance to the outcomes of interest and the need to adjust for potential confounders in assessing the associations under investigation. Older age and higher WBC count at presentation remained independent significant prognostic factors for worse OS. The hazard ratios (HR) were 2.28 (95% CI:1.17–4.47, p = 0.0159) and 2.43 (95% CI 1.20- 4.92, p = 0.0134) respectively. These results are summarized in .
Figure 4. (a) Overall survival (OS) by age group. Stratification by age, with a specific focus on patients aged 70 years or older, who had worse OS rates (p = 0.0134), indicating the impact of age on treatment outcomes. (b) Overall survival (OS) by white blood cell count (WBC). Categorizing according to white blood cell count, revealed that patients with a WBC count greater than 30 x 109/L exhibited poorer OS (p = 0.0069), underscoring the prognostic value of WBC count. (c) Overall survival by history of previous malignancy. Patients with a history of malignancy had poorer OS (p = 0.0353), which highlights the influence of pre-existing conditions on treatment efficacy. (d) Overall survival by measurable residual Disease (MRD) status post-induction. This figure evaluates overall survival rates based on MRD status after induction therapy, demonstrating that MRD-positive patients had significantly poorer OS (p = 0.0056), emphasizing the prognostic importance of MRD status.
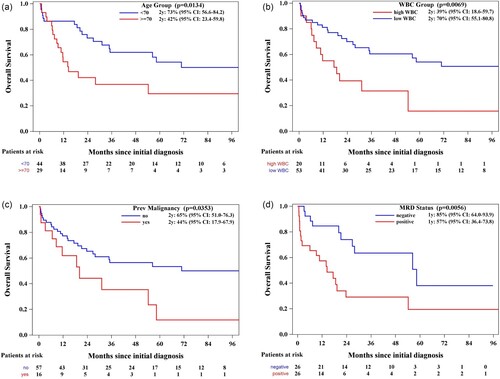
Figure 5. Overall survival and leukemia-free survival by type of asparaginase used, noting no significant differences in outcomes.
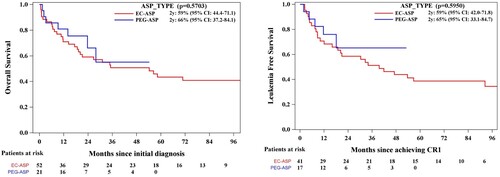
Table 3. Univariate and multivariable overall survival analysis.
Outcomes In special subsets
In the elderly ALL population worse outcomes have been attributed in part to the adverse biology of the disease. Our analysis revealed no statistically significant differences in OS at 2 years across B-ALL, T-ALL, and MPAL subtypes. Specifically, the 2-year OS rates were 60% (95% CI: 46.0–71.5) for B-ALL, 58.3% (95% CI: 18.0–84.4) for T-ALL, and 80% (95% CI: 20.4 - 96.9) for MPAL, p = 0.3827. It is noteworthy that our cohort did not include patients with early T-cell ALL, a subgroup known for poorer prognosis. Hypodiploid karyotypes were identified in 7 patients (14.9%). The 2-year OS for these patients was 42.86% (95% CI: 9.78 - 73.44), compared to 66.29% (95% CI: 52.61–76.87) for those without hypodiploid karyotypes, p = 0.0554. Although this suggests a trend, caution is warranted in interpreting these results due to the small subgroup size. Furthermore, the presence of KMT2A mutations, observed in 4 patients (5.9%), did not demonstrate a significant association with OS when compared to non-mutated patients (p = 0.5705) with again a very small subgroup size. Additionally, cytokine receptor-like factor 2 (CRLF2) overexpression was evaluated in 25 patients, with only 2 testing positive. Among these, one patient died during induction, and the other during intensification in complete remission due to sepsis. This small subset underscores the need for further investigation into the prognostic implications of CRLF2 overexpression in this population.
Rescue therapy
A total of 16 patients did not achieve CR1, among whom 9 experienced early mortality. Of the remaining 7 patients, 4 underwent reinduction therapy: three with a Hyper-CVAD-based regimen and one patient with FLAG-IDA, all 4 patients achieved remission. With regards to response to treatment of relapsed disease out of the 60 patients who achieved CR1, 24 patients (40%) relapsed, with a median time to relapse of 3 years from CR1. Among those, 16 patients received rescue therapy, resulting in the following second CR rates according to therapy: Blinatumomab 6/10, Hyper-CVAD 2/4, FLAG-IDA 1/1, and Inotuzumab Ozogamicin 1/1. Of the 10 patients who achieved a second CR, two underwent consolidation with a bone marrow transplant. Furthermore, six patients experienced a second relapse. For these patients, subsequent treatment was Blinatumomab (2) and Inotuzumab Ozogamicin (1). All three of these patients achieved a third CR and have remained disease-free with a median follow-up duration to date of 16 months (range: 6-18) after the last therapy.
