ABSTRACT
The present work is mainly dedicated to heterocyclic compounds as well as S-glycoside. 1,4-dihydroquinoxaline derivatives 3 were obtained from the reaction 2 with carbon disulfide in presence of potassium hydroxide. S-glycoside 4 was prepared from the reaction of compound 3 with 2,3,4,6-tetra-O-acetyl-α-D-glucopyranosyl bromide. Several heterocyclic derivatives containing thioxobenzo[g]pteridine ring systems were obtained starting from ethyl 3-amino-1,4-dihydroquinoxaline-2-carboxylate 1. These newly synthesized compounds were docked within the active site of cyclooxygenase-2 (COX-2). The results of this docking study revealed that the new compounds might exhibit good anti-inflammatory activity. The structure of new compounds was demonstrated by elemental analysis, IR, 1H NMR spectra and mass spectra.
1. Introduction
Pteridine is an important compound of heterocyclic system fused of pyrimidine ring with pyrazine ring [Citation1–3]. studies of pteridines have an important role in biological activities, such as metabolism of amino acids [Citation4–6]. Pteridine is an introduction to the synthesis of dihydrofolic acid in many microorganisms. Where pteridine and 4-Aminobenzoic acid are converted by dehydrotatease enzyme to daihydrobolic acid in the presence of glutamate [Citation7]. In addition, there are three major categories of naturally occurring patiridines: lumazines [Citation8], isoalloxazine [Citation9] and pterins [Citation10]. The lumazines, isoalloxazines and 5,10-dihydro isoalloxazines have oxosubtubents in positions 2 and 4 with the phenyl ring in position 6- and 7 in isoalloxazine (benzo[g]pteridine-2,4(3H,10H)-dione). The most common category of naturally occurring pteridines are the pterins that have the amino group in position 2 and the oxo group in position 4 [Citation11]. Studies on the development of new methods synthesized new pteridines derivatives increased here we aim to clarify all available structures and methods related to pteridines
Quinoxaline is important of the heterocyclic compounds containing nitrogen atom which playing a broad spectrum of biological and pharmacological activities like as insecticide [Citation12], fungicide [Citation13], herbicide [Citation14], anthelmintic [Citation15], antibacterial [Citation16], antimycobacterial [Citation17], antiprotozoal [Citation18], anticancer properties [Citation19] and anti-inflammatory activity [Citation20]. For example, Wagly et al [Citation21]recorded that the quinoxaline derivative (A) showed anti-inflammatory activity (63.33%) as showed by the standard drug indomethacin. Furthermore, thioether derivatives of 2-chloro-3-methylquinoxaline had been prepared and evaluated for their anti-inflammatorry activity using carrageenan induced rat paw edema method [Citation22]. The biological study of these derivatives demonstrated that derivative (B) exhibited the highest inflammatory inhibition (40.09%) at dose 100 mg/ kg. In the view of the aforesaid facts and in continuation of our work in the synthesis of biologically active heterocycles [Citation23–29]. In the view of the aforesaid facts and in continuation of our work in the synthesis of biologically active heterocycles [Citation30–36],we aimed at synthesis of novel quinoxaline and pteridine derivatives of expected anti-inflammatory activity.
2. Results and discussion
2.1. Chemistry
The ester group of ethyl 3-amino-1,4-dihydroquinoxaline-2-carboxylate 1 [Citation37] reacted with hydrazine hydrate to give the corresponding hydrazide derivative 2, which was refluxed with carbon disulfide in presence of 10% potassium hydroxide to produce the corresponding oxadiazole derivatives 3 in 76% yield. Glycosylation of 5-(2-amino-1,4-dihydroquinoxalin-3-yl)-1,3,4-oxadiazole-2-thiol 3 was carried out by the reaction of compound 3 with the acetylated glucopyranosyl bromide in the presence of potassium hydroxide afforded the corresponding glycoside 4. The 1HNMR of 4 determined the presence of the anomeric proton of the sugar moiety at 5.98 ppm as a doubled with coupling constant equals to 9.32 Hz due to the β-orientation of the thioglycoside bond Scheme 1
Furthermore, the reaction of 1 with benzoyl isothiocyanate in presence of 70% sodium hydroxide under reflux afforded 2,3-dihydro-3-phenyl-2-thioxobenzo[g]pteridin-4(1H,5H,10H)-one 5 which confirmed by analytical and spectral data. Thus IR appearance the (C = O) at 1689 and at 1292 (C = S). The 1HNMR spectrum of compound 5 showed multiplet at 7.32–7.83 ppm corresponding to aromatic protons in addition to the appearance of other two singlet signals at 11.26 and13.03 (2H) corresponding to 2NH protons of the pyrimidine ring. Also, the reaction of compound 1 with phenyl isothiocyanate in presence of triethylamine and dioxane gave 2,3-dihydro-2-thioxobenzo[g]pteridin-4(1H,5H,10H)-one 6 which its reacted with ethanolic sodium hydroxide to yield ethyl-3-(amino-N-phenylmethanethioamino)-1,4-dihydroquinoxaline-2-carboxylate (7) Scheme 2.
