ABSTRACT
Pyrolysis oil produced from municipal solid waste is being used as a fuel and little is known about the toxicity of its vapours. We investigated the effects of exposing mice to pyrolysis oil vapours on oxidative stress in lungs. This study also examined whether selenium administration exerts protective actions against the effects of pyrolysis oil vapours. Our results showed that the exposure of mice to these vapours increased the levels of malondialdehyde (MDA) and nitric oxide (NO) and decreased catalase activity in the lung as compared to that in control mice. Selenium administration before the exposure to pyrolysis oil vapours restored the levels of MDA and NO as well as catalase activity in lungs to normal. Therefore, the exposure of mice to pyrolysis oil vapours increased the oxidative stress in the lung and the administration of selenium could alleviate the effects of this exposure on oxidative stress.
1. Introduction
The continued increase in municipal solid waste (MSW) represents a serious problem which can be managed by oxygen-free pyrolysis technique for the production of pyrolysis oil that is used as an alternative source of fuel [Citation1,Citation2]. There is an association between the exposure to vapours from petroleum products and biological disorders such as oxidative stress [Citation3,Citation4]. The disturbance of the balance between the production of reactive oxygen species (ROS) and the ability of detoxifying these reactive intermediates in the body results in increased oxidative stress [Citation5]. It is characterized by the prominent generation of free radicals that leads to lipid peroxidation and consequently the destruction of lipids membranes yielding end products such as malondialdehyde (MDA) [Citation6]. Antioxidant enzymes such as catalase and superoxide dismutase (SOD) catalyse the detoxification of ROS and thus protect the body from the impacts of oxidative stress [Citation7]. Nitric oxide (NO) regulates different physiological functions such as blood pressure and neurons communication, however, NO level is increased in oxidative stress [Citation8].
Pyrolysis oil produced from MSW has the prominence of use as an alternative fuel and there are no studies examined the toxicity of its vapours in lungs of animals. This study investigated the effects of exposure of mice to pyrolysis oil vapours on oxidative stress in lungs and on plasma lipoproteins and triglycerides. We also examined whether selenium administration before the exposure to pyrolysis oil vapours exerts a protective effect against the actions of the vapours on oxidative stress.
2. Materials and methods
2.1. Animals
A number of 24 male mice, Mus musculus, were divided into four groups (six mice each), and acclimatized for 1 week prior to the initiation of exposure protocol. After this week, the body weights of mice were 25–35 g. Mice were monitored daily for health status during the study. All experimental procedures were carried out according to the National Institutes of Health (NIH) guidelines for the animal care. They were approved by the IRB committee of the Beirut Arab University (BAU), Lebanon (No.: 2016A-0021-S-P-0137 dated June 22, 2016). Mice were housed in standard cages at the animal facility at the Faculty of Science, BAU and maintained at constant temperature (20–25°C) and a 12-hour light/12-hour dark cycle. Mice were fed ad libitum with a free access to chow and water during the study.
2.2. Animal treatment
Pyrolysis oil was obtained from Green Equitech Company (Lebanon). Mice were exposed to vapours produced from pyrolysis oil for 3 hours/day for 4 consecutive days in an exposure chamber (Figure ). Animal groups that contain 6 mice each were: (i) C: control group without exposure or treatment; (ii) PO: mice were exposed to pyrolysis oil vapours; (iii) C + Se: mice were injected intraperitoneally with sodium selenite solution at a dose of 1.78 µg selenium/kg body weight. (iv) PO + Se: mice were treated with 1.78 µg selenium/kg body weight 15 minutes before the exposure to pyrolysis oil vapours.
Figure 1. Exposure chamber fabricated from glass and wood. The two compartments were separated by window wire mesh, the dimensions of each compartment are 0.5 × 0.5 × 0.5 m3.
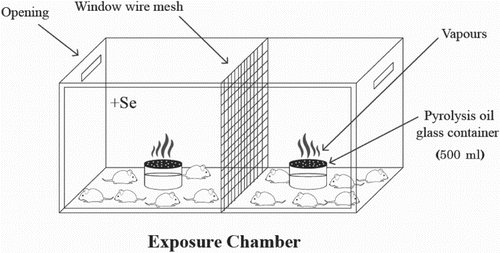
Mice from all groups were sacrificed 18 hours after the last exposure to pyrolysis oil vapours. Blood samples were collected by heart punctures into heparinized tubes and centrifuged for 20 minutes at 3000 rpm then plasma was stored at −80°C till analysis. Lungs were harvested, washed with saline, dried by blotting and stored at −80°C.
