ABSTRACT
This study probed a psycho-motor switch between “attention” and “intention” using electroencephalography (EEG) of the occipital cortex during target versus ball focused aiming when golf putting. Specifically, we investigated (1) the pattern and power of ɑ-frequency between putting methods at 8 and 15 ft. distances and (2) the EEG activity for missed versus holed putts. Twelve highly skilled golfers executed 32 putts in total using both methods (i.e., 8 putts per condition) under naturalistic and competitive conditions. Putting performance (number of putts holed and distance from the hole) and EEG activity at −6 s, −4 s and −2 s before movement initiation were recorded. No performance difference was found for holed or missed putts between the putting methods at either distance (p > 0.05). Overall, EEG data revealed a significant time effect, with higher power at −2 s for all conditions apart from at the most challenging 15 ft. distance when using ball focused aiming, where the power decreased. For missed putts at either distance, there was twice the artefact and eye blink during ball compared to target focused aiming. Data suggest a more robust switch from attention to intention when preparing for execution with target focused aiming. Target focused aiming suggestively helps golfers to remove a potential distraction, such as from seeing the arms, hand and ball, rather than offering any distinct/different advantage to ball focused aiming. In other words, a distraction caused by what the golfer looks at rather than where gaze is directed.
Within the applied context, understanding not only “what and how” to work with an athlete, but also “why” to work in a specific way, is well-established as a hallmark of expertise amongst sport psychologists and coaches (Collins et al., Citation2015). Sometimes, however, athlete behaviour within the performance setting presents itself as entirely novel. Positively, these behaviours, although unfamiliar and difficult to explain by practitioners, can serve to enhance performance outcomes. Understandably, being aware that something might work without a justification to underpin future recommendations for other athletes, can be somewhat frustrating. In addition, a lack of mechanistic understanding could lead to suboptimal advice in pursuit of further developing the new approach.
One such example of this can be said for the dramatic change in putting style demonstrated by two Major golf champions in modern times with notable success (Jordan Spieth and Louis Oosthuizen). Ordinarily, these golfers would orientate their head and eyes over the ball during their putting action (i.e., ball focused aiming; BFA), but instead, opted for an alternative method by actively rotating their head so that they were looking in the direction of the hole or breaking point of the putt whilst putting (i.e., target focused aiming; TFA). Answering the all-important question of “why” this appeared to work for these golfers would, therefore, not only enhance our understanding from a motor control perspective, but also provide a potential extra “tool” for practitioners when working within the applied golf domain.
Notwithstanding golf research on this topic, dating back over five decades (Bowen, Citation1968), there have been relatively few studies to examine the impact of TFA on performance. Indeed, a review by Moffat et al. (Citation2017) identified only eight studies published at the time (Aksamit & Husak, Citation1983; Alpenfels et al., Citation2008; Bowen, Citation1968; Cockerill, Citation1978; Gonzalez et al., Citation2012; Gott & McGown, Citation1988; MacKenzie et al., Citation2011; Wannebo & Reeve, Citation1984). From a performance perspective, Moffat et al. reported that TFA’s effectiveness was mixed, with some studies showing an advantage over BFA, some a disadvantage and others no difference at all. Crucially, there were several important inconsistencies across these studies relating to the participant sample (i.e., inclusion of beginners, experienced and skilled golfers), equipment used (i.e., whether or not the putter was correctly tailored to the golfer based on height and style preference), dependent measures employed (i.e., putts holed vs. distance to the hole when missed), study design (i.e., single-session vs. longitudinal intervention) and experimental conditions (i.e., laboratory vs. naturalistic setting). Additionally, no studies tested TFA under conditions of competition stress and on putts of a representative nature within golf (i.e., different up and down, left and right breaking directions; see also the later study by MacKenzie & MacInnis, Citation2017). Accordingly, the state of evidence was described as inconclusive at best and incapable of providing confident recommendations about whether TFA should be employed, how, with whom, when or why. In attempting to inform future study into TFA, Moffat et al. suggested that a progressive body of research was needed to be undertaken, with methodological advancements systematically introduced to test our knowledge as it develops.
Reflecting this applied and academic need, Moffat et al. (Citation2018) tested skilled golfers (Mhandicap = 5.3) without any TFA experience on a natural putting surface with different breaking directions and under competitive conditions. Data showed no difference in mean putts holed out of 32 trials from 8 ft. between BFA (11.64 ± 2.73) and TFA (11.27 ± 2.41) putting methods. Nor was there a difference in the location pattern of missed putts. This was surprising for performers of such skill status, where a change to their normally well-practiced and established putting technique (i.e., BFA) would have expectedly resulted in a worse short-term result due to a de-automating effect (Carson & Collins, Citation2016). Consequently, Moffat et al. (Citation2018) proposed several explanations for why these results might have occurred. Firstly, they suggested that where a golfer looks once they have read the green and adopted their stance, may not be relevant to the execution process. Rather, the execution is governed by an internalised and non-visually guided motor representation. Another set of interpretations were based on the premise that any expected decrement is almost exactly countered by a process facilitated by TFA. Therefore, a second suggestion was that TFA might help to promote a focus internally towards key, or “core” (Bertollo et al., Citation2016; Bortoli et al., Citation2012; di Fronso et al., Citation2018), task-relevant movements. This state has been termed “intention” as opposed to relying on constant visual “attention” and is described as serving to positively activate the motor representation (Wertheim, Citation1981). Thirdly, TFA might assist golfers in avoiding an over-focus on task-irrelevant factors (the what they look at), possibly screening off any visual distractions from the golfer’s view that are apparent when adopting the BFA method. Fourthly, it was not discounted that a simple expectancy effect might account for this buffer. In their previous study, Moffat et al. (Citation2017) also presented the view that there could be a physio-mechanical explanation for why some previous studies had reported benefits (although these studies were not with skilled participants). In other words, the difference in posture during the movement results in an improved ability to consistently apply the correct force at impact (MacKenzie et al., Citation2011). At present, however, there is a need for several studies to advance this clearly complex conundrum.