Discussion
We present a single-center retrospective study that describes our experience in 73 Ph-negative ALL patients ≥ age 60 treated with a pediatric-inspired treatment protocol. Overall AEs GIII-IV were observed in 46 patients (61%). The most common AEs were thrombosis (35.6%), ≥ 3 grade febrile neutropenia (38.4%), and transaminitis (34.2%). In addition, most of the patients were able to complete the whole protocol (82%).
The switch to PEG-ASP from EC-ASP in patients with ALL treated with the DFCI protocol was supported by a phase III randomized controlled trial by Place et al. [Citation38] in which was noted similar efficacy in the 5-year survival rate and toxicities, in the pediatric population. The advantage of intravenous PEG-ASP includes reduced immunogenicity, less frequent dosing (due to a longer half-life), and flexibility in the route of administration [Citation32]. Following the pivotal results of this trial, a comprehensive modification was implemented, wherein EC-ASP was replaced by PEG-ASP for patients of all age groups. This transition led to the complete discontinuation of EC-ASP from clinical use in our setting, starting in 2020. Nevertheless, it is imperative to underscore the absence of direct comparative investigations between these two formulations and their dose-dependent implications on outcomes and adverse events, particularly in the adult population.
In our prior study, which encompassed 245 patients (with a median age of 35.4 years in the L-ASP group and 43 years in the PEG-ASP group), it was reported a thrombosis rate of 27.34% during the induction and intensification phases. Notably, significantly more thrombosis events were observed in patients treated with PEG-ASP [Citation39]. It is worth noting that, as previously mentioned, our current study included patients dating back to 2002, while the previous report focused on patients from 2011 onward. Hence, the discrepancy observed in the overall thrombosis rate may be attributed to the inclusion of thrombosis events spanning all treatment phases in the elderly cohort, along with the fact that the enrolled patients belong to different timelines with different rates of thromboprophylaxis.
Despite the implementation of low-dose prophylactic heparin as a standard of care since 2011, our study identified a higher than expected thrombosis rate, notably, with nearly one in three thrombotic events being central line related. Although this rate falls within the reported range existing in the literature (3% to 40%), we have gradually increased the dosage of low molecular weight heparin to prevent thrombosis. Our current low-dose heparin dose adjustment strategy is based on the patient’s weight and platelet count; this is detailed in Supplemental Table 2.
We identified male sex and PEG-ASP dose as significant independent factors for thrombotic events (OR 3.68 [95% CI 1.23–11.04], p = 0.0199 and 6.99 [95%CI 1.73–28.28], p = 0.006)., respectively. Therefore, we stress the significance of vigilant monitoring for this subgroup. When thrombosis occurs, our approach is to commence therapeutic anticoagulation. The choice of anticoagulation therapy is tailored according to the patient's platelet count, and asparaginase treatment is resumed subsequently [Citation31, Citation40].
The observed prevalence of elevated transaminase levels associated with PEG-ASP and EC-ASP was significantly different 52.4% and 26.9%, p = 0.041. In our series, all transaminitis cases (34.2%) resolved after temporary discontinuation of therapy. Is difficult to determine whether it can be solely attributed to ASP or is influenced by the combination with other chemotherapy drugs or steroids used in the protocol.
Compared to what is described in the literature, hypofibrinogenemia was found in almost one-third (31.5%) of patients receiving ASP [Citation30, Citation41] and was more frequent in patients who received PEG-ASP (p = 0.017). However, bleeding events did not correlate with fibrinogen levels. In our clinical practice, we correct hypofibrinogenemia (previously with cryoprecipitate and now with fibrinogen concentrate) in patients with active bleeding, in patients scheduled to undergo an invasive procedure with an intermediate or high risk of bleeding, and in patients with fibrinogen < 50 mg/dL [Citation40]. Rates of pancreatitis (5.5%) in our cohort were similar to what has been reported. Most of the cases were associated with PEG-ASP, and its use was permanently discontinued in these cases given the high risk of severe pancreatitis after rechallenge.