2.3. Tissue homogenization
Lungs were homogenized using a tissue homogenizer (WiseTis® Homogenizer HG-15D, Wertheim, Germany) in 0.1 M Tris–HCl buffer at pH 7.4, 0.1 M KCl and 0.5X protease inhibitor (Promega, USA). The homogenates were centrifuged at 10,000 rpm for 20 minutes at 4°C and the supernatants were stored at −80°C till analysis. Total proteins in the lungs supernatants were measured according to Bradford method [Citation9], using a standard curve of bovine serum albumin (Sigma-Aldrich, USA).
2.4. Analysis of oil samples
The elements in pyrolysis oil were analysed by graphite furnace atomic absorption spectrometer (GFAAS) 240FS with programmable sample dispenser PSD120 (Agilent technologies, USA) and the results were expressed in ppb. The sulphur content was determined by multitek (Antek-PAC, USA) applying ASTM D-5453. The Flash point (˚C) of pyrolysis oil sample was determined by flash point apparatus, model 13730-0 (Stanhope-Seta, UK) applying ASTM D-93. The density (kg/L) was determined by density meter DMA 4500 (Anton paar, Deutsh) applying ASTM D-93 [Citation10].
2.5. MDA and NO levels in lungs
The MDA and NO levels in the lungs homogenates were determined by commercial kits purchased from Biovision, USA, according to the manufacturer instructions. Both MDA and NO levels were expressed in nmol/mg protein [Citation11,Citation12].
2.6. Activities of antioxidant enzymes
Catalase and SOD activities in the lungs homogenates were determined by commercial kits (Biovision, USA) per kit instructions and their activities were expressed in mU/mg protein and U/mg protein, respectively [Citation13,Citation14].
2.7. Plasma cholesterol and triglycerides
The levels of total cholesterol (TC) and LDL-cholesterol (LDL-c) in the plasma were determined by commercial kits (Spinreact, Spain) [Citation15,Citation16]. Plasma levels of triglycerides (TG) and HDL-cholesterol (HDL-c) were determined by kits from Roche Diagnostics, Switzerland [Citation17,Citation18]. VLDL-cholesterol (VLDL-c) level was calculated by the Friedewald equation:
(VLDL-c = TG/5) [Citation19].
2.8. Statistical analysis
The analysis of data was performed using SPSS 17 software and the results were presented as mean ± SD. Data from different mice groups were analysed using one-way analysis of variance (ANOVA) followed by Tukey’s test and P < 0.05 was considered statistically significant difference.
3. Results
3.1. Analysis of pyrolysis oil
The chemical analysis of pyrolysis oil showed the presence of high content of sulphur, zinc, lead and sodium (Table ). The low value of the flash point (26°C) in pyrolysis oil indicates the presence of volatile organic components which were inhaled by mice exposed to pyrolysis oil vapours.
Table 1. Analysis of pyrolysis oil. Density (kg/L), sulphur (wt%) and flash point (°C) were measured by American Standard Test Method (ASTM) D-4052, D-5453 and D-93 methods, respectively.
3.2 Body weight
The body weights of all mice in this study were recorded daily before performing the daily exposure. The time of exposure of mice to pyrolysis oil vapours was 3 hours per day for 4 successive days and animals were sacrificed after 18 hours of their last exposure (Table ). There was no significant difference in body weights among different animal groups neither between the different days in each group.
Table 2. Daily recorded body weight (g) for control mice (C), pyrolysis oil vapours exposed group (PO), control mice treated with 1.78 µg Se/kg body weight (C + Se) and pyrolysis oil vapours exposed group treated with 1.78 µg Se/kg body weight (PO + Se).
3.3. The levels of MDA and NO in lungs
The effects of exposure of mice to the vapours of pyrolysis oil for 3 hours per day for 4 successive days on oxidative stress in lungs were assessed. MDA level in lungs of PO group was increased nonsignificantly as compared to that in the control group (P = 0.248) and was increased significantly as compared to that in the control mice treated selenium group (P = 0.046) (Figure ). Selenium administration into these mice groups, PO + Se, restored the levels of MDA in lungs to the normal range as compared to control mice (P = 0.649) or control mice treated with selenium (P = 0.993).