In investigating the possible psycho-motor explanations, there are already several studies of closed and self-paced target sports (including golf putting) that can provide a methodological and mechanistic starting point for data interpretation. Specifically, these studies have utilised electroencephalography (EEG) to examine the levels of activity within the visual (occipital) cortex to understand the role of visual processing in the moments leading up to and immediately prior to movement execution. As such, these could usefully inform about how to probe the extent of visual involvement when using TFA versus BFA. Building on the suggestions of Shaw (Citation1996), Loze et al. (Citation2001) compared the power of occipital ɑ-frequency at 6 s, 4 s and 2 s epochs in expert air pistol shooters for best versus worst shots. Consistent with the attention/intention model proposed earlier by Wertheim (Citation1981), data revealed that, for the successful shots, levels of power increased in the last 2 s epoch prior to pulling the trigger. Such a change in neural activity being indicative of lower visual attention or engagement (see di Fronso et al., Citation2016). Whereas, for the unsuccessful shots, the level of power reduced sequentially across the epochs, signifying the continued use of visual attention. These data were therefore interpreted as showing a facilitative and dynamic state of cognitive control. During the performance preparation, visual attention is initially focused externally towards task-relevant information; however, once the relevant environmental information (e.g., the target) has been located within the visual field and the body/arm/pistol are correctly aligned, attentional control switches to intentional control as a means of motor memory retrieval that no longer requires the online use of visual processing. Importantly, similar findings had been reported by a series of studies in target focused sports, albeit that explanations were often different (Crews & Landers, Citation1993; Hatfield et al., Citation1984). Furthermore, a recent study by Wang et al. (Citation2021) used a comparable sample of skilled golfers (Mhandicap = 3.25) to that of Moffat et al. (Citation2018) and found EEG support for the same switch from external visual to internal information-processing when putting from individually-specific “difficult” distances (see Oliver et al., Citation2021, that report the same cognitive strategy for full swing shots in mid-handicap golfers). Taken together, these studies provide a robust effect for the dynamic use of visual attention across different target-oriented actions, best versus worst executions and have been shown to be magnified for difficult putts in golf.
While we appreciate that the work of Wertheim (Citation1981) could be considered dated, it is interesting how this visual attention/intention idea is starting to become more prominent within contemporary theories of motor control. For instance, meshed control theory (Christensen et al., Citation2016), which accounts for the dynamic use of cognition by elite performers towards strategic (e.g., selecting the correct type of shot to play), situational (e.g., identifying contextual information such as shot value/importance) and action (e.g., executing a movement in a particular way) components of a skill when faced with increased task complexity and performance difficulty. Dynamic use of cognitive processes are also reported within applied research when analysing successful performers (e.g., Bahmani et al., Citation2019). This distinctly cognitive characteristic remains, therefore, of both interest and relevance within the field of motor skill development despite the increasing prevalence of studies from other ecological positions (e.g., Davids et al., Citation2013). What has not been examined in the context of TFA, is whether the lack of performance difference upon first use by skilled BFA golfers can be understood through this dynamic switch from visual attention to motor intention. Moreover, it is important to extend the methodology of Wang et al. (Citation2021) by collecting EEG data within a naturalistic environment and with a competitive task (Moffat et al., Citation2017).
Therefore, the purposes of this study were to investigate (1) whether the pattern of power in EEG occipital ɑ-frequency for TFA was the same as BFA and if any amplification effect occurred based on task complexity (i.e., putting distance). In addition, considering the suggestion by Moffat et al. (Citation2018) that TFA might serve to prevent a negative process instead of offering a novel advantage, (2) to examine this pattern of EEG activity for missed versus holed putts. Answering this question would, in turn, provide insight into the visual engagement with environmental information by highly skilled performers and the nature/degree of cognitive activity during a representative task.
Method
Participants
Twelve highly skilled male amateur golfers, all right-handed (Mage = 36.09 years, SD = 18.56, Mhandicap = 3.72, SD = 1.60, Mexperience = 22.00 years, SD = 13.45), were recruited for this study via purposive sampling. One participant was removed (adjusted N = 11) due to poor EEG data as a result of head size being inappropriate for the equipment. Inclusion criteria required participants to be (1) an amateur golfer with a current single figure handicap of 5 or better, (2) have normal or effectively corrected vision and (3) have no previous experience using TFA as determined by self-report. Ethical approval was obtained from the University of Central Lancashire’s BAHSS Ethics Committee (no. 385) prior to conducting the study; all participants provided written informed consent prior to their participation.