The efficacy and safety of EC-ASP versus PEG-ASP in adult patients have been investigated by Josep-Maria Ribera et al. in patients aged 18–60 years [Citation42]. They found no significant differences in the results or grade 3–4 toxicities during the induction period. However, a trend toward higher liver toxicity was observed in patients receiving PEG-ASP. In contrast, in our older population and throughout the entire protocol, our findings found higher rates of AEs for the PEG-ASP group compared to patients receiving EC-ASP. In summary, patients who received PEG-ASP experienced higher rates of elevated transaminases (52.4% vs. 26.9%, p = 0.041), hypofibrinogenemia (52.4% vs. 23.1%, p = 0.017), pancreatitis (19% vs. 1.3%, p = 0.05), and thrombosis (PEG-ASP: 52.4% vs. EC-ASP: 28.85%, p = 0.061).
Interestingly, and as shown in previous publications, the rates of adverse events did not translate into significant differences in outcomes between these two groups [Citation43]. In our cohort, patients treated with PEG-ASP or EC-ASP did not have significant differences in OS, LFS, or early mortality. Patients who received PEG-ASP had OS at 2 years: 66% (IC95%: 37.2–84.1) while patients who received EC-ASP: 59% (IC95%: 44.4–71.1), p = 0.8089; LFS at 2 years was 65% (IC95%: 33.1–84.7) and 59% (IC95%: 42.0–71.8) for patients who received PEG-ASP vs. EC-ASP, p = 0.5950 and, early mortality rates were 11.54% and 9.52%, respectively (p = 0.80).
The results of our study were comparable to and in some aspects better than most published relating to this population. These results are encouraging and underscore advances in disease treatment (both upfront and in salvage), supportive care, and disease understanding.
In particular, early mortality rates have decreased (11% vs. 20%), and median overall survival has increased (54 vs. 48 months) in our hands compared to our previous data [Citation20]. It is worth noting that these results were obtained through the implementation of a reduced PEG-ASP dosage strategy, which was guided by an iterative assessment of AAL to achieve a targeted serum AAL ASP level within the range of 0.1-0.3 IU/ml. These outcomes corroborate previous reports indicating that therapeutic efficacy can be maintained when adjusting the dosing regimen [Citation44, Citation45]. In the multivariable analysis, increasing age and increased white blood cell (WBC) count remained significant independent predictive factors for worse overall survival (OS) (hazard ratios [HRs]: 2.28 (95% CI:1.17–4.47, p = 0.0159) and 2.43 (95% CI 1.20- 4.92, p = 0.0134) respectively).
Despite the limited exposure to novel therapeutics like Blinatumomab and Inotuzumab Ozogamicin within our cohort, the preliminary results observed were promising. We recognize the burgeoning adoption of these novel agents in the treatment of older patients and note their progressive integration into frontline therapy by some institutions. In our practice access to these drugs in the first line is limited. This situation accentuates the necessity of advancing chemotherapy protocols, especially considering the substantial costs and variable availability of these newer agents.
We recognize that the retrospective nature of the analysis, as well as the comparison between distinct periods, presents a limitation. Furthermore, we acknowledge that the data set is confined to this particular subgroup of patients, and caution that it may not offer a complete representation of the broader population due to the potential influence of selection bias.
In conclusion, our report demonstrates that a pediatric-inspired and ASP-based chemotherapy protocol can be administered to older patients with Ph-negative ALL with encouraging tolerability and efficacy. Despite the increased occurrence of adverse events with PEG-ASP, the results were similar to those of EC-ASP. We consider ASP crucial for these outcomes and emphasize the importance of management and prevention of AEs. While we anticipate that the introduction of novel agents and new asparaginase formulations may further enhance safety and outcomes for this vulnerable population, we still find it worthwhile to pursue improvements in conventional treatment regimens. This is essential considering the high costs and uncertain accessibility of newer agents. Our commitment remains unwavering in continually refining the care of our patients to provide effective, well-tolerated, and accessible treatment options.
Contribution of the authors
MAP, AC, and HS contributed to the study conceptualization and study design. MAP, EGA, and HS contributed to data analysis, interpretation, and writing of the manuscript. GV, MD, SA, YK, BA, DM, RCG, CS, SA, MM, and HS contributed to essential data, interpretations, and manuscript revision. All authors approved the submitted and final version.
Conflict of interest disclosure
This study has not been funded by any pharmaceutical companies. There were no direct COI specifically related to this research project.