Figure 2. MDA levels in the lungs of control (C), pyrolysis oil vapours exposed group (PO), control treated with 1.78 µg Se/kg body weight (C + Se) and pyrolysis oil vapours exposed group treated with 1.78 µg Se/kg body weight (PO + Se). All values are presented as mean ± SD where n = 6. #Significant change at P < 0.05 when compared to control mice treated with selenium as determined by ANOVA analysis followed by Tukey’s test.
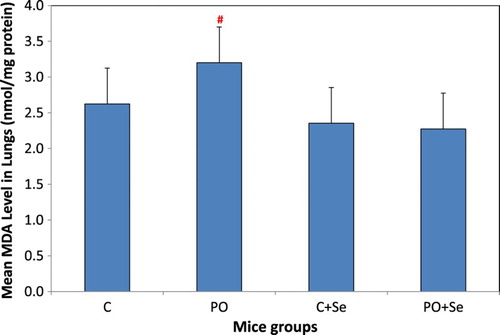
NO level in lungs of PO mice group was significantly higher than that in control mice group (P = 0.0003) and control mice treated selenium (P = 0.001) (Figure ). No significant difference was found in NO level in lungs between the selenium treated PO + Se mice group and control mice (P = 0.814) or control + Se mice group (P = 0.953).
Figure 3. NO levels in the lungs of control (C), pyrolysis oil vapours exposed group (PO), control treated with 1.78 µg Se/kg body weight (C + Se) and pyrolysis oil vapours exposed group treated with 1.78 µg Se/kg body weight (PO + Se). All values are presented as mean ± SD where n = 6. *Significant change at P < 0.05 when compared to control mice and #when compared to control mice treated with selenium as determined by ANOVA analysis followed by Tukey’s test.
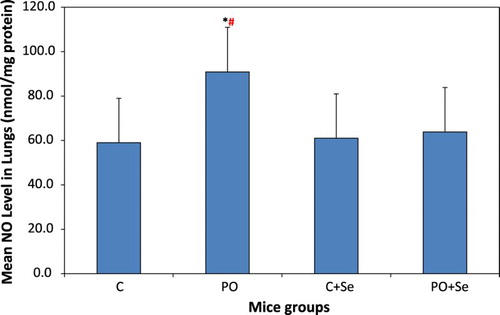
3.4. The activities of antioxidant enzymes
The activity of catalase in the lungs of mice group exposed to pyrolysis oil vapours, PO, was significantly lower than that in the control mice group (P = 0.005) and in the control + Se mice group (P = 0.007) (Figure ). Selenium treatment of PO mice group restored the activity of catalase in PO + Se mice group to normal as compared to that of the control and control + Se mice groups (P = 0.329, 0.401, respectively).
Figure 4. Catalase activity in the lungs of control (C), pyrolysis oil vapours exposed group (PO), control treated with 1.78 µg Se/kg body weight (C + Se) and pyrolysis oil vapours exposed group treated with 1.78 µg Se/kg body weight (PO + Se). All values are presented as mean ± SD where n = 6. *Significant change at P < 0.05 when compared to control mice and #when compared to control mice treated with selenium as determined by ANOVA analysis followed by Tukey’s test.
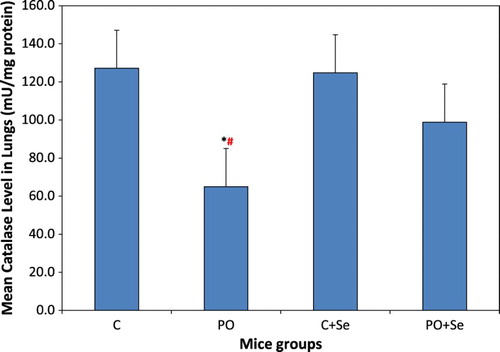
No significant change was found in the activity of SOD in lungs of PO or PO + Se groups when compared to the control mice or the control mice treated with selenium (Figure ).
3.5. Plasma cholesterol and triglycerides
TC, LDL-c, HDL-c, VLDL-c and TG levels in the plasma of all mice groups had no significant change when compared to that of control or control mice treated with selenium (Table ).
Table 3. Plasma TC, LDL-c, HDL-c, VLDL-c and TG levels (mg/dL) in control (C), pyrolysis oil vapours exposed group (PO), control treated with 1.78 µg Se/kg body weight (C + Se) and pyrolysis oil vapours exposed group treated with 1.78 µg Se/kg body weight (PO + Se).
