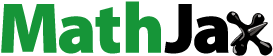
Abstract
The ever-increasing spread of bacterial infection with rising antibacterial drug resistance has become a matter of global concern in the modern times. Therefore, such serious infections leading to high mortality rate has necessitated the search for new classes of effective antibacterial agents with minimum toxicity. Herein, we report the synthesis and identification of a new class of benzofuran based Schiff ligands (6a-6e) and their Cu/Zn complexes (7a-7j) via sequential reactions starting with Salicylaldehyde. Compounds 6a, 7c and 7e exhibit activity in the low microgram range against M. tuberculosis, showing the minimum inhibitory concentrations (MICs) of 1.6 μg/mL. The compounds 6e and 7b also show interesting antibacterial activity against S. Aureus, E. coli and B. Subtilis with comparable area of inhibition of standard drugs under study. This research suggests that benzofuran based compounds can serve as a promising lead scaffold in developing new drugs to combat microbial infections.
GRAPHICAL ABSTRACT
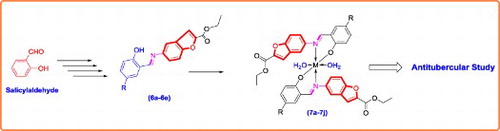
1. Introduction
The increase in drug resistance to clinically used anti-infective agents reveals that there is an urgent need of the search for new antimicrobial compounds to treat multi-resistant infections with different mechanism of action [Citation1].
Schiff base [Citation2] is analogous to aldehyde or ketone in which the carbonyl group (C═O) is replaced by an azomethine or imine group. The Schiff bases found number of applications as catalysts, dyes and pigments, polymer stabilizers, intermediates in synthesis, etc. [Citation3]. The Schiff bases also exhibit a broad range of biological activities like antibacterial, antifungal, antimalarial, antituberculosis, antipyretic, anti-inflammatory and antiviral properties [Citation4]. The imines are present in various natural and synthetic compounds. The imine group from Schiff base has been shown to be critical towards biological activities [Citation5].
Benzofuran is a fundamental structural unit in a variety of biologically active natural products as well as synthetic materials [Citation6]. Benzofuran derivatives shows several biological properties such as anti-inflammatory, antimicrobial, antifungal, antihyperglycemic, analgesic, antiparasitic, and antitumor activities [Citation7–12]. Such a wide range of biological properties inherent in benzofuran scaffold justifies their extensive interest in pharmacological applications. A large number of clinically approved drugs are synthetic or naturally occurring substituted benzofuran derivatives, some of which are fused with other heterocyclic moieties [Citation13]. In addition, substituted benzofurans find application such as of fluorescent sensor [Citation14], oxidant [Citation15], antioxidants, brightening agents, a variety of drugs and in other field of chemistry and agriculture [Citation16].
Recently, it has been reported that Schiff bases and their metal complexes demonstrated significant potency against the MTB, at low micromolar level [Citation17–19]. Numerous examples of Cu (II) and Zn (II) complexes have been explored for their significant antimicrobial activity [Citation20].
Based on these facts, supported by literature [Citation21] Figure and in continuation of our current research interest in the field of synthesis and antimicrobial study of heterocyclic compounds [Citation22–26], we have synthesized and screened the antimicrobial activity of series of transition metal complexes of new Schiff base ligands derived from ethyl 5-aminobenzofuran-2-carboxylate.
2. Material and methods
All required chemicals and solvents were purchased from Sigma-Aldrich (Munich, Germany) and Merck Co. (Darmstadt, Germany) and used without further purification. Melting points were determined with Fisher–Johns blocks (Fisher Scientific, Germany) and are uncorrected. The NMR spectra were recorded on a Bruker Avance 300 apparatus in DMSO-d6. The chemical shifts are measured on the δ (ppm) scale using TMS (Tetramethylsilane) as the internal standard reference. Infrared (IR) spectra measured on a FTIR-7600 Lambda Scientific Pty. Ltd. using KBr disk for the range 4000–400 cm−1. Mass spectra obtained on BRUKER ESQUIRE HCT spectrometer. The TGA (Thermo gravimetric analysis) study carried out on Universal V4.5A TA instrument
3. Experimental
3.1. Preparation of 5-nitro salicylaldehyde (2)
The solution of salicylaldehyde (1) (1 mmol) in acetic acid (10 cm3) was allowed to react with nitric acid (5 cm3) at 0°C. Then reaction mixture was stirred at RT for 4 h to produce 5-nitro salicylaldehyde. The reaction mixture diluted with ice-cold water. The resulting solid was filtered and dried. White solid; Yield: 95%; m.p.: 125–127°C.