ADS has received research funding from Takeda Pharmaceuticals, BMS, and Medivir AB, and consulting fees/honorarium from Takeda, Novartis, Jazz, and Otsuka Pharmaceuticals. ADS is named on a patent application for the use of DNT cells to treat AML. ADS is a member of the Medical and Scientific Advisory Board of the Leukemia and Lymphoma Society of Canada
All other authors report no conflicts of interest.
Supplementary Table.docx
Download MS Word (15.7 KB)Acknowledgement
We thank all the patients who underwent the journey of acute lymphoblastic leukemia treatment with us at the Princess Margaret Cancer Centre, University Health Network.
Disclosure statement
No potential conflict of interest was reported by the author(s).
References
- Smith BD, Smith GL, Hurria A, et al. Future of cancer incidence in the United States: burdens upon an aging, changing nation. J Clin Oncol. 2009;27(17):2758–2765. doi:10.1200/JCO.2008.20.8983
- Sasaki K, Jabbour E, Short NJ, et al. Acute lymphoblastic leukemia: a population-based study of outcome in the United States based on the surveillance, epidemiology, and end results (SEER) database, 1980-2017. Am J Hematol. 2021;96(6):650–658. doi:10.1002/ajh.26156
- Katz AJ, Chia VM, Schoonen WM, et al. Acute lymphoblastic leukemia: an assessment of international incidence, survival, and disease burden. Cancer Causes Control. 2015;26(11):1627–1642. doi:10.1007/s10552-015-0657-6
- Gokbuget N. Treatment of older patients with acute lymphoblastic Leukaemia. Drugs Aging. 2018;35(1):11–26. doi:10.1007/s40266-017-0503-5
- Jammal N, et al. Management of acute lymphoblastic leukemia in older adults. Clin Adv Hematol Oncol. 2022;20(3):161–168.
- Ribera JM, Oriol A, Sanz M-A, et al. Comparison of the results of the treatment of adolescents and young adults with standard-risk acute lymphoblastic leukemia with the Programa Espanol de Tratamiento en Hematologia pediatric-based protocol ALL-96. J Clin Oncol. 2008;26(11):1843–1849. doi:10.1200/JCO.2007.13.7265
- Stengel A, et al. TP53 mutations occur in 15.7% of ALL and are associated with MYC-rearrangement, low hypodiploidy, and a poor prognosis. Blood. 2014;124(2):251–258. doi:10.1182/blood-2014-02-558833
- Roberts KG, Gu Z, Payne-Turner D, et al. High frequency and poor outcome of Philadelphia chromosome-like acute lymphoblastic Leukemia in adults. J Clin Oncol. 2017;35(4):394–401. doi:10.1200/JCO.2016.69.0073
- Roberts KG. The biology of Philadelphia chromosome-like ALL. Best Pract Res Clin Haematol. 2017;30(3):212–221. doi:10.1016/j.beha.2017.07.003
- Aldoss I, Stein AS. Advances in adult acute lymphoblastic leukemia therapy. Leuk Lymphoma. 2018;59(5):1033–1050. doi:10.1080/10428194.2017.1354372
- Siegel SE, Stock W, Johnson RH, et al. Pediatric-inspired treatment regimens for adolescents and young adults with philadelphia chromosome-negative acute Lymphoblastic Leukemia: a review. JAMA Oncol. 2018;4(5):725–734. doi:10.1001/jamaoncol.2017.5305
- Rytting ME, Jabbour EJ, Jorgensen JL, et al. Final results of a single institution experience with a pediatric-based regimen, the augmented Berlin-Frankfurt-Munster, in adolescents and young adults with acute lymphoblastic leukemia, and comparison to the hyper-CVAD regimen. Am J Hematol. 2016;91(8):819–823. doi:10.1002/ajh.24419
- Testi AM, Canichella M, Vitale A, et al. Adolescent and young adult acute lymphoblastic leukemia. Final results of the phase II pediatric-like GIMEMA LAL-1308 trial. Am J Hematol. 2021;96(3):292–301. doi:10.1002/ajh.26066
- Ferrari LC, Rivas MM, Navickas AB, et al. PH negative acute lymphoblastic leukemia in adolescents and young adults treated according a MRD adapted BFM ALL IC 2009 protocol: Argentine real-world data on 171 patients. Ann Hematol. 2023;102(5):1087–1097. doi:10.1007/s00277-023-05151-3
- Amylon MD, Shuster J, Pullen J, et al. Intensive high-dose asparaginase consolidation improves survival for pediatric patients with T cell acute lymphoblastic leukemia and advanced stage lymphoblastic lymphoma: a Pediatric Oncology Group study. Leukemia. 1999;13(3):335–342. doi:10.1038/sj.leu.2401310
- Raetz EA, Salzer WL. Tolerability and efficacy of L-asparaginase therapy in pediatric patients with acute lymphoblastic leukemia. J Pediatr Hematol Oncol. 2010;32(7):554–563. doi:10.1097/MPH.0b013e3181e6f003
- Sallan SE, Gelber RD, Kimball V, et al. More is better! Update of Dana-Farber Cancer Institute/Children's Hospital childhood acute lymphoblastic leukemia trials. Haematol Blood Transfus. 1990;33:459–466.
