ABSTRACT
Objectives:
This study aimed to establish a droplet digital polymerase chain reaction (ddPCR) assay for South-East Asian (SEA) deletion based on a fully integrated digital PCR system DropXpert S6.
Methods:
A total of 151 whole blood samples, 10 chorionic villus samples, and 17 amniotic fluid samples were collected, including 106 SEA heterozygotes, 43 normal individuals, 10 Hb Bart's hydrops details, and 19 SEA deletions combined with other genotypes.Genotypes of these samples were determined by the Gap-PCR method. We perform a series of optimizations of the ddPCR system to ensure the performance of the entire ddPCR reaction, such as droplet stability, fluorescence clustering, sensitivity, and accuracy.
Results:
Our assay exhibited 99.4% (177/178) accuracy compared with the Gap-PCR method, and the minimum detection limit of DNA was 0.1 ng/μL.Both targets have reliable linearity, R2 = 0.9999 for the α-thalassemia SEA deletion allele and R2 = 1 for the wild-type allele. The coefficient of variation for α-thalassemia SEA deletion allele detection at 2 and 10 ng/μL concentrations was 5.42% and 1.91%, respectively. In contrast, the coefficient of variation for wild-type allele detection was 4.06% and 1.83%, demonstrating its high quantitative accuracy. In addition, the DropXpert S6 PCR system showed some advantages over other ddPCR instruments, such as reducing testing costs, simplifying and automating the workflow.
Conclusions:
The DropXpert S6 PCR system provided a highly accurate diagnosis for α-thalassemia SEA deletion and can be used to detect α-thalassemia as an alternative method.
Introduction
α-Thalassemia is the most common single-gene genetic disorder, characterized by the reduction or absence of the α-globin chains due to deletion or mutation of α-globin genes.The α-globin gene cluster is located on chromosome 16 p13.3[Citation1,Citation2]. α-Thalassemia mainly involves deletions or point mutations of the HBA1 and HBA2 genes, leading to clinical significant conditions (i.e. Hb H disease or Hb Bart's hydrops fetalis) with presentations ranging from moderate moderate hemolytic anemia to severe fetal hypocia and hydrops fetalis [Citation3,Citation4]. The prevalence of α-thalassemia in certain areas of China is reported to be more than 7% [Citation5], which varies in different areas [Citation6,Citation7]. The Southeast Asian type ( – SEA) is the most common type of α-thalassemia in Southern China, accounting for approximately 4% of the population [Citation8]. There is currently no effective treatment for severe thalassemia [Citation9]. Therefore, accurate screening and diagnosis are essential for preventing and controlling thalassemia.
At present, various techniques for detecting α-thalassemia for clinical diagnosis are available. Capillary electrophoresis (CE) is a well-established technique for detecting hemoglobinopathies and can be used as a rapid screening method for cord blood Hb Bart’s [Citation10]. Gap-PCR has been widely used in clinical laboratories as a routine genetic method to diagnose α-thalassemia [Citation11]. Multiplex Ligation-Dependent Probe Amplification (MLPA) is used to detect the majority of α-thalassemia deletions [Citation12].The operation of quantitative polymerase chain reaction (qPCR) to quantify the copy number of tested genes is relatively simple, and it has become an important method of α-thalassemia detection [Citation13]. In addition, real-time SYBR Green1 PCR with high-resolution melting (HRM) analysis has been applied to detectα-thalassemia SEA deletion [Citation14]. Although these conventional techniques can diagnose α-thalassemia, they cannot be applied to non-invasive diagnosis of thalassemia. Droplet digital PCR (ddPCR) has recently become a rapidly developing technology first established by Pornprasert S et al [Citation15]. Some reports about thalassemia have demonstrated absolute quantification, reproducibility, and high accuracy, and ddPCR can be used for non-invasive prenatal diagnosis of thalassemia [Citation15,Citation16]. However, the ddPCR detection systems used in these reports were notintegrated. In this study, we aimed to establish a ddPCR assay to detect Southeast Asian type ( – SEA) deletion based on the fully integrated DropXpert S6 PCR system, allowing us to take the next step toward non-invasive detection of Hb Bart’s disease.