Benzo[g]pteridin derivatives 5 and/ or 7 reacted with 2,3,4, 6-tetra-O-α-acetyl glucopyranosyl bromide in aqueous potassium hydroxide afforded the corresponding glycosyl derivatives 8, 9 respectively. The 1HNMR of the product determined thioglycosides led to appearance four single peak due to acetyl group in addition to the other protons of the sugar chain. The formation of these thioglycosides and linkage of the sugar part at a sulfur centre was determined by the 1HNMR. The 1HNMR appearance anomeric proton (H-1) at 5.72 ppm.
In addition, methylation of compounds 5 and 7 by methyl iodide in presence of potassium hydroxide produced 2-(methylthio)benzo[g]pteridin derivatives 10 and 11 respectively, as shown in Scheme 3. 1HNMR spectra of compounds10 and 11 gave a single peak at 2.69 ppm for methyl group and the site attack found on sulfur not found on the nitrogen. Compounds 10, 11 were reacted with hydrazine hydrate in ethanol to afford 12 and 13 respectively. The structures of compounds 12 and 13 were confirmed by 1HNMR spectra which showed signals for NHNH2 at 5.16 and 10.07, respectively. Furthermore, Schiff bases 14 and 15 were prepared by heating under reflux compounds 12 and 13 with D-glucose in presence of glacial acetic acid. Acetylated of Hydrazone derivative 14 was produced by heating with acetic anhydride gave acetylated glycoside 16. The IR spectrum of the compound 16 confirmed the absence of hydroxyl and NH2 group, and apparent absorption bands at 1748 cm−1 due to (C = O).
2.2. Molecular simulation study
Non-steroidal anti-inflammatory drugs (NSAIDs) are used to alleviate and treat inflammation, fever, and pain. It was recognized that these NSAIDs produce their action mainly via inhibiting the cyclooxygenase enzymes (COXs) which biosynthesize prostaglandins (PGs), prostacyclin (PGI2) and thromboxanes (TXA2) [Citation38–40]. Nonselective COX inhibitors as aspirin and indomethacin showed severe side effects as ulcers and renal damage[Citation34–36]. These side effects directed scientists to synthesize COX-2 selective drugs like celecoxib and rofecoxib [Citation37,Citation38] but unfortunately, some of these such as (rofecoxib) was withdrawn from the market due to the reported increase in the risk of the cardiovascular disease [Citation39,Citation40]. So there is a great need for a synthesis of more selective COX-2 inhibitors with fewer side effects.
The secondary pocket present in COX-2 has been attributed to the presence of the smaller valine (Val523). Replacement of histidine (His513) in COX-1 by arginine (Arg513) in COX-2 has been reported to play a key role in the hydrogen-bond network of the COX active site. Histidine (His90), glutamine (Gln192), and tyrosine (Tyr355) control the access of ligands into the secondary pocket. The interaction of Arg513 with the bound ligand has been reported to be a requirement for the time-dependent inhibition of COX-2 [Citation41].
The target compounds 4, 8, 9 and 16 were subjected to molecular simulation study to expect their biological activity as anti-inflammatory agents.COX-2 X-ray crystal structure downloaded from the protein data bank with code (PDB: ID 1CX2). This study was performed using MOE.2010 software (Molecular Operating Environment).
In this work, the compounds 4, 8, 9 and 16 were docked within COX-2 active site to explore the activity of these novel benzo[g]pteridine and 1,4-dihydroquinoxalin derivatives as COX-2 inhibitors. The ligand used in this study was S-58 (bromocelecoxib) which was redocked into COX-2 with a score energy (S) = −11.93 kcal/mol.S-58 showed two hydrogen bonding interactions with Arg513and His90 amino acids with -SO2 group through in a distance equal to 2.41 and 2.30 Å. The docking results including the associated energy with intermolecular interactions obtained upon docking for the docked compounds(4,8, 9,16 and S-58) within COX-2 active site and hydrogen bonding interactions between the amino acid residues and functional groups of compounds are explained in Table .
Table 1. Molecular modelling data for compounds 4, 8, 9, 16 and S-58 during docking in the active site of the COX-2 enzyme (PDB: ID 1CX2).
First, compound 4 revealed energy score = −13.58 kcal/mol exhibiting three hydrogen bonding interactions: a) His90 with oxadiazole N (2.41 A○), b) Arg120 with C = O (2.21 A○) and c) Tyr355 (2.87 A○) (Figure ).
Figure 1. A) Binding of the candidate 4 with COX-2 (using MOE site finder programme), the dotted lines represent H-bonding interactions between oxadiazole N and His90 and between the acetyl C = O atom and Tyr355& Arg120. B) 3D interactions of 4 with Tyr355, Arg120, and His90 acid residues.
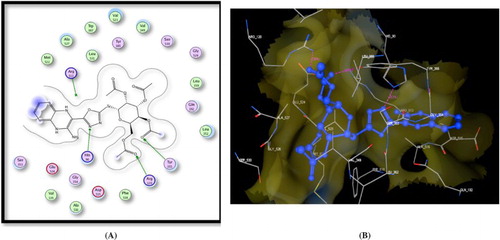
Moreover, the target compound 8 showed good fitting with COX-2 enzyme and performed three hydrogen bonding interactions. One hydrogen bond between Leu352 and pteridine NH, the other one between Gln292 and pteridine C = O and last hydrogen bond interaction between Arg513 and acetyl C = O (Figure ).