- Ribera JM, García O, Oriol A, et al. Feasibility and results of subtype-oriented protocols in older adults and fit elderly patients with acute lymphoblastic leukemia: Results of three prospective parallel trials from the PETHEMA group. Leuk Res. 2016;41:12–20. doi:10.1016/j.leukres.2015.11.012
- O'Brien S, Thomas DA, Ravandi F, et al. Results of the hyperfractionated cyclophosphamide, vincristine, doxorubicin, and dexamethasone regimen in elderly patients with acute lymphocytic leukemia. Cancer. 2008;113(8):2097–2101. doi:10.1002/cncr.23819
- Martell MP, Atenafu EG, Minden MD, et al. Treatment of elderly patients with acute lymphoblastic leukaemia using a paediatric-based protocol. Br J Haematol. 2013;163(4):458–464. doi:10.1111/bjh.12561
- Larson RA, Dodge RK, Linker CA, et al. A randomized controlled trial of filgrastim during remission induction and consolidation chemotherapy for adults with acute lymphoblastic leukemia: CALGB study 9111. Blood. 1998;92(5):1556–1564.
- Islam N, Rahman MM, Aziz MA, et al. Outcome of adult acute lymphoblastic leukaemia following induction chemotherapy with modified MRC UKALL XII/ECOG E2993 protocol. Bangladesh Med Res Counc Bull. 2012;38(2):43–46. doi:10.3329/bmrcb.v38i2.12879
- Huguet F, Leguay T, Raffoux E, et al. Pediatric-inspired therapy in adults with Philadelphia chromosome-negative acute lymphoblastic leukemia: the GRAALL-2003 study. J Clin Oncol. 2009;27(6):911–918. doi:10.1200/JCO.2008.18.6916
- El-Cheikh J, El Dika I, Massoud R, et al. Hyper-CVAD compared With BFM-like chemotherapy for the treatment of adult acute lymphoblastic leukemia. a retrospective single-center analysis. Clin Lymphoma Myeloma Leuk. 2017;17(3):179–185. doi:10.1016/j.clml.2016.11.002
- DeAngelo DJ, Stevenson KE, Dahlberg SE, et al. Long-term outcome of a pediatric-inspired regimen used for adults aged 18-50 years with newly diagnosed acute lymphoblastic leukemia. Leukemia. 2015;29(3):526–534. doi:10.1038/leu.2014.229
- Buyukasik Y, Acar K, Kelkitli E, et al. Hyper-CVAD regimen in routine management of adult acute lymphoblastic leukemia: a retrospective multicenter study. Acta Haematol. 2013;130(3):199–205. doi:10.1159/000351172
- Alacacioglu I, Medeni SS, Ozsan GH, et al. Is the BFM regimen feasible for the treatment of adult acute lymphoblastic leukemia? A retrospective analysis of the outcomes of BFM and hyper-CVAD chemotherapy in two centers. Chemotherapy. 2015;60(4):219–223. doi:10.1159/000375258
- Sive JI, Buck G, Fielding A, et al. Outcomes in older adults with acute lymphoblastic leukaemia (ALL): results from the international MRC UKALL XII/ECOG2993 trial. Br J Haematol. 2012;157(4):463–471. doi:10.1111/j.1365-2141.2012.09095.x
- Storring JM, Minden MD, Kao S, et al. Treatment of adults with BCR-ABL negative acute lymphoblastic leukaemia with a modified paediatric regimen. Br J Haematol. 2009;146(1):76–85. doi:10.1111/j.1365-2141.2009.07712.x
- Aldoss I, Douer D, Behrendt CE, et al. Toxicity profile of repeated doses of PEG-asparaginase incorporated into a pediatric-type regimen for adult acute lymphoblastic leukemia. Eur J Haematol. 2016;96(4):375–380. doi:10.1111/ejh.12600
- Bade NA, Lu C, Patzke CL, et al. Optimizing pegylated asparaginase use: An institutional guideline for dosing, monitoring, and management. J Oncol Pharm Pract. 2020;26(1):74–92. doi:10.1177/1078155219838316