Materials and methods
Samples
Whole blood samples, chorionic villus, and amniotic fluid were collected from the People's Hospital of Guangxi Zhuang Autonomous Region, Nanning, Guangxi Zhuang Autonomous Region, China. These samples included 106 SEA deletion heterozygotes, 10 SEA deletion homozygotes, 43 normal individuals, 19 compound heterozygotes for the SEA deletion and other genotypes (). A total of 151 whole blood samples, 10 chorionic villus samples, and 17 amniotic fluid samples were collected. Amniotic fluid and chorionic villi samples were collected from pregnant women under B-ultrasound mediated after informed consent and signature. Patients with whole blood samples denied a history of transfusion therapy. This study was approved by the Ethics Committee of the People's Hospital of Guangxi Zhuang Autonomous Region. All patients undergoing thalassemia genetic analysis provided informed written consent. Genomic DNA was extracted using a whole blood kit (Yaneng Ltd., Shenzhen, China).
Table 1. List of primers and probes used for detection of α-thalassemia SEA deletion and wild-type fragment.
Design and operation of microfluidic chip
The microfluidic chip (A) used in this study is made of injection-molded plastic and consists of four identical microfluidic networks. Each network is capable of processing a single sample or PCR mix. The main features of the microfluidic networks are depicted in B. Each network has an inlet port that is connected to multiple microchannels. These microchannels have specific production nozzles, which ensure stable step-emulsification droplet generation. The microfluidic chip also includes a rectangular chamber (15 × 21 mm) for droplet storage and PCR reaction, as well as an outlet port connected to multiple microchannels for oil drainage.
Figure 1. The construction of microfluidic chip. The appearance of the microfluidic chip (2A). The feature of microfluidic chip with four identical networks (2B). The appearance of the pressure-permeable connecting caps (2C). The pressure-permeable connecting caps placed onto the inlet ports (2D).
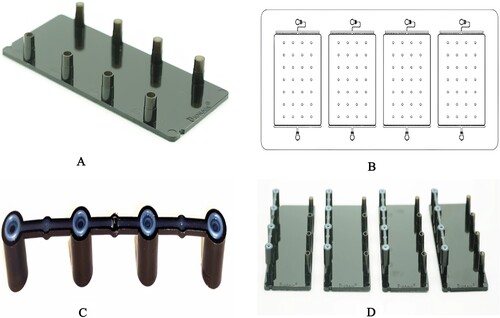
Droplets are formed in parallelized microchannels using step emulsification. The dispersed phase is injected into the microchannels, and droplet detachment occurs when there is a local loss of equilibrium due to surface tension. The microchannels, referred to as nozzles, have a triangular shape, with the cross-section gradually increasing until it reaches the storage zone, where the height abruptly increases.
The microfluidic chip is specifically designed to produce droplets with a diameter of 100 μm, corresponding to a mean droplet volume of 0.5 ± 0.03 nL. The microfluidic chips are pre-filled with an emulsion silicone oil containing droplet stabilizing surfactants. This ensures that the entire microfluidic networks and part of the ports are filled with low-density silicone oil, which has a boiling point over 200 degrees Celsius. The silicone oil acts as a cover for the water droplets, preventing water evaporation during thermocycling.
To prepare the microfluidic chips, the caps are removed from the inlet ports, and 20 μL of PCR mix is pipetted into each of the four inlet ports. Pressure-permeable connecting caps (C) are then placed onto the inlet ports (D). These caps are made of PP material and have a hole in the middle. They also feature a thin TPE circle on the top. They form a seal between the inlet port of the microfluidic chip and the syringes in the droplet generation unit within the DropXpertS6 instrument.