Figure 2. A) Binding of the candidate 8 with COX-2 (using MOE site finder programme), the dotted lines represent H-bonding interactions between NH and Leu352, pteridine C = O, and Gln292 and between the acetyl C = O atom and Arg513. B) 3D interactions of 8 with Leu352, Gln292, and Arg513 acid residues.
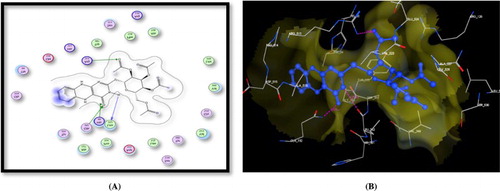
Docking of the target compound 9 recorded three hydrogen bonding interactions with energy score = −12.62kcal/mol: i) Ser530 with acetyl C = O, ii) Tyr385 with acetyl C = O and iii) Arg513 with pteridine C = O. In addition, this compound showed two arene cation interactions between the phenyl ring attached to pteridine moiety and Arg513 and Arg120 (Figure ).
Figure 3. A) Binding of the candidate 9 with COX-2 (using MOE site finder programme), the dotted lines represent H-bonding interactions between acetyl C = O and Ser530 & Tyr385 and pteridine C = O and Arg513 and the arene cation interactions between the phenyl ring and Arg513 & Arg120. B) 3D interactions of 9 with Ser530 & Tyr385 and Arg513 acid residues.
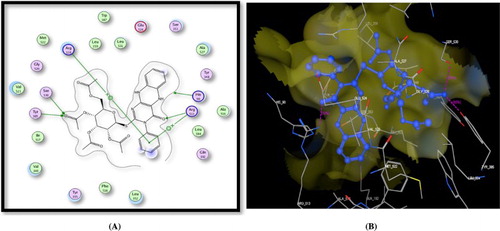
Figure 4. A) Binding of the candidate 16 with COX-2 (using MOE site finder programme), the dotted lines represent H-bonding interactions between acetyl C = O and His90 & Arg513 and Arg120 and the arene cation interactions between the benzene ring and Arg120. B) 3D interactions of 16 with His90 & Arg513 and Arg120 acid residues.
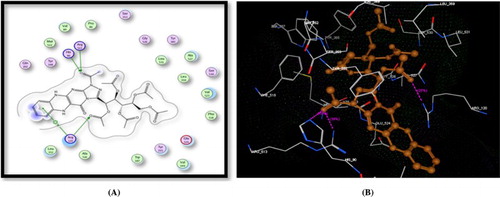
Finally, compound 16 showed hydrogen bonding interactions similar to that exhibited with S-58 (with Arg513 and His90), in addition to other hydrogen bonding interaction between Arg120 and C = O. Furthermore, arene cation interaction had been recorded between Arg120 and benzene ring (Figure ).
3. Experimental sEcTiOn
3.1. Chemistry
3.1.1. General Procedures
Melting points were determined on Electro thermal IA 9,100 series digital melting point apparatus in capillaries and are uncorrected. IR spectra obtained in the solid state as potassium bromide discs using a Perkin-Elmer model 1430 spectrometer. 1H-NMR spectra were recorded on a Varian/Gemini 400 MHz spectrometer in DMSO-d6 as a solvent and TMS as an internal standard (chemical shifts in δ, ppm). Mass spectra measured on an instrument VG-7035 at 70 or 15 eV. Elemental analyses has performed at the Microanalytical Centre, Cairo University, and Giza, Egypt.
3.1.2. 3-amino-1,4-dihydroquinoxaline-2-carbohydrazide (2)
A solution of 1 (0.01 mol, 1.5 g) and (30 ml) ethanol absolute was added to hydrazine hydrate (99%) (0.025 moles, 2.5 ml), then the reaction mixture was refluxed for (8 hrs), after cooling the solution the residue was filtered, washed with ethanol, dried and recrystallized from ethanol gave hydrazide derivatives 2, 80 % yield, m.p 233–235. IR (KBr): ν (cm−1), 1659 (CO), 3120–3530 (NH str., NH2 str.); 1 H NMR (δ, DMSO–d6): 4.39 (s, 2H, NH2 hydraz), 5.62 (s, 2H, NH2), 7.13–8.02 (m, 4H, Ar-H), 9.36 (s, 1H, NH hydraz) 12.42 (s, 1H, N-H quinox) , 12.62 (s, 1H, N-H quinox); 13C NMR (DMSO–d6): 89.1, 119.4, 119.8, 120.3, 131.4. 136.5 (C═C) and 165.9 (CO). Anal. Calcd for (C9H11N5O, 205.22): C, 52.67; H, 5.40; N, 34.13. Found: C, 52.65; H, 5.43; N, 34.11.
3.1.3. 5-(2-Amino-1,4-dihydroquinoxalin-3-yl)-1,3,4-oxadiazole-2-thiol (3)
A mixture of 2 (0.1 mol) and KOH (0.1mole) in ethanol (50 ml) was added to carbon disulfide (8 ml). The mixture was heated under reflux until the evaluation of hydrogen sulfide was stopped. The solvent was removed and the residue was washed with water, dried and recrystallized from ethanol gave 69% yield. m.p. 275–277°C. IR (KBr): ν (cm-1) 1625–1627 (2C═N str.), 3219–3393 (2NH, NH2.); 1H NMR (400 MHz, DMSO-d6): δ 7.65–7.92 (m, 4H, Ar-H), 8.49 (s, 2H, NH2) 12.06 (s, 1H, N-H quinox), 12.21 (s, 1H, N-H quinox), 13.21(s, 1H, SH). Anal.Calcd for (C10H9N5OS, 247.05): C, 48.57; H, 3.67; N, 28.32; S, 12.97. Found: C, 48.59; H, 3.64; N, 28.34; S, 12.95.