- Stock W, Douer D, DeAngelo DJ, et al. Prevention and management of asparaginase/pegasparaginase-associated toxicities in adults and older adolescents: recommendations of an expert panel. Leuk Lymphoma. 2011;52(12):2237–2253. doi:10.3109/10428194.2011.596963
- Luskin MR. Acute lymphoblastic leukemia in older adults: curtain call for conventional chemotherapy? Hematology Am Soc Hematol Educ Program. 2021;2021(1):7–14. doi:10.1182/hematology.2021000226
- Topp MS, Gökbuget N, Stein AS, et al. Safety and activity of blinatumomab for adult patients with relapsed or refractory B-precursor acute lymphoblastic leukaemia: a multicentre, single-arm, phase 2 study. Lancet Oncol. 2015;16(1):57–66. doi:10.1016/S1470-2045(14)71170-2
- Kantarjian HM, Su Y, Jabbour EJ, et al. Patient-reported outcomes from a phase 3 randomized controlled trial of inotuzumab ozogamicin versus standard therapy for relapsed/refractory acute lymphoblastic leukemia. Cancer. 2018;124(10):2151–2160. doi:10.1002/cncr.31317
- Kantarjian H, Stein A, Gökbuget N, et al. Blinatumomab versus chemotherapy for advanced acute lymphoblastic leukemia. N Engl J Med. 2017;376(9):836–847. doi:10.1056/NEJMoa1609783
- Schuh A. Longitudinal therapeutic drug monitoring to optimize pegaspargase dosing in adults with acute lymphoblastic leukemia (all). EHA congress 2022. Abstract: EP407. 2020.
- Place AE, Stevenson KE, Vrooman LM, et al. Intravenous pegylated asparaginase versus intramuscular native Escherichia coli L-asparaginase in newly diagnosed childhood acute lymphoblastic leukaemia (DFCI 05-001): a randomised, open-label phase 3 trial. Lancet Oncol. 2015;16(16):1677–1690. doi:10.1016/S1470-2045(15)00363-0
- Chen R, Atenafu EG, Seki J, et al. Venous thromboembolism incidence associated with pegylated asparaginase (ASP) compared to the native L-ASP: A retrospective analysis with an ASP-based protocol in adult patients with acute lymphoblastic leukaemia. Br J Haematol. 2023;201(4):645–652. doi:10.1111/bjh.18683
- Burke PW, Hoelzer D, Park JH, et al. Managing toxicities with asparaginase-based therapies in adult ALL: summary of an ESMO Open-Cancer Horizons roundtable discussion. ESMO Open. 2020;5(5):e000858.
- Douer D, Gökbuget N, Stock W, et al. Optimizing use of L-asparaginase-based treatment of adults with acute lymphoblastic leukemia. Blood Rev. 2022;53:100908. doi:10.1016/j.blre.2021.100908
- Ribera JM, Morgades M, Montesinos P, et al. Efficacy and safety of native versus pegylated Escherichia coli asparaginase for treatment of adults with high-risk, Philadelphia chromosome-negative acute lymphoblastic leukemia. Leuk Lymphoma. 2018;59(7):1634–1643. doi:10.1080/10428194.2017.1397661
- Liang J, Shi P, Guo X, et al. A retrospective comparison of Escherichia coli and polyethylene glycol-conjugated asparaginase for the treatment of adolescents and adults with newly diagnosed acute lymphoblastic leukemia. Oncol Lett. 2018;15(1):75–82.
- Derman BA, Streck M, Wynne J, et al. Efficacy and toxicity of reduced vs. standard dose pegylated asparaginase in adults with Philadelphia chromosome-negative acute lymphoblastic leukemia. Leuk Lymphoma. 2020;61(3):614–622. doi:10.1080/10428194.2019.1680839
- Patel AA, Heng J, Dworkin E, et al. Efficacy and tolerability of a modified pediatric-inspired intensive regimen for acute lymphoblastic leukemia in older adults. EJHaem. 2021;2(3):413–420. doi:10.1002/jha2.224