The DropXpert S6 System performs digital PCR using an innovative approach that integrates all the necessary steps for dPCR – compartmentalizing, thermal cycling, and data acquisition – into a single instrument. Simply pipette the reaction mixture into the sample reservoirs of C4 Chip as you would do for real-time PCR, and the platform will take care of the rest. This systemoffers a powerful yet easy-to-use digital PCR experience by using its unique microfluidic C4 chip and low-density droplet generation oil, which could cover the droplets underneath to avoid water droplets evaporation during thermocycling. The DropXpert S6 system analyzes more than 95% of the loaded sample, ensuring highly accurate digital PCR results.Two hours of automation throughout the entire process, perfectly achieving ‘chip in and result out’.
Droplet digital PCR for α-thalassemia South-East Asian-type deletion diagnosis
The PCR primers and probes were designed to amplify α-thalassemia SEA deletion and wild-type fragments (). Each reaction consisted of a 20μL solution containing 10μL of APlusTMddPCR Mix (ShenZhenBiorain Technology Co., Ltd), 500nM of wild-type primers, and 500nM of α-thalassemia SEA deletion primers, 400nM of specific probes for α-thalassemia SEA deletion allele, 600nM of specific probes for wild-type α-globin gene allele, and 5μLof template DNA. The PCR reaction mixture is pipetted into C4 chips, which are then sealed using Pressure-permeable connection caps. The chips are then placed onto the chip holder of the Droplet digital PCR system (DropXpert S6). After loading, the program will run the whole process. The samples are partitioned to form thousands of droplets and spontaneously arranged into 2D monolayer arrays of monodisperse droplets at a speed of 2μL/min. When thermocycling is completed, the chips are transferred, and images are acquired for the FAM and HEX detection channels. The cycling condition is as follows: 2 min at 95°C, then 40 cycles of 10 sec denaturation at 95°C followed by 30 sec extension at 56°C. After cycling, the instrument will automatically detect and analyze the amplification products.
Evaluation of the linearity
Qubit 4.0 (Thermo Fisher Scientific) was used to determine theα-thalassemia SEA deletion traittemplate DNA, which was then diluted to eight concentrations (64 ng/μL, 32 ng/μL, 16 ng/μL, 8 ng/μL, 4 ng/μL, 2 ng/μL, 1 ng/μL, 0.5 ng/μL). Samples of each concentration were amplified twice in duplicate.
Evaluation of the limit of blank (LoB) and the limit of detection (LoD)
The sensitivity of ddPCR depends on the number of positive droplets that can be analyzed and the false-positive rate of the mutation detection assay. Therefore, the limit of blank (LoB) and limit of detection (LoD) were calculated to avoid false positive and negative results. Multiple factors can cause false positives in digital PCR (dPCR). It is important to determine the cutoff of false positives for the stability of dPCR detection. The detection threshold is usually set higher than the LoB value to define this cutoff value. During the calibration process, the blank LoB value of each target must be determined first. We used nucleic acid-free water as a blank sample, and 20 total blank samples were replicated. The α-thalassemia SEA deletion trait template DNA was diluted to nine concentrations (5 ng/μL, 2 ng/μL,1 ng/μL, 0.5 ng/μL, 0.2 ng/μL, 0.1 ng/μL, 0.05 ng/μL, 0.02 ng/μL, and 0.01 ng/μL).In addition, the blank control was set. The templates were run twenty times at each concentration to determine the reagent's limit of detection preliminarily.
Evaluation of the repeatability
The assay was repeated 20 times at the same concentration (10 ng/μL,2 ng/μL) to study the repeatability of the reagent.
Evaluation of the accuracy
The extracted DNA samples were amplified by ddPCR, and the results were compared using the Gap-PCR (Yaneng Ltd., Shenzhen, China).
Data analysis
After allele-specific PCR reaction in the presence of fluorogenic probes, the instrument automatically interprets the amplification products in the chip. FAM-labeled and VIC-labeled probes are specifically cleaved to produce strong fluorescence for instrument recognition. Data analysis is performed by the Bio-Rain ddPCR system's software. The threshold is automatically interpreted according to the fluorescence intensities of negative and positive droplets.