3.2. Preparation of ethyl 5-nitrobenzofuran-2-carboxylate (3)
To the solution of 5-nitro salicylaldehyde (1 mmol) in N-methyl pyrrolidine (NMP) (8 cm3), Na2CO3 (2.5 mmol) was added stirred for 30 min., followed by addition of ethyl bromo acetate (1.5 mmol) and stirred for overnight at 100°C. The reaction mixture was dissolved in water and then extracted with ethyl acetate. The combined organic layer was washed with brine solution, dried over Na2SO4 and then evaporated to dryness. Off White solid; Yield: 88%; m.p.: 176–178°C.
3.3. Preparation of ethyl 5-aminobenzofuran-2-carboxylate (4)
To the solution of ethyl 5-nitrobenzofuran-2-carboxylate (3) (1 mmol) in ethanol solvent under N2 atmosphere Raney-Ni (3.0 mmol) was added to the reaction mass. Then put H2 pressure. The reaction mixture was stirred for 6 h at room temperature. The reaction mixture was filter through the high-flow; the combined organic layer was evaporated to dryness. Yellow solid, 91% yield, m.p.: 189–191°C; IR(KBr, υ, cm−1): 3379 (-NH2), 3309 (-NH2), 2924 (aliphatic –CH–), 1711(C═O); 1H-NMR (300 MHz, CDCl3): δ = 7.50 (s, 1H, Ar–H), 7.36 (d, J = 9.6 Hz, 1H, Ar–H), 6.80 (m, 2H, Ar–H), 5.08 (s, 2H, NH2), 4.31 (q, J = 7.5, 14.4 Hz, 2H, O–CH2–), 1.31 (m, 3H, –CH3); 13C-NMR (75 MHz, DMSO + CDCl3): 157.09 (C═O), 144.04, 140.91, 140.28, 122.70, 112.11, 108.35, 106.70, 99.24, 55.79 (O–CH2–), 8.64 (–CH3); EIMS m/z: 205.2. Found: 206.4, (M+). Anal. Calcd. for C11H11NO3: C, 64.38; H, 5.40; N, 6.83. Found: C, 64.43; H, 5.38; N, 6.80.
3.4. General procedure for the preparation of Schiff bases (6a–6e)
The compound (4) (0.01 mol) and appropriate aldehyde derivatives (5a–5e) (0.01 mol) taken in round bottom flask (RBF) containing 10 cm3 of methanol. Reaction mixture was refluxed for 30 min. Completion of reaction was checked with TLC. Upon cooling the reaction mixture a solid product (6a–6e) (Figure and Table ) precipitated out, which was filtered, dried and purified by recrystallization from ethanol.
Table 1. Structures of Schiff bases (6a–6e).
3.4.1 (E)-ethyl 5-(2-hydroxybenzylideneamino) benzofuran-2-carboxylate (6a)
Light green solid (EtOH); mp 130–132°C; IR(KBr, υ, cm−1): 3360 (Ar–OH), 1750 (C═O), 1620 (CH═N); 1H-NMR (DMSO-d6, 300 MHz): δ = 12.99 (s, 1H, Ar–OH), 8.99 (s, 1H, –CH═N), 7.77 (m, 3H, Ar–H), 7.62 (m, 2H, Ar–H), 7.41 (m, 1H, Ar–H), 6.95 (m, 2H, Ar–H), 4.35 (m, 2H, O–CH2), 1.32 (t, 3H, –CH3); EIMS m/z: 310.32 [M + H]; Anal. Calcd. for C18H15NO4: C, 69.89; H, 4.89; N, 4.53; Found: C, 69.24; H, 4.97; N, 4.65.
3.4.2 (E)-ethyl5-(5-chloro-2-hydroxybenzyli- deneamino)benzofuran-2-carboxylate (6b)
Yellow solid (EtOH); mp 162–164°C; IR(KBr, υ, cm−1): 3445 (Ar–OH), 1771(C═O), 1618(CH═N); 1H-NMR (DMSO-d6, 300 MHz): δ = 12.93 (s, 1H, Ar–OH), 8.99 (s, 1H, CH═N), 7.80 (m, 4H, Ar–H), 7.62 (m, 1H, Ar–H), 7.45 (m, 1H, Ar–H), 7.01 (m, 1H, Ar–H), 4.36 (m, 2H, O–CH2–), 1.34 (t, 3H, –CH3); 13C-NMR (DMSO-d6, 75 MHz) δ: 161.82 (C–OH), 158.75 (C═O), 158.45 (CH═N), 153.99, 146.19, 144.50, 132.65, 130.86, 127.48, 122.55, 122.26, 120.64, 118.58, 115.04, 114.24, 112.98, 61.29 (O–CH2–), 14.07 (–CH3); EIMS 343.76 m/z: 344.71 [M + H] (100); Anal. Calcd. for C18H14ClNO4: C, 62.89; H, 4.10; N, 4.07; Found: C, 62.78; H, 4.04; N, 3.98.