Procedure
In order to promote greater ecological validity, this study took place under real-world conditions. Two holes on the outdoor practice green were selected at a Cheshire golf club, based on the breaks and slopes which reflected similar on-course conditions. Data collection took place over 2 days; on both days, green speed was typical of championship conditions, registering 9.5 on the Stimpmeter.Footnote1 Surrounding these two holes, eight shots were set up, one at 8ft. and the other at 15ft. from each hole (), identified by a tee sitting just above the grass surface. These eight positions were spaced equidistantly apart, providing a variety of challenging putts (including breaks from right-to-left and left-to-right, uphill and downhill). These determined the points from which participants should place their ball in order to complete a self-paced pre-putt routine and complete the shot.
The putting distances of 8ft. and 15ft. and location of each putt were carefully selected (Karlsen et al., Citation2008). According to Pelz (Citation1999), during competitive play, both represent meaningful distances for a 1-putt being converted approximately 70% of the time at 8ft. and 33% of the time for 15ft. by leading US tour professional golfers (PGATour, Citation2021). Based on these success rates we determined that each distance would provide a different level of challenge. Participants used their own putters and all putts were performed with new unmarked and legally conforming golf balls provided (Titleist Pro V1).
Further contributing towards the study’s ecological validity, the task was designed to recreate conditions of competition pressure by using financial incentives (Baumeister & Showers, Citation1986). Participants were informed about an individual evaluation based on the number of successful putts holed and a cash prize of £50 to be awarded to the highest scoring participant. A competitive leader board was promulgated to all participants over the 2 days of trials (Baumeister, Citation1984; Beilock & Carr, Citation2001). All participants reported that they were highly motivated to perform at their best, primarily because of their competitive nature but also because they wanted to win the cash prize.
Following the fitting of the EEG cap (see equipment details below), participants were allowed time to familiarise themselves with the equipment and pace of the greens, using non-trial holes on the same putting surface. Participants were then briefed on the process and asked to complete their normal pre-putt routine for all putts, but to utilise TFA for the specified trials. Participants were shown how to adopt the TFA technique by one of the co-researchers, who is accredited as a Specialist Coach by The Professional Golfers’ Association of Great Britain and Ireland. The TFA condition required them to fix their gaze on the target (either entry point of the hole for straight putts or the breaking point for sloped putts) for a minimum period of 2 s prior to stroke initiation and to leave the eyes fixed on this position throughout the putting stroke (cf. Binsch et al., Citation2009). The putting order for each block of eight putts was quasi-balanced across participants, alternating between starting with the BFA or TFA technique and from 8 to 15 ft. Once the trials had begun, the participant was not disturbed by the research team and was therefore allowed to putt as they would in a real competition, without any time limit or starting time imposed. Putting trials in the TFA condition were visually checked for correct technique, with no lack of adherence demonstrated. Inclusive of setup, familiarisation and testing procedures, the time taken for 32 putts ranged from 55 to 60 min per participant.
Performance measures
All putts were scored as holed or missed. For the missed putts, two-performance error measures were taken; radial (cm) and length (cm), measured using a purpose-built grid system (2 × 2 m divided into 10 cm2 sections). Missed putts were marked on the green and, following each block of eight putts, were classified to the nearest grid section when positioned on the green with the grid centre originating at the centre of the hole. In this way, we were able to determine the extent of errors. Putts finishing outside of the grid were marked as so.
EEG measures
EEG data were collected using electrodes housed within a stretchable lycra cap (waveguard) and ultra-mobile EEG unit (Ant Neuro B. V., The Netherlands). EEG data were recorded across two regions of interest (ROIs), the left and right occipital (O1 and O2), referenced to a ground placed between the Pz and Oz electrodes in accordance with standards of the international 10:20 System (Jasper, Citation1958). Analogue EEG data were subjected to a 0.5 Hz high-pass and 70 Hz low-pass filter, together with a notch filter at 50 Hz. EEG activity was sampled at 140 Hz, with a gain of 30,000 applied to the signal. Electrode impedance was ensured as below 5 KΩ before the start of each putting trial and EEG data were captured throughout the putting trial. A priori impedance testing ensured a sufficient signal-to-noise ratio.
To time-lock EEG data capture with the initiation of each putting stroke, time-locking was achieved against the artefact signal created by the initiation of movement. To avoid mistakes, a laptop computer keyboard was used by the first author to manually code the trial number, outcome and approximate timing (in milliseconds) of each putt onto the continuously recording EEG data file whilst also confirming that the movement was in fact the putt. This enabled easier cross-referencing of EEG data with the sequence of putts and subsequent results of putts holed or missed following data collection. In other words, this coding resulted in quicker, more directed and accurate guidance when examining the entire EEG dataset to identify the reference moment for all time epochs (see below) and remove the possibility of including EEG data unrelated to the putt (e.g., when moving between holes). Equally, this manual approach reduced the potential for disturbance of each participant when performing the putting trials due to additional equipment being employed. Only artefact-free segments were used for analysis, with detection using the methods detailed below.