4. Discussion
Pyrolysis oil produced from MSW contains aliphatic hydrocarbons such as aliphatic alcohols and aldehydes as well as aromatic compounds [Citation20]. Some of these volatile organic compounds are toxic and may increase oxidative stress in plasma and DNA damage in lymphocytes in humans [Citation21]. We used pyrolysis oil produced from MSW which contains some components different from that produced from biomass. For example, the MSW may include components such as batteries, plastics and pigments [Citation22,Citation23]. A previous study indicated the cytotoxicity and genotoxicity of pyrolysis oil produced from biomass in cell culture and the oxidative stress in Caenorhabditis elegans models [Citation24]. Another study suggested that pyrolysis oil produced from biomass may cause acute toxic effects in human and cancer [Citation22]. No studies have examined the effects of vapours of pyrolysis oil produced from MSW on oxidative stress in lungs of mammals. In this study, we investigated the effects of exposure of mice to pyrolysis oil vapours on oxidative stress in the lung and on the levels of plasma lipoproteins and triglycerides. We also examined whether selenium administration into these mice could alleviate the adverse effects of pyrolysis oil vapours.
Our data showed a nonsignificant increase in MDA levels and a significant increase in NO levels in lungs of mice exposed to pyrolysis oil vapours as compared to that in the control mice indicating increased oxidative stress in lungs. It has been demonstrated that MDA levels were increased in plasma of nail technicians after exposure to volatile organic compounds [Citation25]. The low value of flash point analysis of pyrolysis oil indicates the presence of volatile organic compounds such as aliphatic alcohols and aldehydes which are involved in the elevation of plasma MDA levels [Citation21,Citation25]. In addition, the high content of lead in the pyrolysis oil obtained from Green Equitech Company resulted from the components of the MSW that might include batteries, plastics and pigments [Citation23]. NO reacts with superoxide anion (O2·-) to generate reactive nitrogen species (RNS) [Citation26,Citation27]. NO levels were increased in lungs of mice and in larval tracheal tissues of Drosophila melanogaster when were exposed to volatile organic compounds [Citation28,Citation29].
The levels of MDA and NO in lungs of mice treated with pyrolysis oil vapours were restored to normal in mice pretreated with selenium. The supplementation with selenium decreases the generation of ROS and thus decreases the oxidative stress [Citation30]. The dose of selenium at 1.78 µg Se/kg body weight as sodium selenite utilized in the current study was also reported previously to alleviate the effects of exposure to waterpipe smoking on oxidative stress [Citation31].
Catalase is an antioxidant enzyme that catalyses the decomposition of hydrogen peroxide to water and oxygen to protect the cells from elevated oxidative stress [Citation32]. The elevated level of NO in the lung after exposure to pyrolysis oil vapours was associated with the decrease of the activity of catalase. It has been demonstrated that the increased generation of NO in pancreatic beta cells in vitro resulted in a decrease in catalase activity [Citation33]. Pretreatment of mice exposed to pyrolysis oil vapours with selenium restored the catalase activity in the lungs to normal. Similar results were also found in mice exposed to waterpipe smoke and pretreated with selenium where both NO levels and catalase activity were restored to normal by selenium administration [Citation31]. The supplementation of selenium ameliorates the effects of oxidative stress by reinforcing the antioxidant functions of antioxidant enzymes [Citation34].
SOD is also one of the antioxidant enzymes, it catalyses the dismutation of superoxide radicals [Citation35]. In our study, the SOD activity in the lungs of mice exposed to pyrolysis oil vapours was not affected. This data is in agreement with the results of a previous study that found a decrease in catalase activity with no change in the activity of SOD in the lungs of mice exposed to diesel exhaust emission [Citation36].
The levels of plasma lipoproteins and triglycerides in mice treated with pyrolysis oil vapours for 4 successive days showed no significant changes. It appears that the effect of exposure to pyrolysis oil vapours on the plasma lipids levels is dependent on the length of exposure.
In conclusion, the results of this study demonstrated that the short-term exposure to vapours released from pyrolysis oil induced oxidative stress in the lungs of mice and selenium pretreatment exerted protective effects against the effects of this exposure. Further investigation is required to examine the effects of long-term exposure of animals to the vapours of pyrolysis oil.