Results
Quantitative results of SEA deletion by ddPCR
In the α-thalassemia SEA deletion trait, there is a wild-type allele and α-thalassemia SEA deletion allele. The two amplicons theoretically have similar copy numbers (A1-A5). Thus, the DNA copy numbers of both gene alleles were close to 1(). The copy numbers of alleles are assumed to be two. There are two α-thalassemia SEA deletion alleles in Hb Bart's hydrops fetalis andno wild-type allele (B1-B5). The ratio of the DNA copy number of both gene alleles was close to 0 (). The normal individual has two wild-type alleles and no α-thalassemia SEA deletion allele (C1-C5). The DNA copy numbers of both gene alleles were close to 2 ().
Figure 2. The difference in fluorescence amplitudes of α-thalassemia SEA deletion trait(A1-A5), Hb Bart's hydrops fetalis (B1-B5), and normal individual (C1-C5). Fluorescence of FAM and HEX channels indicated the presence of wild-type a-globin gene allele and α-thalassemia SEA deletion allele, respectively. Threshold lines are automatically interpreted by software.
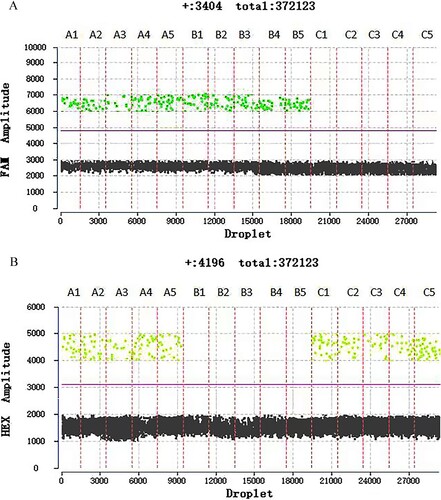
Linearity and repeatability study
The linearity of the two assay targets was investigated separately. It found that both targets had strong linearity in the template concentration range of 0.5 ng/μL to 64 ng/μL on the digital PCR platform, with R2 of 0.9999 and 1, respectively (). Twenty repeated experiments on low template DNA concentrations (2 ng/μL) and medium template DNA concentrations (10 ng/μL) were performed in the repeatability study. The coefficients of variation for the FAM channel were 5.42% and 1.91%, and for the HEX channel, they were 4.06% and 1.83% ().
Figure 4. Linear study shows a positive linear correlation between the detected and the expected concentration. R2 = 0.9999 for α-thalassemia SEA deletion allele and R2 = 1 for the wild-type allele.
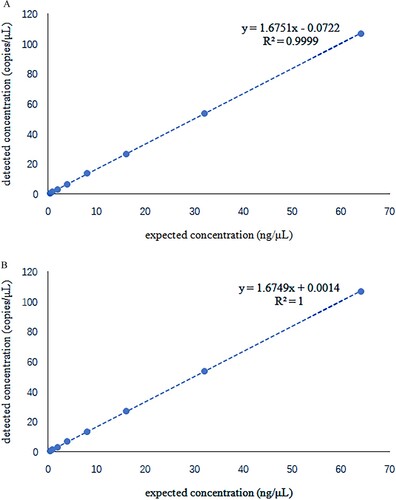
Figure 5. A total of 20 repeated experiments were performed for a repeatability study on template DNA concentration 2 ng/μL(4A) and 10 ng/μL(4B). The positive copy numbers of FAM and HEX channels were counted, respectively. Coefficients of variation were calculated based on positive copy numbers. The coefficient of variation is equal to the standard deviation divided by the mean. The coefficient of variation of the FAM channel (α-thalassemia SEA deletion allele) was 5.42%and 1.91% at 2 and 10 ng/μL template concentrations. In the HEX channel (wild-type allele) was 4.06% and 1.83% at 2 and 10 ng/μL template concentrations, respectively.