3.4.3. (E)-ethyl5-(5-bromo-2-hydroxybenzyli- deneamino)benzofuran-2-carboxylate (6c)
Pale yellow solid (EtOH); mp 155–157°C; IR(KBr, υ, cm−1): 3375 (Ar–OH), 1770 (C═O), 1619 (CH═N); 1H-NMR (DMSO-d6, 300 MHz): δ = 12.96 (s, 1H, Ar–OH), 8.98 (s, 1H, CH═N), 7.83 (m, 4H, Ar–H), 7.58 (m, 2H, Ar–H), 6.95 (m, 1H, Ar–H), 4.36 (m, 2H, O–CH2), 1.34 (t, 3H, –CH3); EIMS (388.21) m/z: 389.05 [M + H]; Anal. Calcd. for C18H14BrNO4: C, 55.69; H, 3.63; N, 3.61; Found: C, 55.76; H, 3.46; N, 3.58.
3.4.4. (E)-ethyl5-(2-hydroxy-5-nitrobenzyli- deneamino)benzofuran-2-carboxylate (6d)
Orange yellow solid (EtOH); mp 190–192°C; IR(KBr, υ, cm−1): 3446 (Ar–OH), 1741 (C═O), 1624 (CH═N); 1H-NMR (DMSO-d6, 300 MHz): δ = 12.95 (s, 1H, Ar–OH), 9.21 (s, 1H, CH═N), 8.65 (s, 1H, Ar–H), 8.25 (m, 1H, Ar–H), 8.08 (m, 1H, Ar–H), 7.73 (m, 3H, Ar–H), 7.07 (m, 1H, Ar–H), 4.34 (m, 2H, O–CH2–), 1.33 (t, 3H, –CH3); 13C-NMR (DMSO-d6, 75 MHz) δ: 166.72 (C–OH), 163.15 (C═O), 161.69 (CH═N), 158.80, 154.66, 146.84, 138.90, 129.80, 128.47, 127.92, 123.03, 121.31, 119.24, 118.53, 117.73, 117.19, 61.78 (O–CH2–), 14.48 (–CH3); EIMS m/z: 355.33 [M + H] (100); Anal. Calcd. for C18H14N2O6: C, 61.02; H, 3.98; N, 7.91; Found: C, 60.94; H, 4.03; N, 8.01.
3.4.5. (E)-ethyl5-((1-hydroxynaphthalen-2-yl)methyleneamino)benzofuran-2-carboxylate (6e)
Dark yellow solid (EtOH); mp 118–120°C; IR(KBr, υ, cm−1): 3382 (Ar–OH), 1760 (C═O), 1625 (CH═N); 1H-NMR (DMSO-d6, 300 MHz): δ = 15.76 (s, 1H, Ar–OH), 9.72 (s, 1H, CH═N), 8.51 (s, 1H, Ar–H), 7.97 (m, 2H, Ar–H), 7.81 (m, 4H, Ar–H), 7.54 (m, 1H, Ar–H), 7.35 (m, 1H, Ar–H), 7.05 (m, 1H, Ar–H), 4.36 (m, 2H, O–CH2), 1.33 (m, 3H, –CH3); 13C-NMR (DMSO-d6, 75 MHz) δ: 15.76 (s, 1H, C–OH), 9.72 (s, 1H, CH═N), 8.51 (s, 1H, Ar–H), 7.97 (m, 2H, Ar–H), 7.81 (m, 4H, Ar–H), 7.54 (m, 1H, Ar–H), 7.35 (m, 1H, Ar–H), 7.05 (m, 1H, Ar–H), 4.36 (m, 2H, O–CH2), 1.33 (m, 3H, –CH3); EIMS m/z: 360.1 [M + H]; Anal. Calcd. for C22H17NO4: C, 73.53; H, 4.77; N, 3.90; Found: C, 73.61; H, 4.86; N, 3.95.
3.5. General procedure for the preparation of metal complexes (7a–7j)
A solution of metal chloride (CuCl2/ZnCl2) in methanol was added gradually to a stirred ethanolic solution of the Schiff base ligand (6a–6e) in the molar ratio 1:2. The reaction mixture was further stirred for 2 h at 78°C. Then it was cooled in ice bath to ensure the complete precipitation of the formed complexes (7a–7j) Figure and Table . The precipitated solid complex was filtered and washed with water. Finally, the complex was washed with diethyl ether and dried in vacuum desiccators over anhydrous CaCl2.
