Abstract
In this research, the soil samples were contaminated with 0.05% and 0.1% of cadmium and lead. Seventy pots containing 200 g of soil were polluted with 5% diesel (w/w) (10 g). The physicochemical parameters of the soil were determined. There was a decrease in the pH, and electrical conductivity but the nitrogen, phosphorous, organic carbon and organic matter increased during the 84 days of biodegradation. The bacteria isolated from diesel oil contaminated soil were species of Bacillus and Pseudomonas. The GC-MS analysis revealed a significant decrease in the alkane and an increase in the ester constituents of the biodegraded oil samples after 84 days. Diesel oil co-contaminated soil supplemented with 0.1% of cadmium and lead had a percentage degradation of 89.5% and 80.54% respectively while compared to the un-supplemented samples (28.36%) after 84 days. This indicates that lead and cadmium as co-contaminant in diesel contaminated soil improved the rate of biodegradation of diesel.
1. Introduction
Contamination of soil is prominent among the most critical environmental issues throughout the world, and it has a hugely harmful impact on people, animals, microorganisms, and plants [Citation1]. Correspondingly, heavy metals pollute the earth and have injurious impacts on the environment and its sectors [Citation1]. Activities in the over half a century operations in the oil and gas industry in Nigeria have resulted in unprecedented release of hydrocarbons and associated pollutants including heavy metals into the Niger Delta environment from refine and unrefined petroleum products [Citation2,Citation3]. The severe pollution scenarios have resulted in the degradation of the environment especially soil, resulting in a substantial decline in biodiversity, affect public health and disrupt life support system for local people. Hydrocarbon spills in the form of petroleum products both on land and in water, have been a problem since discovery of oil as an energy source. They can have devastating effects on the biota of an environment. Most hazardous waste sites of public health concerns are contaminated with organic pollutants and heavy metals, and remediation of these sites pose a complex problem because of the mixed nature of contaminants. Industrialization and agricultural practices contribute significantly to the degradation and contamination of environment, which contrarily affects the soil and water bodies [Citation4]. The quest for environmentally sustainable approach towards cleaning contaminated environment has made the ever-evolving field of biodegradation a standard practice for the remediation and restoration of degraded environment.
The most common problem-causing cationic heavy metals are mercury, cadmium, lead, nickel, copper, zinc and chromium, whereas the most common anionic metal is arsenic. Common organic pollutants at these sites include petroleum, polycyclic aromatic hydrocarbons (PAHs), chlorinated solvents, herbicides and pesticides [Citation5]. Heavy metal pollution is a significant problem to the ecosystem since these heavy metals particularly cadmium and lead are potentially toxic even at very trace amounts. Cadmium and lead are more perilous because they tend to bioaccumulate [Citation6]. According to Dindar et al. [Citation7], a high bioremediation rate was observed when waste water sludge containing heavy metals; zinc, copper, nickel, lead and cadmium was used as a biostimulating agent in the bioremediation of engine oil contaminated soil. Therefore, the aim of the study was to investigate the effects of cadmium and lead on biodegradation of diesel in soil.
2. Materials and methods
2.1. Collection of samples
Soil sample was collected from biological garden, Federal University of Technology, Minna, Nigeria. Four litres of diesel oil was purchased from Garima Petroleum Station, Bosso, Minna, Niger State Nigeria. The heavy metals used in this study were cadmium (salts of cadmium tetraoxosulphate (vi) (CdSO4)) and lead (salts of lead trioxonitrate (v) (PbNO3)).
2.2. Experimental setup
The experimental layout consisted of five treatments (diesel oil and heavy metals). Seventy (70) clay pots were soaked in water overnight, 200 g of soil was placed in each pot. The first treatment (A) consisted of 200 g of soil mixed with 5% (10 g) (w/w) diesel, which also served as control. The second treatment (B) consisted of 200 g of soil mixed with 5% (10 g) (w/w) of diesel plus 0.1 mg (0.05%) cadmium. The third treatment (C) consisted of 200 g of soil mixed with 5% (10 g) (w/w) of diesel and 0.2 mg (0.1%) cadmium. The fourth treatment (D) consisted of 200 g of soil mixed with 5% (10 g) (w/w) of diesel and 0.1 mg (0.05%) lead. The fifth treatment (E) consisted of 200 g of soil mixed with 5% (10 g) (w/w) diesel and 0.2 mg (0.1%) lead. Each of the five treatments was reproduced in 7 pots making it 35 pots. The experiment was set up in duplicate. The samples were analysed every 14 days for a period of 84 days [Citation8].