3.1.4. (3-(5-(2,3,4,6-Tetra-O-acetyl-β-D-glucopyranosylthio)-1,3,4-oxadiazol-2-yl)-1,4-dihydroquinoxalin-2-amine (4)
To a solution of 3 (2.2 mol) in acetone (70 ml) was added KOH (0.499 mol) and 2,3,4,6-tetra-O-acetyl-α-D-glucopyranosyl bromide (3.6 mol). Stirring was continued at room temperature for 14 hrs., until reaction was complete by TLC (chloroform/methanol; 3/1). Evaporation of the solvent gave a solid and washed with water (20 ml). The solid was filtered and recrystallized by hexane yield 52%. m.p. 217–219°C. IR (KBr): ν (cm−1) 1633–1637 (2C═N str.), 3224–3382 (2NH, NH2.Str.); 1H NMR (400 MHz, DMSO-d6): δ: 1.93, 1.97, 1.99, 2.03 (4s, 12H, 4CH3CO), 3.65 (m, 1H, H-5’), 3.89 (dd, 1H, J = 3.7 Hz, J = 11.3 Hz, H-6’), 4.22 (dd, 1H, J = 3.4, J = 11.3 Hz, H6'‘), 4.56 (t, 1H, J = 9.5 Hz, H-4’), 5.98 (d, 1H, J1'−2’ = 9.32 Hz, H-1’) 7.13–8.02 (m, 4H, Ar-H), 9.36 (s, 2H, NH2) 12.42 (s, 1H, N-H quinox) , 12.62 (s, 1H, N-H quinox). Anal.Calcd for (C24H27N5O10S, 577.56). C, 49.91; H, 4.71; N, 12.13; S, 5.55. Found: C, 49.93; H, 4.70; N, 12.11; S, 5.54
3.1.5. 2,3-Dihydro-2-thioxobenzo[g]pteridin-4(1H,5H,10H)-one (5)
A solution of 1 (10 mmol) and benzoyl isothiocyanate (10 mmol) in ethanolic NaOH (0.4 g in 40 ml) was heated under reflux for 8 h. The reaction mixture was cooled and suspended in 7 ml conc. Hydrochloric acid. The residue was filtered, washed with water, and recrystallized from ethanol to give 5; mp 263–265°C. IR (KBr): ν (cm−1): 1292 (C═S), 1689(C═O), 3170–3290 (4NH); 1H NMR (δ, DMSO-d6): 7.32–7.83(m, 4H, Ar-H), 11.26 (s, 1H, N─H Pyrimi), 12.39 (s, 1H, N─H quinox), 12.73 (s, 1H, N─H quinox.,), 13.03 (s, 1H, N─H Pyrimi). Anal.Calcd for: (C10H8N4OS, 232.26). C, 51.71; H, 3.47; N, 24.12;; S, 13.81. Found: C, 1.69; H, 3.49; N, 24.15;; S, 13.83.
3.1.6. Ethyl-3-(aminoN-phenylmethanethioamino)-1,4-dihydroquinoxaline-2-carboxylate (6)
A mixture of 1 (8 mmol), phenyl isothiocyanate (8 mmol), and triethylamine (1 ml) in dioxane (35 ml) was heated under reflux for 9 h. The mixture was evaporated half of its volume; the solid was filtered, washed with ethanol, dried, and recrystallized from 1,4-dioxane to give 6. IR (KBr): ν (cm−1): 1343 (C═S), 1719 (C═O), 3270–3298 (NH)1; HNMR (δ, DMSO-d6): 2.01(s, 3H, CH3), 3.25(s, 2H, CH2), 7.23–8.28 (m, 9H, Ar-H), 8.98 (s, 1H, NHPh), 11.06 (s, 1H, N─H quinox), 12.35 (s, 1H, N─H quinox.,), Anal. Calcd for Anal.Calcd for:(C18H18N4O2S354.43): C, 61.00; H, 5.12; N, 15.81; S, 9.05. Found: C, 60.98; H, 5.11; N, 15.82; S, 9.03.
3.1.7. 2,3-Dihydro-3-phenyl-2-thioxobenzo[g]pteridin-4(1H,5H,10H)-one (7)
A mixture of 6 (10 mmol) in sodium ethoxide (0.23 g) of sodium metal in (40 ml ethanol) was heated under reflux with stirring for 9 h. After cooling, the mixture was neutralized with (10 ml) 10% HCl and the solid formed was filtered, washed with water, dried, and recrystallized from ethanol to afford 7. Yield (73%). m.p.: 254–256 oC; IR (KBr): ν (cm−1): 3353, 3210 (3NH), 1695 (CO), cm−1 ; 1 H NMR (δ, DMSO-d6): 7.54–7.97 (m, 9H, Ar–H), 9.55 (s, H, NH), 11.21 (s, 1H, NH), 12.26 (s, 1H, NH) .Anal.Calcd for (C16H12N4OS, 308.36). C, 62.32; H, 3.92; N, 18.17; S, 10.40. Found: C, 62.35; H, 3.90; N, 18.15; S, 10.38.