Table 2. Structures of metal complexes (7a–7j).
3.5.1. Cu (II) complex (7a)
IR(KBr, υ, cm−1): 3430, 1751, 1624, 1530, 1410, 520, 420.
3.5.2. Cu (II) complex (7b)
IR(KBr, υ, cm−1): 3420, 3360, 1769, 1520, 1420, 530, 430.
3.5.3. Cu (II) complex (7c)
IR(KBr, υ, cm−1): 3447, 3352, 1773, 1608, 1413, 540, 522, 440; EIMS m/z [M + 3]: 876.8.
3.5.4. Cu (II) complex (7d)
IR(KBr, υ, cm−1): 3430, 1750, 1620, 1420, 520, 430.
3.5.5. Cu (II) complex of (7e)
IR(KBr, υ, cm−1): 3440, 1761, 1615, 1422, 530, 425.; EIMS m/z [M + 2]: 835.17
3.5.6. Zn (II) complex (7f)
IR(KBr, υ, cm−1): 3393, 3108, 1752, 1604, 1589, 1459, 1435, 536, 454.
3.5.7. Zn (II) complex (7g)
IR(KBr, υ, cm−1): 3422, 1769, 1619, 1599, 1451, 1406, 537, 486, 457.
3.5.8. Zn (II) complex (7h)
IR(KBr, υ, cm−1): 3421, 3104, 1770, 1605, 1589, 1452, 1407, 535, 527, 485, 447 cm−1; 1H-NMR (DMSO-d6, 300 MHz): δ = 9.29 (s, 2H), 7.84(m, 2H), 7.69(m, 2H), 7.60(s, 2H), 7.52(s, 2H), 7.46(m, 2H), 7.28(m, 2H), 6.72(m, 2H), 4.36(m, 4H), 1.34(t, 6H); EIMS m/z: 876.2 [M + 1].
3.5.9. Zn (II) complex (7i)
IR(KBr, υ, cm−1): 3403, 1752, 1619, 1608, 1473, 1449, 527, 450, 436.
3.5.10. Zn (II) complex (7j)
IR(KBr, υ, cm−1): 3470, 3439, 1762, 1617, 1601, 1459, 1426, 536, 463, 453; EIMS m/z [M + 1]: 819.1.
4. Results and discussion
4.1. Chemistry
The main purpose of this work was the synthesis, identification and in vitro antimicrobial screening of newer Schiff bases along with their Cu (II) and Zn (II) complexes. Scaffold ethyl 5-aminobenzofuran-2-carboxylate (4) was prepared by reported procedure [Citation21]; in brief synthesis began by nitrating the salicylaldehyde (1). The 2-hydroxy-5-nitrobenzaldehyde (2) on subsequent treatment with ethyl bromoacetate in presence of sodium carbonate in N-methyl pyrrolidine gave ethyl 5-nitrobenzofuran-2-carboxylate (3) in good yields and purity. Subsequent reduction of the nitro group at the 5th position of the benzofuran core gave the corresponding ethyl 5-aminobenzofuran-2-carboxylate (4) in excellent yields. This compound (4) on further condensed with 2-hydroxybenzaldehyde derivatives using acetic acid as catalyst in ethanol gave the scaffold (6a–6e). The final complexes (7a–7j) were then assembled by coupling the so obtained Schiff bases (6a–6e) with Cu (II) and Zn (II) as depicted in Figure .
All the synthesized Schiff bases, Cu (II) and Zn (II) complexes are stable solids and soluble in DMSO at room temperature. The spectral characterization (1H-NMR, 13C-NMR, Mass, XRD, TGA, ESR) confirm the molecular structures of desired compounds.
The 1H-NMR spectrum of the Schiff bases were scanned in the range of 0–16 ppm. The 1H-NMR spectrum of Schiff base 6b showed a singlet at 8.98 ppm. This is assigned to the imine –CH═N– proton. The presence of this peak with a slight shift in the zinc complex 7h at 9.29 ppm, suggests the proposed mode of coordination. A singlet observed at 12.93 ppm is attributed to the phenolic –OH proton. This peak has disappeared in the case of zinc complex 7h, due to deprotonation. The aromatic protons have resonated in the region 7.01–7.80 ppm. The triplet signals at 1.34 ppm and multiplate at 4.36 ppm are assigned to methyl (–CH3) and methylene (–O–CH2–) protons respectively. While in the case of zinc complex 7h, all these proton resonances have shown significant shifts, indicating the complexation. The EIMS spectra of 7h shows peak at 876.2 for molecular ion peak [M + 1].