2.3. Isolation and characterization of diesel oil-degrading bacteria
One gram of diesel oil contaminated soil sample from the different treatments were serially diluted. The 0.1 ml was plated on diesel oil agar. The diesel oil agar (1.8 g K2HPO4, 4.0 g NH4Cl, 0.2 g MgSO4.7H2O, 1.2 g KH2PO4, 0.01 g FeSO4.7H2O, 0.1 g NaCl, 1% diesel oil, 1 L of distilled water and 20 g agar) was incubated for 14 days at 37°C. Colonies of different hydrocarbon utilizing bacteria were picked using a sterile inoculating wire loop and subcultured to purify by streaking on nutrient agar plates. The nutrient agar plates were incubated at 37°C for 24 h. All the isolates were Gram stained and subjected to biochemical tests and characterized using the Bergey's Manual of Determinative Bacteriology [Citation9].
2.4. Physicochemical analysis
This involved determination of pH, electrical conductivity [Citation10], organic matter [Citation11], exchangeable acidity (Al + H) and aluminium [Citation12], phosphate, exchangeable aluminium in soil [Citation12] and metals in soil [Citation13,Citation14].
2.5. Statistical analysis of data
Data were subjected to analysis of variance (P ≤ 0.05) using SPSS version 20 and the averages were compared by Duncan Multiple Range Tests (DMRT) P ≤ 0.05. The effect of studied factors was considered significant when P ≤ 0.05.
3. Results and discussion
3.1. Hydrocarbon utilizing bacteria
The characteristics of dominant bacteria able to utilize diesel oil belong to two genera, namely: Bacillus and Pseudomonas. Numerous bacteria are capable of degrading petroleum hydrocarbons, utilizing them as the sole source of carbon and energy. However, the survival of these bacteria in hydrocarbon polluted soil depends on several factors such as pH, temperature, organic content and other physicochemical properties of hydrocarbon contaminated soil. The continuous increase in hydrocarbon utilizing bacteria population in soil sample co-contaminated with 0.1% lead showed that, the presence of lead at that concentration was not sufficient to inhibit the growth of these bacteria or possibly enhanced the ability of these bacterial to tolerate trace amount of heavy metals.
3.2. pH profile of diesel oil and heavy metal polluted soil during biodegradation
The pH profile of polluted soil sample during biodegradation is presented in Figure . A steady decrease in pH of soil samples was observed during biodegradation from day 0 to day 84 of biodegradation. The pH of the soil samples A, B, C, D, and E were observed to decrease from 6.91, 6.09, 5.51, 5.87, and 5.26 to 6.0, 5.06, 4.95, 5.19 and 4.00 respectively after 84 days (Figure ). Statistically, there was a significant difference in the pH of the different treatments during diesel oil biodegradation at (P < 0.05). The decrease in pH is a pointer to the fact that biodegradation of diesel oil in the polluted soil increases with increase in biodegradation time.
Figure 1. pH of soil samples during diesel oil biodegradation. KEY: A = 200 g of Soil + 5% (10 g) (w/w) diesel oil (Control), B = 200 g of Soil + 5% (10 g) (w/w) diesel oil + 0.1 mg (0.05%) cadmium, C = 200 g of Soil + 5% (10 g) (w/w) diesel oil + 0.2 mg (0.1%) cadmium, D = 200 g of Soil + 5% (10 g) (w/w) diesel oil + 0.1 mg (0.05%) lead, E = 200 g of Soil + 5% (10 g) (w/w) diesel oil + 0.2 mg (0.1%) lead.