At the end of the trials, selected data were subsequently reduced to a 6 s pre-putt period and divided into three 2 s epochs. Six seconds was selected because this enabled a greater chance of capturing any functional switches in focus completed in the preparation phase. This approach was advocated and exemplified within research pertaining to pre-performance routines (e.g., Cotterill et al., Citation2010; Singer et al., Citation1989) and has also been used in other target-focused studies (e.g., Loze et al., Citation2001). Epochs were extracted from −6, −4 and −2 s relative to the moment of putt initiation. Displays of digitally converted EEG data were then inspected visually by a qualified EEG technician to identify and remove from further analysis any pre-putt epochs with artefact, such as eye blinks and/or visible muscle activity. For each participant, the EEG technician examined 32 × 6 s epochs from when the participant addressed the putt and set up his putting stance in position to putt. These data and rejections were subsequently checked and confirmed through off-line application of independent component analysis-based algorithms (Hoffman & Falkenstein, Citation2008) to the same inspected epochs (Akhtar et al., Citation2012). This showed a rejection/retention accuracy of over 95% so the original decisions were accepted.
Finally, data were analysed using spectral analysis incorporating a Fast Fourier Transform (FFT) with a raised cosine window, yielding absolute power values for the EEG data ɑ-frequency range (8–13 Hz) for each of the three pre-putt epochs for the 32 putts. All procedures and processes followed previously published EEG studies, such as Loze et al. (Citation2001).
Data analysis
Differences in the number of putts holed and the mean total distance from the hole were examined using a Shapiro–Wilk test for determining normality of distribution. The number of putts holed were found to be normally distributed (p > 0.05) for all conditions and analysed using a 2 × 2 (Distance × Mode) ANOVA with repeated measures on all factors. For the mean total distance from the hole, all but the TFA from 8 ft. condition were found to be normally distributed. Therefore, a 2 × 2 (Distance × Mode) ANOVA with repeated measures on all factors was performed with the Greenhouse-Geisser correction taken as a more conservative estimate of the F-ratio and p-values. Differences in EEG ɑ-power were examined using a 2 × 2 × 2 × 3 (Distance × Mode × Site × Time) ANOVA with repeated measures on all factors. This provided an “omnibus test” controlling Type 1 error rates across the study. Subsequently, and if significant findings were apparent, a further two 2 × 2 × 3 (Mode × Site × Time) ANOVAs were conducted, one for the 8ft. putts and one for the 15ft. putts. As detailed below, a separate set of analyses were completed on missed putt data. Effect sizes are reported as ηp2 and interpreted using Cohen’s d thresholds of small (0.2; S), medium (0.5; M) and large (0.8; L) (Cohen, Citation1992).
Results
Putting outcome
Mean values for the number of putts holed were: BFA (4.00 ± 1.48) and TFA (4.09 ± 1.38) at 8 ft. and BFA (3.45 ± 2.07) and TFA (3.00 ± 2.10) at 15 ft. Results of the 2 × 2 ANOVA revealed no significant differences, F(1, 10) = 0.405, p = 0.54. Mean total distance from the hole for each condition were: BFA (45.42 ± 30.39) and TFA (47.15 ± 27.45) at 8 ft. and BFA (51.10 ± 24.36) and TFA (48.67 ± 27.11) at 15 ft. Results of the 2 × 2 ANOVA revealed no significant differences, F(1, 10) = 0.812, p = 0.389.
EEG successful putts
EEG data were analysed separately based on outcome, initially looking at putts made. To control the experiment-wise chance of a Type 1 error at 5%, initially all permutations of the data were tested using a 2 × 2 × 2 × 3 (Distance × Mode × Site × Time) ANOVA with repeated measures on all factors. This Omnibus Test revealed a number of significant effects, including some associated with Mode, due to higher values of ɑ-power for the 15 ft. putts.
Therefore, as the next follow-up stage, two 2 × 2 × 3 (Mode × Site × Time) ANOVAs were completed, one for the 8 ft. putts and one for the 15 ft. putts, the outputs of which formed the basis for discussion. These outcomes are shown in and , with the data presented pictorially in . As shown, the clearest effect was the significant Time effect, with ɑ-power increasing towards the moment of movement initiation.
Figure 2. EEG data for O1 at 8 ft. (A), O2 at 8 ft. (B), O1 at 15 ft. (C) and O2 at 15 ft. (D) in putts made.
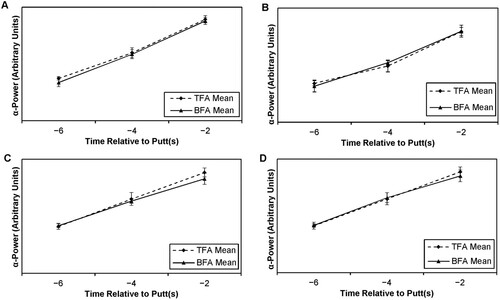
Table 1. ANOVA outcomes for 8 ft. putts made.
Table 2. ANOVA outcomes for 15 ft. putts made.
Follow-up Tukey tests, with calculation of Cohen’s d to examine effect sizes, showed the following:
Each Time epoch was significantly different to others (i.e., from −6 s to −4 s then −4 s to −2 s; all at p < 0.001, with large effect sizes). This was apparent for both 8 and 15 ft. putts.