The 13C NMR of Schiff base 6b has 18 carbon resonances. The spectrum has shown characteristic signals at 158.45 and 158.750 ppm are assigned to azomethine and carbonyl carbons respectively. Aromatic carbons have resonated in the expected region of 112.98–146.19 ppm. The peak at 61.29 and 14.07 ppm are assigned to methylene carbon (O–CH2–) and methyl carbon (–CH3) respectively.
The FTIR spectrum of the Schiff base 6b shows a broad band at 3445 cm−1, assigned to υ(OH) of the phenolic group. This band has disappeared in all the complexes indicating the coordination of phenolic oxygen via de-protonation. This fact is even confirmed by the shift of phenolic (C–O) vibrations in the ligand, from 1413 cm−1 to higher frequencies in all the complexes. However, the complexes have shown new broad peaks in the region of 3393–3447 cm−1, assignable to coordinated water. The vibrations at 1769 cm−1and 1619 cm−1 in the ligand are due to ester carbonyl υ(C═O) and imine υ(C═N) respectively. The imine υ(C═N) vibrations have shifted to lower frequencies in all the complexes, indicating the participation of phenolic oxygen and imine nitrogen in the coordination. The key IR absorption bands are summarized in Table .
Table 3. Spectral data of Schiff bases (6a–6e) and metal complexes (7a–7j).
The thermal behaviour of some selected metal complexes was characterized on the basis of TGA method. The thermal behaviour of the metal complexes was studied in temperature range of 25–1000°C. The Cu (II) complex 7a decomposed in four steps. The first step occurred bellow 100°C and corresponded to the loss of water molecules of Lattice water. The second step of decomposition appeared within the temperature ranges 110–160°C and corresponded to the loss of coordinated water molecules. The organic ligand was further decomposed within the temperature range 400°C leaving behind CuO as final product. This pattern of observation of TGA found similar for other complexes including Zn (II) complexes. The platue observed for Zn (II) complexes above the 400°C is due to formation of stable ZnO. The molar conductivity values were measured at room temperature in DMF (10−3 M) for all the complexes. The values obtained fall in the range of 8.48–20.2 indicating non-electrolytic nature of the complexes [Citation27]. The molar conductivity data is represented in Table .
The ESR spectrum of the Cu (II) complex in a polycrystalline state was recorded at room temperature. The gll and g⊥ values for 7c, 7d and 7e are reported in the Table . The gavg value is calculated and reported in the same table. The spectrum showed asymmetric bands with two g values. The values are almost same for all complexes indicating that the type of bonding is the same in all complexes and that the Cu-O bond lengths do not vary much from complex to complex [Citation28,Citation29].
Table 4. ESR spectral data of Cu (II) complexes.
The trend gll> g⊥> 2.00277, indicates that the unpaired electron lay predominately in the dx2-y2 orbital with possibly mixing of orbital because of the low symmetry.
The axial symmetry parameter “G” is determined as
G values found to be more than 4 suggesting very weak or no interaction in the solid state.
The results of elemental analyses and spectral characterization of the prepared Schiff bases and their metal complexes are in satisfactory agreement with the theoretical values which confirm the proposed molecular formula of these compounds as represented in Tables and .
5. Microbiology
5.1. In vitro antitubercular assay
The anti-tubercular activity of synthesized compounds was evaluated using previously reported method Almar Blue assay (MABA) [Citation30]. A blue colour in the well was interpreted as no bacterial growth, and pink colour was scored as growth. From this experiment, MIC (minimum inhibitory concentration) can be defined as lowest drug concentration which prevented the colour change from blue to pink. The observed results are represented in Table and Figure .
Table 5. Anti tuberculosis study of Schiff bases (6a–6e) and metal complexes (7a–7j) with MIC.
The hydroxyl fatty acids like Mycolic acids present in the mycobacterial cell wall are significant key structure for survival and growth of M. tuberculosis bacteria. Majority of first line drugs against M. tuberculosis works by inhibiting biosynthesis of mycolic acids leading to mycobacterial cell death. Recent advancements revealed Schiff bases derivatives as more potent compound against M. tuberculosis H37 RV strain with minimum MIC value. Enticed by these reports, all synthesized compounds were evaluated against M. tuberculosis (H37 RV strain ATCC No- 27294) by Microplate Alamar Blue Assay (MABA). All compounds exhibited moderate to good antitubercular activity as compared to standard drugs. At 1.60 µg/mL concentration compounds 6a, 7c and 7e showed inhibitory activity against M. tuberculosis.