3.1.8. 2,3-Dihydro-3-phenyl-2(2,3,4,6-tetra-O-acetyl-β-D-glucopyranosyl Thioxobenzo[g]pteridin-4(1H,5h,10h)-one(8)
To a solution of 5 (2.77 g, 0.01 mol) in aqueous potassium hydroxide (0.56 g, 0.01 mol) in distilled water (5 ml), a solution of 2,3,4,6-tetra-O-acetyl-α-D-glucopyranosyl bromide (4.11 g, 0.011 mol) in acetone (30 ml) was added. The reaction mixture was stirred at room temperature for 14 . The solvent was evaporated under reduced pressure and the crude product was filtered off and washed with water. The solid was dried, and crystallized from diethyl ether as a brown powder. Yield (63%). m.p.: 209–211 1H NMR (δ, DMSO-d6): 2.11–2.23(4s, 12H, 4COCH3), 3.76 (m, 2H, H-6’, H-6’’), 3.82 (m, 1H, H-5’), 3.99 (m, 2H, H-4’, H-3’), 5.48 (m, 1H, H-2’), 5.72 (d, 1H, J1'-2’ = 9.7 Hz, H-1’), 6.59 (d, 2H, J = 5.8 Hz, Ar-H), 7.65 (d, 2H, J = 5.9 Hz, Ar-H), 8.1 (s, 1H, NH, Anal. Calcd for: (C24H26N4O10S, 562.55)C, 51.24; H, 4.66; N, 9.96; S, 5.70. Found: C, 51.23; H, 4.68; N, 9.98; S, 5.72.
3.1.9. 2,3-dihydro-3-phenyl-2(2,3,4,6-tetra-O-acetyl-β-D-glucopyranosyl)-thioxobenzo[g]pteridin-4(1H,5H,10H)-one (9)
To a solution of 7 (2.77 g, 0.01 mol) in aqueous potassium hydroxide (0.56 g, 0.01 mol) in water (5 ml), a solution of 2,3,4,6-tetra-O-acetyl-α-D-glucopyranosyl bromide (4.11 g, 0.011 mol) in acetone (30 ml) was added. The reaction mixture was stirred at room temperature for 14 h (under TLC control). The solvent was evaporated under reduced pressure at 40oC and the crude product was filtered off and washed with water. The residue was dried and crystallized from diethyl ether as a brown powder. Yield (59%). m.p.: 198–200 1HNMR (δ, DMSO-d6): 2.32–2.52 (4s, 12H, 4COCH3), 3.65 (m, 2H,H-6’, H-6’’), 3.71 (m, 1H, H-5’), 3.88 (m, 2H,H-4’, H-3’), 5.39 (m, 1H, H-2’), 5.69 (d, 1H, J1'-2’ = 9.8 Hz, H-1’), 6.58–7.35 (m, 2H, Ar-H). Anal.Calcd for: (C30H30N4O10S, 638.64)C, 56.42; H, 4.73; N, 8.77;S, 5.02.
3.1.10. 2-(Methylthio)benzo[g]pteridin-4(3H,5H,10H)-one (10) and 2-(methylthio)-3-phenylbenzo[g]pteridin-4(3H,5h,10h)-one (11)
A solution of absolute ethanol (20 ml) and potassium hydroxide (0.002 mol) was added to compound 5 and 7. Then the solution stirring at room temperature for 20 min, then methyl iodide added dropwise and the solution stirred at 80oC for 7 hr. The solution evaporated under vacuum pressure, the residue recrystallization by ethanol to give compounds 10 and 11 respectively. 1HNMR (δ, DMSO-d6):2.69 (s, 3H, CH3)
3.1.11. 2-Hydrazinylbenzo[g]pteridin-4(3H,5H,10H)-one (12) and 2-hydrazinyl-3-phenylbenzo[g]pteridin-4(3H,5h,10h)-one (13)
A solution of 10 and/ or 11 (0.3 g, 1 mmol) was dissolved in absolute ethanol (20 ml) and hydrazine hydrate (6 ml, 99%) added to the mixture, then the reaction mixture was heated under reflux for 7 h, the solvent evaporated under vacuum. Then the residue was recrystallized ethanol to give compound 12 and 13 respectively.
3.1.12. Compound 12
284–286 oC. IR (KBr): ν (cm−1):3325–3369 (broad NH NH2), 1688 (C = O). 1HNMR (δ, DMSO-d6): 4.45–4.63 (bs, 2H, NH2), 6.98–7.12 (m, 4H, Ar-H), 9.79 (s, H, NH), 11.23 (s, 1H, NH), 10.12 (bs, 1H, NH), 12.53 (bs, 1H, NH). Anal.Calcd for: (C10H10N6O, 230.23):C, 52.17; H, 4.38; N, 36.50. Found: C, 52.20; H, 4.36; N, 36.52 .
3.1.13. Compound 13
297–299 oC. IR (KBr): ν (cm−1): 3336–3372 (broad NH NH2), 1689 (C = O). 1HNMR (δ, DMSO-d6): 5.16 (bs, 2H, NH2),7.23–8.01 (m, 9H, Ar–H), 11.12 (s, 1H, NH), 10.07 (bs, 1H, NH). Anal.Calcd for (C16H14N6O, 306.32): C, 62.74; H, 4.61; N, 27.44. Found: C, 62.71; H, 4.63; N, 27.46.