Acknowledgements
The authors would like to thank Dr Ziad Abdallah, Beirut Arab University, Department of Mathematics and Computer Science, for his support in the statistical analysis of data of this study.
Disclosure statement
No potential conflict of interest was reported by the authors.
ORCID
Youssef B. Fawaz http://orcid.org/0000-0002-8239-3957
Mohamed E. Moustafa http://orcid.org/0000-0003-1308-6221
References
- Wu D, Zhang A, Xiao L, et al. Pyrolysis characteristics of municipal solid waste in oxygen-free circumstance. Energy Procedia. 2017;105:1255–1262. doi: 10.1016/j.egypro.2017.03.442
- Ansah E, Wang L, Shahbazi A. Thermogravimetric and calorimetric characteristics during co-pyrolysis of municipal solid waste components. Waste Manag. 2016;56:196–206. doi: 10.1016/j.wasman.2016.06.015
- Njoroge R, Macharia B, Sawe D, et al. Effects of crude kerosene on testosterone levels, aggression and toxicity in rat. Toxicol Rep. 2015;2:175–183. doi: 10.1016/j.toxrep.2014.11.017
- Ma C, Lin L, Chen H, et al. Volatile organic compounds exposure and cardiovascular effects in hair salons. Occup Med. 2010;60(8):624–630. doi: 10.1093/occmed/kqq128
- Betteridge DJ. What is oxidative stress? Metab. Clin. Exp. 2000;49(2 Suppl 1):3–8. doi: 10.1016/S0026-0495(00)80077-3
- Ayala A, Munoz M, Arguelles S. Lipid peroxidation: production, metabolism, and signaling mechanisms of malondialdehyde and 4-hydroxy-2-nonenal. Oxid Med Cell Longev. 2014; 2014:1–31. DOI:10.1155.
- Shim S, Kim H. Oxidative stress and the antioxidant enzyme system in the developing brain. Korean J Pediatr. 2013;56(3):107–111. doi: 10.3345/kjp.2013.56.3.107
- Pierini D, Bryan N. Nitric oxide availability as a marker of oxidative stress. Methods Mol Biol. 2015;1208:63–71. doi: 10.1007/978-1-4939-1441-8_5
- Bradford MM. A rapid and sensitive method for the quantitation of microgram quantities of protein utilizing the principle of protein–dye binding. Anal Biochem. 1976;72:248–254. doi: 10.1016/0003-2697(76)90527-3
- Khan M, Sultana M, Al-Mamun M, et al. Pyrolytic waste plastic Oil and Its diesel blend: fuel characterization. J Environ Public Health. 2016; 2016:1–6. DOI:10.1155.
- Ohkawa H, Ohishi N, Yagi K. Assay for lipid peroxides in animal tissues by thiobarbituric acid reaction. Anal Biochem. 1979;95:351–358. doi: 10.1016/0003-2697(79)90738-3
- Cortas N, Wakid N. Determination of inorganic nitrate in serum and urine by kinetic cadmium-reduction method. Clin Chem. 1990;36:1440–1443.
- Aebi HE. Catalase in vitro. Method Enzymol. 1984;105:121–126. doi: 10.1016/S0076-6879(84)05016-3
- Nishikimi M, Roa N, Yogi K. Measurement of superoxide dismutase. Biochem Bioph Res Commun. 1972;46:849–854. doi: 10.1016/S0006-291X(72)80218-3
- Meiattini F, Prencipe L, Bardelli F, et al. The 4-hydroxybenzoate/4-aminophenazone chromogenic system used in the enzymic determination of serum cholesterol. Clin Chem. 1978;24:2161–2165.
- Okada M, Matsui H, Ito Y, et al. Low-density lipoprotein cholesterol can be chemically measured: a new superior method. J Lab Clin Med. 1998;132:195–201. doi: 10.1016/S0022-2143(98)90168-8
- Siedel J, Schmuck R, Staepels J. Long-term stable liquid ready to use mono-reagent for the enzymatic assay of serum or plasma triglycerides (GPO-PAP method). Clin Chem. 1993;39:1127–1139.
- Matsuzaki Y, Kawaguchi E, Norita Y. Evaluation of two kinds of reagents for direct determination of HDL-cholesterol. J. Anal Bio Sc. 1996;19:419–427.