5.2. In vitro antibacterial assay
The test compounds were screened in vitro for antibacterial activity against S. Aureus, E. coli and B. Subtilis. The effect of test compounds on the several bacterial strains were assayed by Disc diffusion method. The compounds are allowed to diffuse out into the medium and interact in a plate freshly seeded with the test organisms. The resulting zones of inhibition shall be uniformly circular as there will be a confluent lawn of growth. The diameter of zone of inhibition can be measured in cm.
Petriplates containing 20 mL Muller Hinton medium were seeded with 24 h culture of bacterial strains. The solutions of concentration 200 and 100 µg/mL were prepared in N,N-dimethyl sulphoxide (DMSO). Wells were cut and 20 µL solution of test sample added. The plates were then incubated at 37°C for 24 h. The antibacterial activity was assayed by measuring the diameter of the inhibition zone formed around the well [Citation31]. The observed results are represented in Table and Figure .
Table 6. Antibacterial activity of Schiff bases (6a–6e) and metal complexes (7a–7j) with Area of inhibition (cm).
The presence of active pharmacophore present in the molecular structures of the synthesized compounds, well positioned halogen, benzofuran moiety and imine (–CH═N–) group, these functional groups might interfere in the mechanism of cell mitosis and hence stop further growth of bacteria. The samples evaluated are showing divergent potency due to the effectual barrier of cell wall membrane of bacteria for access of external matter like test samples under this study.
Complexes showed improved anti-tubercular and antibacterial activities as compared with parent Schiff base ligands. This could be due to the increased lipophilicity of the complexes. The enhanced activity can be elucidated on the basis of co-ordination theory [Citation32]. As stated by Overtone’s concept only material with lipophilic nature favours the transfer across the lipid membrane bacterial cell wall. Hence the degree of lipophilicity of a molecule is a key factor which governs the magnitude of antibacterial activity. Consistent with Ligand Field Theory (LFT), overlapping of metal orbitals with orbitals of ligands minimizes the positive charge on central metal by gaining the electrons from donor groups of the Schiff base ligands [Citation33,Citation34]. This delocalization of electrons from ligand to metal enhances the lipophilic nature of the metal complex. With increased lipophilic character metal complex can easily pass across the bacterial cell wall therefore it can blocks various enzymes of bacteria [Citation35]. Along with this, these complexes might be interacting with DNA gyrase enzyme which is required for DNA multiplication step. This enzyme is blocked by metal complexes which disturb the reproduction of the bacteria cell, eventually kill the bacteria [Citation36,Citation37]. Additionally, these samples retard the respiration process of the cell and thus confine the production of proteins. If the synthesis of proteins is stopped then formation bacterial cell wall is impractical which ultimately consequences to cell death and therefore limits further growth and infection of the bacteria. The antibacterial activities of Cu (II) complexes demonstrated stronger antibacterial activities than Zn (II) complexes. This can be in connection with stronger affinity of Cu (II) for biomolecules. This could enhance the permeability of the Cu (II) complexes through cell membrane resulting in stronger antibacterial activity than Zn (II) complexes [Citation38].
6. Conclusion
In this paper, we synthesized and characterized some new Schiff bases derivatives with benzofuron core and their Cu (II) & Zn (II) complexes in good yields. Followed by evaluation of their antibacterial activity against a panel of bacterial strains. The observed antimicrobial screening results indicate that some of the newly obtained compounds showed significant antibacterial activity, especially against M. tuberculosis. Among them, the activities of compounds 6a, 7c and 7e were strong against M. tuberculosis which is almost two fold higher than that of Pyrazinamide and Ciprofloxacin. In addition to this compounds 6e and 7b have shown to exhibit promising antimicrobial activity against S. aureus, E. coli and B. subtilis.
Acknowledgements
We thank Principal, Government of Maharashtra, Ismail Yusuf Arts, Science and Commerce College for providing research and library facilities. The authors also thank Management and Principal of C. S.’s Patkar-Varde College, Goregaon (W), Mumbai for their constant encouragement and support. The authors thank Dr. Kishore Bhat of Governmental Dental College, Belgaum, for antibacterial study.
Disclosure statement
No potential conflict of interest was reported by the authors.