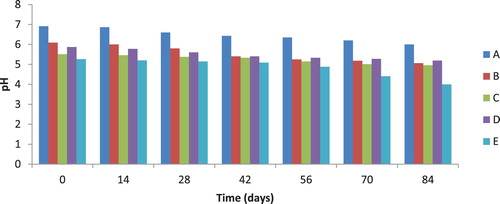
The reduction in pH observed in this study could be as a result of acidic metabolites resulting from diesel oil biodegradation. However, this effect is not strange since the pH values of the diesel oil plus heavy metal contaminated soil were lower than the pH of the control soil sample (only diesel oil contaminated soil). Riskuwa-Shehu et al. [Citation15] recorded a decrease in pH value from 7.15 to 6.70 for crude oil polluted soil incubated with P. aeruginosa UL07 after 10 days.
The same reduction in pH values was reported by previous researchers [Citation16,Citation17]. Acidic pH ranges reported for biodegraded soil could be the most suitable pH range for heavy metals uptake by microorganisms as soil alkaline pH negatively influences the uptake of heavy metals [Citation18]. However, Olama et al. [Citation19] observed maximum diesel oil degradation by B. cereus at pH 7. Metabolic processes are highly susceptible to even a slight change in pH [Citation20], a change in pH has an effect on the biodegradative activity of microbial populations, as well as on the solubilization and absorption/desorption of ions and pollutants.
3.3. Total nitrogen and phosphorous contents of soil during diesel oil biodegradation
The total nitrogen profile of polluted soil samples investigated during biodegradation is presented in Figure . It was observed that total nitrogen content of diesel oil contaminated soil co-contaminated with 0.05% and 0.1% of cadmium and lead increased all through the biodegradation period. The control soil sample contaminated only with diesel oil showed no significant change in total nitrogen content throughout the experiment. Similarly, the phosphorous content of soil samples was checked during biodegradation and the results are presented in Figure . The phosphorous contents of test soil samples were observed to increase above that of the control soil sample from day 0 to day 84 of biodegradation with the maximum value (56.99 ppm) recorded for soil sample contaminated with 5% diesel oil and 0.1% lead. According to Sarkar et al. [Citation17], nitrogen and phosphorous are essential nutrients for hydrocarbons degradation.
3.4. Organic carbon content of soil samples during diesel oil biodegradation
The results for organic carbon of soil samples during biodegradation are shown in Figure . It was observed that the organic carbon content of soil samples co-contaminated with diesel oil and trace amount of heavy metals were much lower than that of the control soil sample (A).
Since diesel oil is a form of organic substance, the reduction in organic carbon content could be attributed to the degradation of the diesel oil by soil microbial flora. Obasi et al. [Citation21] reported an increase in soil organic matter and organic carbon in crude oil polluted soil. Similarly, Ijah et al. [Citation22] reported that the increase in soil organic matter and organic carbon in crude oil contaminated soil brought about a corresponding decrease in nitrogen, phosphorous and an increase in pH values. Furthermore on the effect of soil organic matter and bacterial community shift on bioremediation of diesel-contaminated soil, Liu et al. [Citation23], reported that the total petroleum hydrocarbon degradability reduced with the increase of soil organic matter and organic carbon. Therefore, the decrease in organic carbon recorded for test soil samples compared with the control soil sample is an indication of effective hydrocarbon degradation as a result of increased microbial activities. Hydrocarbon degraders use the hydrocarbon as their sole source of carbon and therefore clean up the oil component in the process. Sandor and Schrader [Citation24] reported a significant decrease in soil organic carbon at the end of their experiment. Similarly, Azarpira et al [Citation25] reported that as compared to initial stage, organic carbon decreased in the final stage.
3.5. Cation exchange capacity (CEC) during diesel oil biodegradation
The results of CEC obtained for soil samples analysed in this study are presented in Table . A uniform CEC trend (10.4, 11.3, 11.98, 12.19, 12. 35 (Cmolkg−1) for samples A, B, C, D, E respectively) was obtained for the soil samples after 42 days. Statistically, there was no significant difference (p < 0.05) in the cation exchange capacity of contaminated soil during the diesel oil biodegradation. The result of this study showed that there was no significant difference (p < 0.05) in the cation exchange capacity of contaminated soil during the diesel oil biodegradation (Table ). These results agree with [Citation26] who observed that the cation exchange capacity (CEC) of bioremediated soil containing spent engine oil increased considerably compared to control. Cation exchange capacity is the total capacity of a soil to hold exchangeable cations. CEC is an inherent soil characteristic that is not easily altered significantly. It influences the soils ability to hold on to essential nutrient and provides a buffer against soil acidification [Citation27]. The results showed that trace amount of heavy metals had no significant effect on CEC of the oil-contaminated soil during biodegradation. This result agrees with [Citation27] who reported that heavy metal adsorption is unsupported by cation exchange mechanism and, hence, they are more influenced by pH variations.