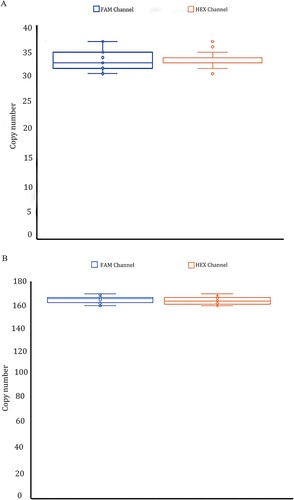
LoB, LoD, and limit detection results
The LoB study shows a total of 20 repeated tests of blank samples were not positive. According to a LoD study, the sensitivity of SEA deletion reagent on a digital PCR platform can reach 0.1 ng/L (). The limit detection of this study demonstrated that the sensitivity of SEA deletion reagent could reach 0.1 ng/μL on the digital PCR platform.
Table 2. Limit of detection study result. No positive droplet was detected in any fluorescence channel in twenty repeats of blank control. When the template DNA concentration was 0.1 ng/μL, one of the twenty repetitions was not detected, and the detection rate was 95%. The detection rate of all repetitions was 100% when the template DNA concentration was greater than or equal to 0.2 ng/μL.
Clinical accuracy verification
A total of 178 samples were tested by the DropXpert S6, of which 124 samples carried the SEA deletion, 43 were normal individuals, and 11 were homozygous for the SEA deletion. Of the 124 samples detected as carriers of SEA deletion, 106 were heterozygous for the SEA deletion and 18 were SEA deletion combined with other mutations ().One sample with compound deletion for – SEA and – α21.9 was misidentified as a SEA deletion homozygote because its copy ratio was o. In this study, the accuracy of the DropXpert S6 was 99.4% (177/178).
Table 3. Genotypes, sample types, number of cases and copy number ratios in the samples of this study. Copy number ratio intergroup tests were significantly different except for no difference between SEA heterozygotes and Compround heterozygosity for SEA deletion and other mutations (P = 0.9848).
Discussion
ddPCR is the third-generation PCR technology widely used in various fields, such as gene expression, genetic disease, tumor genes, and viruses [Citation15–17]. The ddPCR-based assay we established in this study could be used to detect α-thalassemia SEA deletion.By analyzing the copy numbers of the wild-type allele and α-thalassemia SEA deletion allele with DropXpert S6, the ratio of the wild-type allele and α-thalassemia SEA deletion allele can be easily calculated. The minimum detection limit of DNA was 0.1 ng/μL. Due to the wide linear range, whole blood, amniotic fluid, and chorionic villus samples can meet the required DNA concentration to be tested. Compared to Gap-PCR, clinical evaluation using 178 samples showed that the accuracy of DropXpert S6 was 99.4%.
Gap-PCR and MLPA methods for detecting SEA deletion are the most widely used in Chinese laboratories [Citation18,Citation19]. However, these two methods arelaborious and tedious and have more influencing factors.HRM and qPCRhave high requirements for primer probes and instruments; otherwise, the variation of TM and CT valuesis too large, which may lead to false results [Citation20]. Next-generation sequencing (NGS) can detect all known or unknown mutations in the sequencing range [Citation21]. Nevertheless, this technique is costly, time-consuming, and data must be processed using specialized bioinformatics. Digital PCR is a powerful absolute nucleic acid quantification method with unprecedented accuracy and precision [Citation22,Citation23]. Our study demonstrated that DropXpert S6 had high amplification efficiency, and results were interpreted easily without the influence of Tm and CT values variation.
In most of the existing droplet-based ddPCR systems, the droplet generation, PCR reaction, and droplet detection are performed separately in different instruments [Citation15,Citation22]. This makes the operation of digital PCR cumbersome, and the accuracy of quantification is affected by the dead volume generated during droplet transfer. Unlike the conventional digital PCR instrument, the DropXpert S6 system in this study integrates droplet generation, PCR amplification, and fluorescence detection into the instrument through the microfluidic method. It enables fluorescent detection in a single instrument reduces detectioncost, and simplifies and automates the workflow. The advantage is that there is no manual droplet transfer and amplification, reducing the error of results caused by human factors and reducing dead volume. In addition, the operator brings the work of convenience and ease to the experiment. According to our results, digital PCR has good repeatability, high sensitivity (0.1 ng/μL), and a wide linear range of DNA templates available (0.5 ng/μL∼64 ng/μL).