3.1.14. 2-Glucosehydrazonebenzo[g]pteridin-4(3H, 5h, 10h)-one (14) and 2-glucosehydrazone-3-phenylbenzo[g]pteridin-4(3H, 5H, 10h)-one (15)
A solution of 12 and /or 13 (1 mmol), D-glucose (1.80 g,1 mmol), ethanol (30 ml) and few drops of glacial acetic acid used as catalyst was heated at 80oC for 4 h. The solid was filtered off, dried and recrystallized from ethanol to yield compound 14 and 15.Yield 56%, 53% respectively.
3.1.15. Compound 14
193–195°C. IR (KBr): ν (cm−1): 3357–3238 ( NH), 1688 (C = O), 1672 (C = N). 1HNMR (δ, DMSO-d6, ppm): 3.32–3.64 (protons of sugar moeity) 3.93 (m, 1H, OH), 4.35 (m, 1H,OH), 4.58 (m, 1H, OH), 4.90 (m, 1H, OH), 5.12 (m, 1H, OH), 7.14–7.25 (m, 5H, 4Ar-H, NH), 8.59 (m,1H, N = CH), 12.42 (s, 1H, N─H quinox), 12.83 (s, 1H, N─H quinox.), 13.13 (s, 1H, N─H Pyrimi). Anal. calcd for Anal. calcd for C16H20N6O6 (392.37): C, 48.98; H, 5.14; N, 21.42. Found: C, 48.96; H, 5.17; N, 21.44.
3.1.16. Compound 15
210–212oC. IR (KBr): ν (cm−1): 3266–3338 ( NH), 1687 (C = O), 1669 (C = N). 1HNMR (δ, DMSO-d6, ppm): 3.93 (m, 1H, OH), 4.20 (m, 1H, OH), 4.45 (m, 1H, OH), 4.93 (m, 1H, OH), 5.25 (m, 1H, OH), 7.19–7.32 (m, 10H, 4Ar-H, NH), 9.32 (m, 1H, N = CH), 11.31 (s, 1H, NH), 12.36 (s, 1H, NH). Anal. calcd for C22H24N6O6 (468.46): C, 56.40; H, 5.16; N, 17.94. . Found: C, 56.42; H, 5.18; N, 17.92.
3.1.17. (1S)-1,2,3,4,5-Penta-O-acetyl-1-C-(1-acetyl [1,2,4]triazolo[4,3-a] 2,3-Dihydro-2-thioxobenzo[g]pteridin-4-one (16)
A mixture of 14 (0.5 g,1 mmol) dissolved in acetic anhydride(10 ml) stirred on water bath for 11 h. The solution cooled and poured into ice-water with stirring and the precipitated collected by filtration, washed with water, dried and recrystallized from ethanol to produce compound 16.Yield 63%, m.p. 227–229oC. IR (KBr): ν (cm−1): 1748(C = O), 1687 (C = O), 1609 (C = N). 1HNMR (δ, DMSO-d6):1.93–2.24 (s, 15H, 5OCO(CH3)), 2.32 (s, 3H, COCH3), 4.23 (m, 1H, CHOAc), 5.13–5.22 (m, 2H, 2CHOAc), 6.31 (m,1H, CHOAc), 6.89–7.03 (m, 4H, 4Ar-H), 11.31 (s, 1H, NH), 12.36 (s, 1H, NH). Anal.calcd C28H30N6O12, 642.57): C, 52.34; H, 4.71; N, 13.08. Found: C, 52.36; H, 4.69; N, 13.06.
3.2. Molecular docking study
The crystal structures of bromocelecoxib bound at COX-2 isoform (Protein Data Bank; PDB: ID 1CX2). Docking was done using London dG force and sophistication of the results was performed using force field energy. Preparation of the synthesized compounds for docking was attained via their 3D structure built by Molecular Operating Environment (MOE, Version 2005.06, Chemical Computing Group Inc., QC, Canada). Definite procedures were in use before docking which includes: 3D protonation of the structures, running conformational analysis using systemic search, selecting the least energetic conformer and applying the same docking protocol used with ligands. Docking for the synthesized compounds was applied.
Acknowledgements
The author thankful to College of Science and Pharmacy, Jouf University, Sakaka, Kingdom of Saudi and Chemistry Department, Faculty of Science, Zagazig University, Zagazig, Egypt.Faculty of Science, Aswan University, Egypt and Faculty of Pharmacy, Beni Suef University.
Disclosure statement
No potential conflict of interest was reported by the authors.
ORCID
Amira A. Ghoneim http://orcid.org/0000-0003-0194-5017
Nadia Ali Ahmed Elkanzi http://orcid.org/0000-0002-1687-1834
Rania B. Bakr http://orcid.org/0000-0001-6564-1712
References
- Xiang J, Zheng L, Chen F, et al. A cascade reaction consisting of pictet- spengler-type cyclization and smiles rearrangement: application to the synthesis of novel pyrrole-fused dihydropteridines. Org Lett. 2007;9:765–767. doi: 10.1021/ol0629364
- Asif M. The pharmacological importance of some diazine containing drug molecules. Sop Trans. Org. Chem. 2014;1:1–10. doi: 10.15764/STAC.2014.01001
- Rinderspacher A. Six-Membered ring systems: diazines and benzo derivatives, progress in heterocyclic chemistry. Elsevier. 2013;25:357–390.