Mode effects were due to significantly higher values in TFA than BFA, with significant differences at −4 s and −2 s (medium effect sizes for 8 ft. and small at 15 ft.).
Mode × Site effects were due to higher values at O1 than O2 that were significant at −4 s and −2 s (small effect sizes at −4 s, medium effect sizes at −2 s) with larger effect sizes at 15 ft. than 8 ft.
For 15 ft. putts, Mode, Site, Time and various interactions were due to a complex pattern of differences, all with small effect sizes, which seemed nested within the larger (and simpler to interpret!) effects listed above.
So, in summary and notwithstanding the complexities of the 15 ft. data, there was a consistent increase in ɑ-power approaching the moment of the putt, which was universal across sites O1 and O2. Furthermore, that increase seemed to be greater with TFA than BFA across both distances of 8 and 15 ft., respectively, as shown by the significant main effects of Mode. Furthermore, there is this tendency for higher ɑ-power changes eventuating in the final epoch in TFA compared to BFA.
EEG putts missed
For a variety of reasons, not least the large differences in number of data values returned for individual participants for putts missed, these putts were treated as a separate analysis. A similar sequential process was applied, starting with a 2 × 2 × 2 × 3 (Distance × Mode × Site × Time) ANOVA with repeated measures on all factors. A four-way significant interaction effect was found and these findings are presented pictorially in , with statistical results displayed in .
Figure 3. EEG data for O1 at 8 ft. (A), O2 at 8 ft. (B), O1 at 15 ft. (C) and O2 at 15 ft. (D) in putts missed.
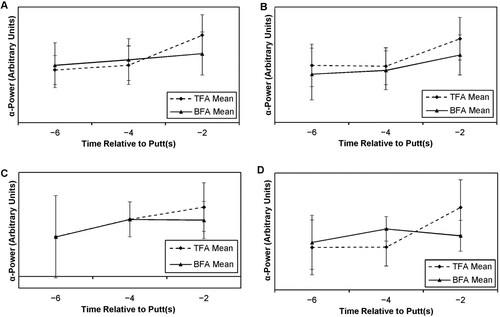
Table 3. ANOVA results for missed putts.
It is also worth noting the number of data points rejected for each putting mode due to eye blink and movement artefact. These categorical data were examined by use of Friedman’s two-way analysis by ranks, demonstrating a significant difference across the variables. Inspection shows this as due to higher rejection of BFA (means of 3.3 and 4 for each distance) as opposed to TFA (means of 1.6 and 1.3 for 8 and 15 ft., respectively) trials. In short, participants tended to have more eye movement in BFA than TFA trials.
Whilst putts missed show lower levels of significance and effect than putts made, the Time effect is still apparent. The magnitude of that difference (although significant in most cases) in ɑ-power the closer to the moment of the putt is smaller in putts missed than in putts made. Also, there is a tendency for ɑ-power to be greater in longer distances.
With putts missed, the findings from the FFT analysis are actually supplemented by the amount of muscle and eye movement artefact in the two modes. Regardless of the distance putted from, it seems that missed putts are often associated with (or possibly caused by) greater visual activity. Externally the eyes are moving or blinking, or internally, the increase in EEG ɑ-power associated with success is not occurring. The results demonstrate that, with putts missed, there is twice as much artefact and eye blink with the BFA mode than the TFA mode. Finally, post hoc analysis revealed that, for each site, both modes exhibited significantly greater FFT levels at 15 ft. than at 8 ft. (see ). The magnitude of difference between the modes was greater with putts made than with putts missed.
Once again, as the final stage of analysis, Tukey tests and Cohen’s d were calculated. These showed the following:
Final epochs were significantly different to the preceding ones (so −6 s and −4 s different to −2 s), with large effect sizes, and;
Mode × Time differences were due to different patterns across BFA and TFA. Specifically, this is seen by differences between −6 s and −4 s together with a drop in ɑ-power values for BFA against a small significant increase in values for TFA.
Notably, a four-way interaction effect seems clear in the figures and reaches significance with a small effect size. Follow-up tests showed that is due to the differential pattern in Mode apparent in the 15 ft. putt for O2 between −4 s and −2 s ((D)), which is different to (A–C).
Building from the ideas in this paper and earlier literature, the more complex picture in putts missed data are likely due to the greater number of underlying causes.
Discussion
The purposes of this study were to investigate (1) whether the pattern of power in EEG occipital ɑ-frequency for TFA was the same as BFA and if any amplification effect occurred based on task complexity (i.e., putting distance). Secondly, considering the suggestion by Moffat et al. (Citation2018) that TFA might serve to prevent a negative process instead of offering a novel advantage, (2) to examine this pattern of EEG activity for missed versus holed putts. In summary, data revealed a significant time effect, with higher ɑ-power at −2 s for all conditions apart from at the most challenging 15 ft. distance when using BFA, where the power decreased. For missed putts at either distance, there was twice the artefact and eye blink during ball compared to target focused aiming.