ORCID
Bhushan Nazirkar http://orcid.org/0000-0002-9762-0614
Mustapha Mandewale http://orcid.org/0000-0001-8346-7594
Ramesh Yamgar http://orcid.org/0000-0002-9013-5376
References
- Moellering R Jr. Discovering new antimicrobial agents. Int J Antimicrob Agents. 2011;37:2–9. doi: 10.1016/j.ijantimicag.2010.08.018
- Schiff H. Mittheilungen aus dem Universitatslaboratorium in Pisa: eine neue Reihe organischer Basen. Justus Liebigs Annalen Der Chemie. 1864;131:118–119. doi: 10.1002/jlac.18641310113
- Dhar D, Taploo C. Schiff bases and their applications. J Sci Ind Res. 1982;41:501–506.
- Przybylski P, Huczynski A, Pyta K, et al. Biological properties of Schiff bases and Azo derivatives of phenols. Curr Org Chem. 2009;13:124–148. doi: 10.2174/138527209787193774
- Bringmann G, Dreyer M, Faber J, et al. Ancistrotanzanine C and related 5,1- and 7,3-coupled naphthylisoquinoline alkaloids from Ancistrocladus tanzaniensis. J Nat Prod. 2004;67:743–748. doi: 10.1021/np0340549
- Dawood K. Benzofuran derivatives: a patent review. Expert Opin Ther Pat. 2013;23:1133–1156. doi: 10.1517/13543776.2013.801455
- Habtemariam S. Antiinflammatory activity of the antirheumatic herbal drug, gravel root (Eupatorium purpureum): further biological activities and constituents. Phytother Res. 2001;15:687–690. doi: 10.1002/ptr.887
- Pauletti P, Araujo A, Young M, et al. Nor-Lignans from the leaves of Styrax ferrugineus (Styracaceae) with antibacterial and antifungal activity. Phytochemistry. 2000;55:597–601. doi: 10.1016/S0031-9422(00)00225-9
- Masubuchi M, Kawasaki K, Ebiike H, et al. Design and synthesis of novel benzofurans as a new class of antifungal agents targeting fungal N-myristoyltransferase. Part 1. Bioorg Med Chem Lett. 2001;11:1833–1837. doi: 10.1016/S0960-894X(01)00319-5
- Wrobel J, Dietrich A, Antane M. Benzothiophenes, benzofurans, and indoles useful in the treatment of insulin resistance and hyperglycemia. US Patent. 2001;6, 251, 936:1–57.
- Kayser O, Chen M, Kharazmi A, et al. Aurones interfere with Leishmania major mitochondrial fumarate reductase. Z Naturforsch C. 2002;57:717–720. doi: 10.1515/znc-2002-7-828
- Hayakawa I, Shioya R, Agatsuma T, et al. 4-Hydroxy-3-methyl-6-phenylbenzofuran-2-carboxylic acid ethyl ester derivatives as potent anti-tumor agents. Bioorg Med Chem Lett. 2004;14:455–458. doi: 10.1016/j.bmcl.2003.10.039
- Proksch P, Rodriguez E. Chromenes and benzofurans of the asteraceae, their chemistry and biological significance. Phytochemistry. 1983;22:2335–2348. doi: 10.1016/0031-9422(83)80118-6
- Oter O, Ertekin K, Kirilmis C, et al. Characterization of a newly synthesized fluorescent benzofuran derivative and usage as a selective fiber optic sensor for Fe(III). Sens Actuators B Chem. 2007;122:450–456. doi: 10.1016/j.snb.2006.06.010
- Karatas F, Koca M, Kara H, et al. Synthesis and oxidant properties of novel (5-bromobenzofuran-2-yl)(3-methyl-3-mesitylcyclobutyl)ketonethiosemicarbazone. Eur J Med Chem. 2006;41:664–669. doi: 10.1016/j.ejmech.2006.01.003
- Habermann J, Ley S, Smits R. Three-step synthesis of an array of substituted benzofurans using polymer-supported reagents. J Chem Soc Perkin Trans. 1999;1:2421–2423. doi: 10.1039/a904384e
- Chohan Z, Farooq M. Mixed ligand biologically active complexes cobalt (II), copper (II), nickel (II) and zinc (II) with triazine-derived NO and NS donor systems. Pak J Pharm Sci. 1995;17:14–18.