- Martin S, Blaha M, Elshazly M, et al. Comparison of a novel method vs the Friedewald equation for estimating low-density lipoprotein cholesterol levels from the standard lipid profile. JAMA. 2013;310(19):2061–2069. doi: 10.1001/jama.2013.280532
- Velghe I, Carleer R, Yperman J, et al. Study of the pyrolysis of municipal solid waste for the production of valuable products. J Anal Appl Pyrolysis. 2011;92(2):366–375. doi: 10.1016/j.jaap.2011.07.011
- Hong E, Lee E, Kim Y, et al. The correlations between oxidative stress markers and indoor volatile organic compounds among the general population in Ansan and Incheon cities, Korea. Toxicol Environ Health Sci. 2009;1(1):37–48. doi: 10.1007/BF03216462
- Cordella M, Torri C, Adamiano A, et al. Bio-oils from biomass slow pyrolysis: A chemical and toxicological screening. J Hazard Mater. 2012;231-232:26–35. doi: 10.1016/j.jhazmat.2012.06.030
- Korzun E, Heck H. Sources and fates of lead and cadmium in municipal solid waste. J Air Waste Manage Assoc. 1990;40(9):1220–1226. doi: 10.1080/10473289.1990.10466766
- Chatterjee N, Eom H, Jung S, et al. Toxic potentiality of bio-oils, from biomass pyrolysis, in cultured cells and Caenorhabditis elegans. Environ Toxicol. 2014;29(12):1409–1419. doi: 10.1002/tox.21871
- Gresner P, Swiercz R, Krol M, et al. Does the low-level occupational exposure to volatile organic compounds alter the seasonal variation of selected markers of oxidative stress? A case–control study in nail technicians. J Occup Med Toxicol. 2016;11:36–46. doi: 10.1186/s12995-016-0125-6
- Elahi M, Kong Y, Matata B. Oxidative stress as a mediator of cardiovascular disease. Oxid Med Cell Longev. 2009;2(5):259–269. doi: 10.4161/oxim.2.5.9441
- Baker P, Lin Y, Schopfer F, et al. Fatty acid transduction of nitric oxide signaling: multiple nitrated unsaturated fatty acid derivatives exist in human blood and urine and serve as endogenous peroxisome proliferator-activated receptor ligands. J Biol Chem. 2005;280(51):42464–42475. doi: 10.1074/jbc.M504212200
- Wang F, Li C, Liu W, et al. Effect of exposure to volatile organic compounds (VOCs) on airway inflammatory response in mice. J Toxicol Sci. 2012;37(4):739–748. doi: 10.2131/jts.37.739
- Inamdar A, Bennett J. A common fungal volatile organic compound induces a nitric oxide mediated inflammatory response in Drosophila melanogaster. Sci Rep. 2014;4:418–426.
- Cay M, Naziroglu M, Koylu H. Selenium and vitamin E modulates cigarette smoke exposure-induced oxidative stress in blood of rats. Biol Trace Elem Res. 2009;131(1):62–70. doi: 10.1007/s12011-009-8347-4
- Charab M, Abouzeinab N, Moustafa M. The protective effect of selenium on oxidative stress induced by waterpipe (Narghile) smoke in lungs and liver of mice. Biol Trace Elem Res. 2016;174(2):392–401. doi: 10.1007/s12011-016-0737-9
- Banerjee A, Trueblood M, Zhang X, et al. N-acetylcysteineamide (NACA) prevents inflammation and oxidative stress in animals exposed to diesel engine exhaust. Toxicol Lett. 2009;187(3):187–193. doi: 10.1016/j.toxlet.2009.02.022
- Sigfrid L, Cunningham J, Beeharry N, et al. Cytokines and nitric oxide inhibit the enzyme activity of catalase but not its protein or mRNA expression in insulin-producing cells. J Mol Endocrinol. 2003;31(3):509–518. doi: 10.1677/jme.0.0310509
- Sordillo LM. Selenium-dependent regulation of oxidative stress and immunity in periparturient dairy cattle. Vet Med Int. 2013; 2013:1–8. DOI: 10.1155.
- Gongora M, Qin Z, Laude K, et al. Role of extracellular superoxide dismutase in hypertension. Hypertension. 2006;48(3):473–481. doi: 10.1161/01.HYP.0000235682.47673.ab
- Laskin D, Mainelis G, Turpin B, et al. Pulmonary effects of inhaled diesel exhaust in young and old mice: a pilot project. Res Report; 2010(151):3–31.