Before examining these EEG findings, it is interesting to note that, identically to Moffat et al. (Citation2018), utilising TFA had no immediate effect (positive or negative) on golfers that were skilled in using BFA, as measured by the number of putts holed and the total mean distance from the hole. Again, this is despite theoretical literature suggesting that a decrement in performance should occur (Carson & Collins, Citation2016; Zanone & Kelso, Citation1992). In this regard, these findings do not offer anything new to our understanding of TFA but instead, confirm that the result is consistent for putts of 8 ft. and is extended when putting from a longer distance of 15 ft. As such, for golfers of the same skill level tested in this study, from distances between 8 and 15 ft., at least, we recommend that golfers might try TFA as a “cost-free” experiment.
Moving on to examine the process measure, data showed the same pattern of EEG activity in the occipital cortex as reported for elite-level pistol shooters in the study by Loze et al. (Citation2001), when examining putts holed. In fact, this did not differ when using BFA or TFA. Interestingly, however, data did not always reflect this pattern for putts missed. Specifically, data clearly shows a marked difference in the final epoch in the BFA putts, with the most pronounced effect occurring at the 15 ft. distance. Instead of the power in EEG occipital ɑ-frequency increasing, it actually decreased which is in contrast to the TFA data that increased for either outcome and at either distance. Adopting the interpretation by Wertheim (Citation1981) and subsequently advocated by Wang et al. (Citation2021) during golf putting, these data suggest that golfers using the TFA technique were consistently able to switch from an external state of “attention” to an internal state of “intention” as the time approached closer to the movement initiation. Whereas, a stark characteristic of missed putts when using the BFA technique, was an inability to switch to a state of “intention”. Indeed, our utility of trials rejected for reasons of movement artefact would lend support towards this explanation. Of interest, other literature may overlook similar findings as being low quality in nature, but in this regard they offer a useful insight into the proficiency of visual processing. This suggests, therefore, that TFA may not provide an advantage to performance, since there was no difference between holed and missed putts, but rather could buffer against a potential negative, namely, visual distraction, that is seemingly more likely to occur when using BFA.
In light of these findings, we speculate that visual distraction when using BFA might originate from seeing the hands or putter head in the visual field. In other words, a distraction caused by what the golfer looks at rather than where gaze is directed. Of course, further study is required to clarify this speculation, ideally using both eye-tracking and EEG measures, with a larger number of missed trials included, although we appreciate that this is a significant challenge within the applied and outdoor setting. In addition, when coupled with the putt outcome data, it is now important to test what might happen when skilled golfers are provided a longer period of time to train using TFA. Based on the results presented in this study, it could be expected that performance would actually improve to a greater extent when compared with the same training regime of BFA due to an even more reliable ability to achieve an intentive state. Adopting the multi-action plan framework by Bortoli et al. (Citation2012), training with TFA may assist golfers to better clarify the technical components that are “core” to putting success and prevent a focus on stimuli that are irrelevant (e.g., grip placement) and/or over-taxing on working memory (e.g., a focus on too many technical components or in step-by-step manner) during the execution. In this way, TFA could be considered as one of the tools for coaches to promote a desirable “Type-2” (consciously controlled, optimal) performance state. Furthermore, such research may contribute to explanations underpinning quiet eye; based on our data, we would hypothesise that quiet eye was indicative of visual disengagement rather than a direct coupling between perception and action for this type of skill (Mann et al., Citation2007; Williams et al., Citation2004).
Despite the novel EEG insights provided when testing golfers using the TFA method, this study was not without limitation. Firstly, measuring the occipital sites allowed us to probe the role of vision, however, reflecting the capability of other EEG studies, our interpretation would be enhanced with a bigger montage of EEG site. For instance, in the study by Loze et al. (Citation2001), that this study generally sought to replicate, left and right anterior-temporal sites (T3 and T4) were also analysed to assess for processes governing self-talk. Given that well-established, closed and self-paced motor skills (e.g., golf putting) would expectedly rely on sensory as opposed to verbal control structures at this high skill level, associated ɑ-power activity at these additional sites could have allowed us to comment on any degree of regression in the way that movements were being controlled between the BFA and TFA styles (Fitts & Posner, Citation1967). Secondly, our analysis would have benefitted from more missed putts that were not impacted by movement artefact, especially for BFA putts. Although, this reduced number of putts for this exact reason is a meaningful finding in itself.
In conclusion, this study has extended the empirical data pertaining to the use of TFA by testing skilled golfers at a further distance and by employing EEG measures for the first time. Outcome data were able to confirm previous findings by Moffat et al. (Citation2018) that there is no immediate change in success and extend their methodology by finding the same result for error distance. Perhaps most profound of all, is that this study has shown, and is consistent for all EEG data across studies, over many years and for different sporting tasks, that you are not attending as you execute the skill. In other words, where you look when putting is immaterial.
Author contribution
The authorship order of the first three was determined alphabetically due to equal contribution within the study.
Disclosure statement
No potential conflict of interest was reported by the author(s).
Data availability statement
The data that support the findings of this study are available from the corresponding author upon reasonable request.
Notes
1 Stimp is the measure of green speed and is determined by rolling a ball with an initial speed of 6 ft. s−1 from an elevated grooved track and measuring how far it rolls on a flat portion of the putting surface.