- Eswaran S, Adhikari A, Pal N, et al. Design and synthesis of some new quinoline-3-carbohydrazone derivatives as potential antimycobacterial agents. Bioorg Med Chem Lett. 2010;20:1040–1044. doi: 10.1016/j.bmcl.2009.12.045
- Thomas K, Adhikari A, Telkar S, et al. Design, synthesis and docking studies of new quinoline-3-carbohydrazide derivatives as antitubercular agents. Eur J Med Chem. 2011;46:5283–5292. doi: 10.1016/j.ejmech.2011.07.033
- Vavríkova E, Polanc S, Kocevar M, et al. New fluorine-containing hydrazones active against MDR-tuberculosis. Eur J Med Chem. 2011;46:4937–4945. doi: 10.1016/j.ejmech.2011.07.052
- Renuka J, Reddy K, Srihari K, et al. Design, synthesis, biological evaluation of substituted benzofurans as DNA gyraseB inhibitors of mycobacterium tuberculosis. Bioorg Med Chem. 2014;22:4924–4934. doi: 10.1016/j.bmc.2014.06.041
- Mandewale M, Kokate S, Thorat B, et al. Zinc complexes of hydrazone derivatives bearing 3,4-dihydroquinolin-2(1H)-one nucleus as new anti-tubercular agents. Arab J Chem. 2016. doi: 10.1016/j.arabjc.2016.07.016
- Mandewale M, Thorat B, Nivid Y, et al. Synthesis, structural studies and antituberculosis evaluation of new hydrazone derivatives of quinoline and their Zn (II) complexes. J Saudi Chem Soc. 22(2):218–228. doi: 10.1016/j.jscs.2016.04.003
- Mandewale M, Thorat B, Shelke D, et al. Synthesis and biological evaluation of new hydrazone derivatives of quinoline and their Cu (II) and Zn (II) complexes against mycobacterium tuberculosis. Bioinorg Chem Appl. 2015. doi: 10.1155/2015/153015
- Mandewale M, Thorat B, Yamgar R. Synthesis and anti-mycobacterium study of some fluorine containing Schiff bases of quinoline and their metal complexes. Der Pharma Chemica. 2015;7:207–215.
- Yamgar R, Nivid Y, Nalawade S, et al. Novel zinc (II) complexes of heterocyclic ligands as antimicrobial agents: synthesis, characterisation, and antimicrobial studies. Bioinorg Chem Appl. 2014. doi: 10.1155/2014/276598
- Mohamed G, Omar M, Hindy A. Metal complexes of schiff bases: preparation characterization and biological activity. Turk J Chem. 2006;30:361–382.
- Babu V, Ramesh A, Raghuram P, et al. Electron spin resonance studies on complexes of copper (II) with o-phenolic oximes. Polyhedron. 1982;1:607–610. doi: 10.1016/S0277-5387(00)80854-2
- Agharia E. ESR spectral studies of some copper (II) complexes of 1-(1-hydroxy-2-naphthyl)-3-(phenyl or substituted phenyl)-prop-2-en-1-ones. J Applicable Chem. 2014;3:2514–2525.
- Lourenco M, DeSouza M, Pinheiro A, et al. Evaluation of anti-tubercular activity of nicotinic and isoniazid analogues. ARKIVOC. 2007;15:181–191.
- Kiehlbauch J, Hannett G, Salfinger M, et al. Use of the national committee for clinical laboratory standards guidelines for disk diffusion susceptibility testing in New York state laboratories. J Clin Microbiol. 2000;38:3341–3348.
- Tweedy B. Possible mechanism for reduction of elemental sulfur by monilinia fructicola. Phytopathology. 1964;55:910–914.
- Kralova A, Kissova K, Svajlenova O, et al. Biological activity of copper (II) N-salicylideneaminoacidato complexes. Reduction of chlorophyll content in freshwater alga Chlorella vulgaris and inhibition of photosynthetic electron transport in spinach chloroplasts. Chem Pap. 2004;58:357–361.
- Parekh J, Inamdhar P, Nair R, et al. Synthesis and antibacterial activity of some Schiff bases derived from 4-aminobenzoic acid. J Serb Chem Soc. 2005;70:1155–1162. doi: 10.2298/JSC0510155P
- Vaghasia Y, Nair R, Soni M, et al. Synthesis, structural determination and antibacterial activity of compounds derived from vanillin and 4-aminoantipyrine. J Serb Chem Soc. 2004;69:991–998. doi: 10.2298/JSC0412991V
- Galm U, Heller S, Shapiro S, et al. Antimicrobial and DNA gyrase-inhibitory activities of novel clorobiocin derivatives produced by mutasynthesis. Antimicrob Agents Chemother. 2004;48:1307–1312. doi: 10.1128/AAC.48.4.1307-1312.2004
- Alvarez E, Vartanian V, Brodbelt J. Metal complexation reactions of quinolone antibiotics in a quadrupole ion trap. Anal Chem. 1997;69:1147–1155. doi: 10.1021/ac9609081
- Selimović E, Jeremić S, Ličina B, et al. Kinetics, DFT study and antibacterial activity of zinc(II) and copper(II) terpyridine complexes. J Mex Chem Soc. 2018;62(1):1–18. doi: 10.29356/jmcs.v62i1.576