- Gourley DG, Schüttelkopf AW, Leonard GA, et al. Pteridine reductase mechanism correlates pterin metabolism with drug resistance in trypanosomatid parasites. Nat Struct Mol Biol. 2001;8:521–525. doi: 10.1038/88584
- Schüttelkopf AW, Hardy LW, Beverley SM, et al. Structures of leishmania major pteridine reductase complexes reveal the active site features important for ligand binding and to guide inhibitor design. J Mol Biol. 2005;352:105–116. doi: 10.1016/j.jmb.2005.06.076
- Vickers TJ, Orsomando G, de la Garza RD, et al. Biochemical and genetic analysis of methylenetetrahydrofolate reductase in leishmania metabolism and virulence. J Biol Chem. 2006;281:38150–38158. doi: 10.1074/jbc.M608387200
- Voet D, Voet J, Pratt C. Electron transport, and oxidative phosphorylation, biochemistry. 3rd ed. Hoboken (NJ): John Wiley & Sons; 2004; 797–842 DOI.
- Cushman M, Patel HH, Scheuring J, et al. Fluorine-19 NMR studies on the mechanism of riboflavin synthase. synthesis of 6-(trifluoromethyl)-7-oxo-8-(D-ribityl) lumazine and 6-(trifluoromethyl)-7-methyl-8-(D-ribityl) lumazine. J Org Chem. 1992;57:5630–5643. doi: 10.1021/jo00047a015
- Climent T, González-Luque R, Merchán M, et al. Theoretical insight into the spectroscopy and photochemistry of isoalloxazine, the flavin core ring. J Phys Chem A. 2006;110:13584–13590. doi: 10.1021/jp065772h
- Goodwill KE, Sabatier C, Stevens RC. The crystal structure of tyrosine hydroxylase with bound cofactor analog and iron at 2.3 Å resolution: self-hydroxylation of Phe300 and the pterin-binding site. Biochem. 1998;37:13437–13445. doi: 10.1021/bi981462g
- Negishi E-i, Hu Q, Huang Z, et al. Palladium-catalyzed alkenylation by the negishi coupling. Aldrichim Acta. 2005;38:71–87.
- Venters D. Folate synthesis in Ae. aegypti and drosophila melanogaster larvae. Trans R Soc Trop Med Hyg. 1971;65:687–688. doi: 10.1016/0035-9203(71)90063-0
- Xu H, Fan L-l. Synthesis and antifungal activities of novel 5, 6-dihydro-indolo [1, 2-a] quinoxaline derivatives. Eur J Med Chem. 2011;46:1919–1925. doi: 10.1016/j.ejmech.2011.02.035
- Corbett TH, LoRusso P, Demchick L, et al. Preclinical antitumor efficacy of analogs of XK469: sodium-(2-[4-(7-chloro-2-quinoxalinyloxy) phenoxy] propionate. Invest New Drugs. 1998;16:129–139. doi: 10.1023/A:1006174622061
- Budakoti A, Bhat AR, Azam A. Synthesis of new 2-(5-substituted-3-phenyl-2-pyrazolinyl)-1, 3-thiazolino [5, 4-b] quinoxaline derivatives and evaluation of their antiamoebic activity. Eur J Med Chem. 2009;44:1317–1325. doi: 10.1016/j.ejmech.2008.02.002
- Tandon VK, Yadav DB, Maurya HK, et al. Design, synthesis, and biological evaluation of 1, 2, 3-trisubstituted-1, 4-dihydro benzo [g] quinoxaline-5, 10-diones and related compounds as antifungal and antibacterial agents. Bioorg Med Chem. 2006;14:6120–6126. doi: 10.1016/j.bmc.2006.04.029
- Carta A, Paglietti G, Nikookar MER, et al. Novel substituted quinoxaline 1, 4-dioxides with in vitro antimycobacterial and anticandida activity. Eur J Med Chem. 2002;37:355–366. doi: 10.1016/S0223-5234(02)01346-6
- Hui X, Desrivot J, Bories C, et al. Synthesis and antiprotozoal activity of some new synthetic substituted quinoxalines. Bioorg Med Chem Lett. 2006;16:815–820. doi: 10.1016/j.bmcl.2005.11.025
- Noolvi MN, Patel HM, Bhardwaj V, et al. Synthesis and in the vitro antitumor activity of substituted quinazoline and quinoxaline derivatives: search for an anticancer agent. Eur J Med Chem. 2011;46:2327–2346. doi: 10.1016/j.ejmech.2011.03.015
- Abu-Hashem A, Gouda M, Badria F. Synthesis of some new pyrimido [2′, 1′: 2, 3] thiazolo [4, 5-b] quinoxaline derivatives as anti-inflammatory and analgesic agents. Eur J Med Chem. 2010;45:1976–1981. doi: 10.1016/j.ejmech.2010.01.042
- Wagle S, Adhikari AV, Kumari NS. Synthesis of some new 2-(3-methyl-7-substituted-2-oxoquinoxalinyl)-5-(aryl)-1, 3, 4-oxadiazoles as potential non-steroidal anti-inflammatory and analgesic agents. Ind J Chem. 2008;47:439–448.