References
- Akhtar, M. T., Mitsuhashi, W., & James, C. J. (2012). Employing spatially constrained ICA and wavelet denoising, for automatic removal of artifacts from multichannel EEG data. Signal Processing, 92(2), 401–416. https://doi.org/10.1016/j.sigpro.2011.08.005
- Aksamit, G., & Husak, W. (1983). Feedback influences on the skill of putting. Perceptual and Motor Skills, 56(1), 19–22. https://doi.org/10.2466/pms.1983.56.1.19
- Alpenfels, E., Christina, B., & Heath, C. (2008). Instinct putting. Penguin Group.
- Bahmani, M., Bahram, A., Diekfuss, J. A., & Arsham, S. (2019). An expert’s mind in action: Assessing attentional focus, workload and performance in a dynamic, naturalistic environment. Journal of Sports Sciences, 37(20), 2318–2330. https://doi.org/10.1080/02640414.2019.1631102
- Baumeister, R. F. (1984). Choking under pressure: Self consciousness and paradoxical effects of incentives on skilful performance. Journal of Personality and Social Psychology, 46(3), 610–620. https://doi.org/10.1037/0022-3514.46.3.610
- Baumeister, R. F., & Showers, C. J. (1986). A review of paradoxical performance effects: Choking under pressure in sports and mental tests. European Journal of Social Psychology, 16(4), 361–383. https://doi.org/10.1002/ejsp.2420160405
- Beilock, S. L., & Carr, T. H. (2001). On the fragility of skilled performance: What governs choking under pressure? Journal of Experimental Psychology: General, 130(4), 701–725. https://doi.org/10.1037/0096-3445.130.4.701
- Bertollo, M., di Fronso, S., Filho, E., Conforto, S., Schmid, M., Bortoli, L., Comani, S., & R, C. (2016). Proficient brain for optimal performance: The MAP model perspective. PeerJ, 4, e2082. https://doi.org/10.7717/peerj.2082
- Binsch, O., Oudejans, R. R. D., Bakker, F. C., & Savelsbergh, G. J. P. (2009). Unwanted effects in aiming actions: The relationship between gaze behavior and performance in a golf putting task. Psychology of Sport and Exercise, 10(6), 628–635. https://doi.org/10.1016/j.psychsport.2009.05.005
- Bortoli, L., Bertollo, M., Hanin, Y., & Robazza, C. (2012). Striving for excellence: A multi-action plan intervention model for shooters. Psychology of Sport and Exercise, 13(5), 693–701. https://doi.org/10.1016/j.psychsport.2012.04.006
- Bowen, R. T. (1968). Putting errors of beginning golfers using different points of aim. Research Quarterly. American Association for Health. Physical Education and Recreation, 39(1), 31–35. https://doi.org/10.1080/10671188.1968.10616526
- Carson, H. J., & Collins, D. (2016). Implementing the Five-A model of technical change: Key roles for the sport psychologist. Journal of Applied Sport Psychology, 28(4), 392–409. https://doi.org/10.1080/10413200.2016.1162224
- Christensen, W., Sutton, J., & McIlwain, D. (2016). Cognition in skilled action: Meshed control and the varieties of skill experience. Mind and Language, 31(1), 37–66. https://doi.org/10.1111/mila.12094
- Cockerill, I. M. (1978). Visual control in golf putting. In C. H. Nadeau, W. R. Halliwell, K. M. Newell, & G. C. Roberts (Eds.), Psychology of motor behaviour and sport (pp. 377–384). Human Kinetics.
- Cohen, J. (1992). A power primer. Quantitative Methods in Psychology, 112, 155–159. https://doi.org/10.1037/0033-2909.112.1.155
- Collins, D., Burke, V., Martindale, A., & Cruickshank, A. (2015). The illusion of competency versus the desirability of expertise: Seeking a common standard for support professions in sport. Sports Medicine, 45(1), 1–7. https://doi.org/10.1007/s40279-014-0251-1
- Cotterill, S. T., Sanders, R., & Collins, D. (2010). Developing effective pre-performance routines in golf: Why don't we ask the golfer? Journal of Applied Sport Psychology, 22(1), 51–64. https://doi.org/10.1080/10413200903403216
- Crews, D. J., & Landers, D. M. (1993). Electroencephalographic measures of attentional patterns prior to the golf putt. Medicine and Science in Sports and Exercise, 25(1), 116–126. https://doi.org/10.1249/00005768-199301000-00016
- Davids, K., Araújo, D., Vilar, L., Renshaw, I., & Pinder, R. (2013). An ecological dynamics approach to skill acquisition: Implications for development of talent in sport. Talent Development & Excellence, 5(1), 21–34.
- di Fronso, S., Robazza, C., Filho, E., Bortoli, L., Comani, S., & Bertollo, M. (2016). Neural markers of performance states in an Olympic athlete: An EEG case study in air-pistol shooting. Journal of Sports Science and Medicine, 15(2), 214–222.
- di Fronso, S., Tamburro, G., Robazza, C., Bortoli, L., Comani, S., & Bertollo, M. (2018). Focusing attention on muscle exertion increases EEG coherence in an endurance cycling task. Frontiers in Psychology, 9, 1249. https://doi.org/10.3389/fpsyg.2018.01249
- Fitts, P. M., & Posner, M. I. (1967). Human performance. Brooks/Cole Publishing Company.