In this particular study, we focused on designing primers only for SEA deletion to determine whether DropXpert S6 can differentiate between SEA heterozygotes and SEA homozygotes. The results of the study showed that it was possible to distinguish SEA heterozygotes from SEA homozygotes based on copy ratios. Samples that had SEA deletion combined with other mutations, such as point mutations, triplications, or deletions, were detected as SEA heterozygotes because of the lack of mutation-associated primers. However, it was surprising to find that one sample with a compound deletion for – SEA and – α21.9 was misclassified as an SEA homozygote. This misclassification was due to the position of the primer design. Hence, it suggests that rare or novel large deletions may be present if SEA homozygotes are detected in venous blood. In prenatal diagnosis, if an SEA homozygote is detected, the genotype of the couple can easily be used to diagnose whether the fetus is Hb Bart's hydrops fetalis or Hb H disease caused by interactions of a rare large deletion with SEA deletion heterozygote.
The DropXpert S6 is capable of performing direct quantification without the need for a calibration curve, while also providing a high level of automation, droplet generation, amplification, and fluorescence signal detection. This entire process can be completed within 2 hours, making it a highly efficient and time-saving option for technical experts. However, when compared to conventional Gap-PCR, ddPCR has some limitations. For instance, it requires expensive instruments, which makes it challenging to be used commonly in primary hospitals. Moreover, additional research is necessary to determine whether DropXpert S6 can detect the 17 common β-thalassemia point mutations that reverse dot blot hybridization detects.
The droplets are formed by step emulsification in the DropXpert S6; the C4 chip allows the partitioning of the sample into thousands of nanoliter droplets, and each constitutes an individual nanoreactor. Each droplet contains either zero or one copy of the template DNA, following a Poisson distribution, enabling absolute quantification. Non-invasive prenatal diagnosis (NIPD) for thalassemia requires a highly sensitive quantitative method to distinguish fetal DNA sequences against the high background of identical maternal DNA [Citation16]. This makes it possible for us to use the DropXpert S6 PCR system for non-invasive prenatal diagnosis of thalassemia due to the absolute quantitative advantage of the instrument.
Disclosure statement
No potential conflict of interest was reported by the author(s).
Additional information
Funding
References
- Currat M, Trabuchet G, Rees D, et al. Molecular analysis of the β-globin gene cluster in the niokholo mandenka population reveals a recent origin of the βS Senegal mutation. Am J Hum Genet. 2002;70:207–223. doi:10.1086/338304
- Higgs DR, Vickers MA, Wilkie AO, et al. A review of the molecular genetics of the human alpha-globin gene cluster. Blood. 1989;73:1081–1104. doi:10.1182/blood.V73.5.1081.1081
- Liang ST, Wong VC, So WW, et al. Homozygous α-thalassaemia: clinical presentation, diagnosis and management. A review of 46 cases. Br J Obstet Gynaecol. 1985;92:680–684. doi:10.1111/j.1471-0528.1985.tb01447.x
- Lam YH, Ghosh A, Tang MH, et al. Second-trimester hydrops fetalis in pregnancies affected by homozygous alpha-thalassaemia-1. Prenat Diagn. 1997;17:267–269.
- Lai K, Huang G, Su L, et al. The prevalence of thalassemia in mainland China: evidence from epidemiological surveys. Sci Rep. 2017;7:920, doi:10.1038/s41598-017-00967-2
- Pan H, Long G, Li Q, et al. Current status of thalassemia in minority populations in Guangxi, China. Clin Genet. 2007;71:419–426.