- Singh DP, Hashim SR, Singhal RG. Anti-inflammatory activity of some new thioether derivatives of quinoxaline. Int. J. Drug Dev. Res. 2010;2:810–815.
- Bakr RB, Azouz AA, Abdellatif KR. Synthesis, cyclooxygenase inhibition, anti-inflammatory evaluation and ulcerogenic liability of new 1-phenylpyrazolo [3, 4-d] pyrimidine derivatives. J Enzyme Inhib Med Chem. 2016;31:6–12. doi: 10.1080/14756366.2016.1186018
- Abdelgawad MA, Bakr RB, El-Gendy AO, et al. Discovery of a COX-2 selective inhibitor hit with anti-inflammatory activity and gastric ulcer protective effect. Future Med Chem. 2017;9:1899–1912. doi: 10.4155/fmc-2017-0115
- Abdelgawad MA, Bakr RB, Alkhoja OA, et al. Design, synthesis and antitumor activity of novel pyrazolo [3, 4-d] pyrimidine derivatives as EGFR-TK inhibitors. Bioorg Chem.. 2016;66:88–96. doi: 10.1016/j.bioorg.2016.03.011
- Bakr RB, Mehany ABM, Abdellatif KRA. Synthesis, EGFR inhibition and anti-cancer activity of New 3, 6-dimethyl-1-phenyl-4-(substituted-methoxy) pyrazolo [3, 4-d] pyrimidine derivatives. Anti-Cancer Agents in Medicinal Chemistry (Formerly Current Medicinal Chemistry-Anti-Cancer Agents). 2017;17:1389–1400.
- Abdellatif KRA, Abdelall EKA, Bakr RB. Nitric oxide-NASIDS donor prodrugs as hybrid safe anti-inflammatory agents. Curr Top Med Chem. 2017;17:941–955. doi: 10.2174/1568026616666160927153435
- Khalafallah A, Abd El-Aal R, El-Kanzi N. Synthesis of several polyfunctionally substituted fused pyrimidines incorporating quinone compounds. J Chin Chem Soc. 2004;51:769–774. doi: 10.1002/jccs.200400116
- Soleiman H, Elkanzi N. Heterocyclic synthesis containing New spiro, isolated (β-Lactam and Thiazolidinone) 3-cyano-2, 4-diamino thiophene derivatives. Phosphorus, Sulfur, and Silicon. 2008;183:1679–1690. doi: 10.1080/10426500701724548
- El Azab IH, Elkanzi NA, Gobouri AA. Design and synthesis of some New quinoxaline-based heterocycles. J Heterocycl Chem. 2018;55:65–76. doi: 10.1002/jhet.2978
- Harizi H, Corcuff J-B, Gualde N. Arachidonic-acid-derived eicosanoids: roles in biology and immunopathology. Trends Mol Med. 2008;14:461–469. doi: 10.1016/j.molmed.2008.08.005
- Tzeng S-F, Hsiao H-Y, Mak O-T. Prostaglandins and cyclooxygenases in glial cells during brain inflammation. Current Drug Targets-Inflammation & Allergy. 2005;4:335–340. doi: 10.2174/1568010054022051
- Leone S, Ottani A, Bertolini A. Dual acting anti-inflammatory drugs. Curr Top Med Chem. 2007;7:265–275. doi: 10.2174/156802607779941341
- Dogné J-M, Supuran CT, Pratico D. Adverse cardiovascular effects of the coxibs. J Med Chem.. 2005;48:2251–2257. doi: 10.1021/jm0402059
- Sigthorsson G, Crane R, Simon T, et al. COX-2 inhibition with rofecoxib does not increase intestinal permeability in healthy subjects: a double blind crossover study comparing rofecoxib with placebo and indomethacin. Gut. 2000;47:527–532. doi: 10.1136/gut.47.4.527
- Antman EM, Bennett JS, Daugherty A, et al. Use of nonsteroidal antiinflammatory drugs: an update for clinicians: a scientific statement from the American heart association. Circulation. 2007;115:1634–1642. doi: 10.1161/CIRCULATIONAHA.106.181424
- Dogne J-M, Hanson J, Supuran C, et al. Coxibs and cardiovascular side-effects: from light to shadow. Curr Pharm Des. 2006;12:971–975. doi: 10.2174/138161206776055949
- Weber A, Casini A, Heine A, et al. Unexpected nanomolar inhibition of carbonic anhydrase by COX-2-selective celecoxib: new pharmacological opportunities due to related binding site recognition. J Med Chem. 2004;47:550–557. doi: 10.1021/jm030912m
- McGettigan P, Henry D. Cardiovascular risk with non-steroidal anti-inflammatory drugs: systematic review of population-based controlled observational studies. PLoS Med. 2011;8:e1001098. doi: 10.1371/journal.pmed.1001098
- Trelle S, Reichenbach S, Wandel S, et al. Cardiovascular safety of non-steroidal anti-inflammatory drugs: network meta-analysis. BMJ. 2011;342:c7086. doi: 10.1136/bmj.c7086
- Habeeb AG, Praveen Rao P, Knaus EE. Design and synthesis of celecoxib and rofecoxib analogues as selective cyclooxygenase-2 (COX-2) inhibitors: replacement of sulfonamide and methylsulfonyl pharmacophores by an azido bioisostere. J Med Chem. 2001;44:3039–3042. doi: 10.1021/jm010153c