- Gonzalez, D. A., Kegel, S., Ishikura, T., & Lee, T. (2012). Effects of vision on head-putter coordination in golf. Motor Control, 16(3), 371–385. https://doi.org/10.1123/mcj.16.3.371
- Gott, E., & McGown, C. (1988). Effects of a combination of stances and points of aim on putting accuracy. Perceptual and Motor Skills, 66(1), 1399–1143. https://doi.org/10.2466/pms.1988.66.1.139
- Hatfield, B. D., Landers, D. M., & Ray, W. J. (1984). Cognitive processes during self-paced motor performance: An electroencephalographic profile of skilled marksmen. Journal of Sport Psychology, 6(1), 42–59. https://doi.org/10.1123/jsp.6.1.42
- Hoffman, S., & Falkenstein, M. (2008). The correction of eye blink artefacts in the EEG: A comparison of two prominent methods. PLoS ONE, 3(8), e3004. https://doi.org/10.1371/journal.pone.0003004
- Jasper, H. H. (1958). The ten-twenty electrode system of the international federation. Electroencephalography and Clinical Neurophysiology, 10, 371–375.
- Karlsen, J., Smith, G., & Nilsson, J. (2008). The stroke has only a minor influence on direction consistency in golf putting among elite players. Journal of Sports Sciences, 26(3), 243–250. https://doi.org/10.1080/02640410701530902
- Loze, G. M., Collins, D., & Holmes, P. S. (2001). Pre-shot EEG alpha-power reactivity during expert air-pistol shooting: A comparison of best and worst shots. Journal of Sports Sciences, 19(9), 727–733. https://doi.org/10.1080/02640410152475856
- MacKenzie, S. J., Foley, S. M., & Adamczyk, A. P. (2011). Visually focusing on the far versus the near target during the putting stroke. Journal of Sports Sciences, 29(12), 1243–1251. https://doi.org/10.1080/02640414.2011.591418
- MacKenzie, S. J., & MacInnis, N. R. (2017). Evaluation of near versus far target visual focus strategies with breaking putts. International Journal of Golf Science, 6(1), 56–67. https://doi.org/10.1123/ijgs.2017-0009
- Mann, D. T., Williams, A. M., Ward, P. (2007). Perceptual-cognitive expertise in sport: a meta-analysis. Journal of sport and exercise psychology, 29(4), 457–478. https://doi.org/10.1123/jsep.29.4.457
- Moffat, D., Carson, H. J., & Collins, D. (2018). Golf putting: Equivalent performance with ball focused and target focused aiming. Central European Journal of Sport Sciences and Medicine, 23(3), 5–16. https://doi.org/10.18276/cej.2018.3-01
- Moffat, D., Collins, D., & Carson, H. J. (2017). Target versus ball focused aiming when golf putting: What has been done and what has been missed. International Journal of Golf Science, 6(1), 35–55. https://doi.org/10.1123/ijgs.2017-0002
- Oliver, A., McCarthy, P. J., & Burns, L. (2021). Using a “Think Aloud” protocol to understand meta-attention in club-level golfers. International Journal of Sport and Exercise Psychology, 19(5), 780–793. https://doi.org/10.1080/1612197X.2020.1766536
- Pelz, D. (1999). Dave pelz's short game Bible. Aurum Press.
- PGATour. (2021). Statistics. https://www.pgatour.com/content/pgatour/stats/stat.422.y2019.html
- Shaw, J. C. (1996). Intention as a component of the alpha-rhythm response to mental activity. International Journal of Psychophysiology, 24(1–2), 7–23. https://doi.org/10.1016/S0167-8760(96)00052-9
- Singer, R. N., Flora, L. A., & Abourezk, T. L. (1989). The effect of a five-step cognitive learning strategy on the acquisition of a complex motor task. Journal of Applied Sport Psychology, 1(2), 98–108. https://doi.org/10.1080/10413208908406408
- Wang, K.-P., Frank, C., Tsai, Y.-y., Lin, K.-H., Chen, T.-T., Cheng, M.-Y., Huang, C.-J., Hung, T.-M., & Schack, T. (2021). Superior performance in skilled golfers characterized by dynamic neuromotor processes related to attentional focus. Frontiers in Psychology, 12, 633228. https://doi.org/10.3389/fpsyg.2021.633228
- Wannebo, M., & Reeve, T. G. (1984). Effects of skill level and sensory information on golf putting. Perceptual and Motor Skills, 58(2), 611–613. https://doi.org/10.2466/pms.1984.58.2.611
- Wertheim, A. H. (1981). Occipital alpha activity as a measure of retinal involvement in oculomotor control. Psychophysiology, 18(4), 432–439. https://doi.org/10.1111/j.1469-8986.1981.tb02476.x
- Williams, A. M., Janelle, C. M., & Davids, K. (2004). Constraints on the search for visual information in sport. International Journal of Sport and Exercise Psychology, 2(3), 301–318. https://doi.org/10.1080/1612197X.2004.9671747
- Zanone, P. G., & Kelso, J. A. S. (1992). Evolution of behavioural attractors with learning: Nonequilibrium phase transitions. Journal of Experimental Psychology: Human Perception and Performance, 18(2), 403–421. https://doi.org/10.1037/0096-1523.18.2.403