- Bing L, Zhang X, Yin AH, et al. High prevalence of thalassemia in migrant populations in Guangdong Province, China. BMC Public Health. 2014;14:905.
- Xu XM, Zhou YQ, Luo GX, et al. The prevalence and spectrum of a and b thalassaemia in Guangdong Province: implications for the future health burden and population screening. J Clin Pathol. 2004;57:517–522.
- Barrett AN, Saminathan R. Thalassaemia screening and confirmation of carriers in parents. Best Pract Res Clin Obstet Gynaecol. 2017;39:27–40. doi:10.1016/j.bpobgyn.2016.10.015
- Li YQ, Liang L, Tian M, et al. Electrophoresis features and genotypes of Hb Bart’s hydrops fetalis. Scand J Clin Lab Invest. 2019;80:129–132.
- Xiao W, Xu X, Liu Z. Rapid detection of α-thalassemia of Southeast Asian deletion by polymerase chain reaction and its application to prenatal diagnosis. Chinese J Hematol. 2000;21:192–194.
- Kipp BR, Roellinger SE, Lundquist PA, et al. Development and clinical implementation of a combination deletion PCR and multiplex ligation-dependent probe amplification assay for detecting deletions involving the human α-globin gene cluster. J Mol Diagn. 2011;13:549–557.
- Zhou W, Wang G, Zhao X, et al. A multiplex qPCR gene dosage assay for rapid genotyping and large-scale population screening for deletional α-thalassemia. J Mol Diagn. 2013;15:642–651.
- Pornprasert S, Phusua A, Suanta S, et al. Detection of alpha-thalassemia-1 Southeast Asian type using real-time gap-PCR with SYBR Green1 and high resolution melting analysis. Eur J Haematol. 2008;80:510–514. doi:10.1111/j.1600-0609.2008.01055.x
- Pornprasert S, Prasing W. Detection of alpha (0)-thalassemia South-East Asian-type deletion by droplet digital PCR. Eur J Haematol. 2014;92:244–248. doi:10.1111/ejh.12246
- SawakwongpraK TK, Ngonsawan W, et al. Droplet-based digital PCR for non-invasive prenatal genetic diagnosis of α and β-thalassemia. Biomed Rep. 2021;15:82, doi:10.3892/br.2021.1458
- Millier MJ, Stamp LK, Hessian PA. Digital-PCR for gene expression: impact from inherent tissue RNA degradation. Sci Rep. 2017;7:17235, doi:10.1038/s41598-017-17619-0
- Oscorbin I, Kechin A, Boyarskikh U, et al. Multiplex ddPCR assay for screening copy number variations in BRCA1 gene. Breast Cancer Res Treat. 2019;178:545–555. doi:10.1007/s10549-019-05425-3
- Kojabad AA, Farzanehpour M, Galeh HEG, et al. Droplet digital PCR of viral DNA/RNA, current progress, challenges, and future perspectives. J MedVirol. 2021;93:4182–4197.
- Chong SS, Boehm CD, Higgs DR, et al. Single-tube multiplex-PCR screen for common deletional determinants of α-thalassemia. Blood. 2000;95:360–362. doi:10.1182/blood.V95.1.360
- Zhou BY, Wang YX, Xu SS, et al. Molecular spectrum of α- and β-thalassemia among young individuals of marriageable age in Guangdong Province, China. Biomed Environ Sci. 2021;34:824–829.
- Pornprasert S, Sukunthamala K, Kunyanone N, et al. Analysis of real-time PCR cycle threshold of alpha-thalassemia-1 Southeast Asian type deletion using fetal cell-free DNA in maternal plasma for noninvasive prenatal diagnosis of Bart’s hydrops fetalis. J Med Assoc Thai. 2010;93:1243–1248.
- Fan DM, Yang X, Huang LM, et al. Simultaneous detection of target CNVs and SNVs of thalassemia by multiplex PCR and next-generation sequencing. Mol Med Rep. 2019;19:2837–2848.