Table 1. Cation exchange capacity (Mol/kg) of soil during diesel oil biodegradation.
3.6. Electrical conductivity of soil during diesel oil biodegradation
Electrical conductivity (EC) of soil samples decreased significantly from day 0 to day 84 of diesel biodegradation as shown in Table . It was observed that EC of heavy metal contaminated soil samples were lower than the control soil (sample A), with minimum reduction observed with soil sample contaminated with 5% diesel and 0.1% lead. Statistically, there was a significant difference (p < 0.05) in the electrical conductivity of soil during diesel oil biodegradation.
Table 2. Electrical conductivity (Us/cm) of soil samples during biodegradation.
Electrical conductivity of soil is a measure of the amount of salts in the soil. It is an important indicator of soil health (NRCS, 2013). EC is one of the several environmental factors considered towards enhancing biodegradation process [Citation28]. High EC value decreases microbial soil activities [Citation29], therefore the reduction in EC values (Table ) during biodegradation in this present study could be due to the increased activities of diesel oil utilizing bacteria in the soil and leaching out the salt content. This result correlates with the findings of Ekperusi and Aigbodion [Citation30] who reported that EC of soil highly increased after contamination of soil with diesel oil and heavy metals, and gradually decreased with the incubation of earthworm. Christopher et al. [Citation31] reported that the EC of crude oil contaminated soil could be as high as 3320 µS/cm which could hinder microbial growth. However, the EC values of contaminated soil samples at day zero in this study was tolerable for the survival of inherent bacterial that further reduced the EC values during degradation of diesel oil.
3.7. Reduction in heavy metal concentration
The reduction in heavy metals as shown in Table could be due to sorption of heavy metals by soil microorganisms basically bacteria and fungi in the formation of oxides, hydroxides, carbonates, exchangeable cations, and or bound to organic matter in the soil [Citation32]. A corresponding significant decrease in the heavy metals concentration with Eudrilus eugeniae after 30, 60 and 90 days was reported by Ekperusi and Aigbodion [Citation30]. Similarly, reduction in heavy metal (cadmium and lead) content of hydrocarbon contaminated soil was observed by Lang et al. [Citation33]. The adsorption of heavy metal by microorganisms is largely dependent on environmental factors such as soil pH.
Table 3. Reduction of cadmium and lead content of soil during biodegradation (mg/kg).
Table presents the concentration of cadmium present in soil during biodegradation of diesel-contaminated soil. It was observed that cadmium and lead showed a corresponding decrease all through the biodegradation period. Statistically, there was a significant difference (p < 0.05) in the cadmium and lead content of soil during diesel oil bioremediation.
3.8. Percentage biodegradation of diesel oil during the biodegradation
Biodegradation of diesel increased steadily from day 0 to day 84 of bioremediation as shown in Table . The degradation rates of diesel oil in soil samples co-contaminated with trace amount of heavy metal were observed to be higher than the control.
Table 4. Percentage biodegradation of diesel oil.
Increasing pH has been shown to increase the toxicity of cadmium to various bacteria (B. subtilis, E. coli, M. luteus, S. bovis), actinomycetes (Micromonospora chalcea, Nocardia corallina, Streptomyces flavovirens), and fungi (Saccharomyces cerevisiae, Schizosaccharomyces octosporus) [Citation27]. Therefore, the decrease in heavy metals and pH of soil samples in this study is of great interest in diesel oil biodegradation. The initial content of heavy metals used in this study can be said to be within the survival limit of microorganisms which supports their adsorption.
Heavy metal tolerance test during biodegradation of crude oil investigated by Tiku et al. [Citation8] showed that some crude oil degraders were tolerant to cadmium at concentrations of 20, 100, 200, 250, and 300 µg/ml. The presence of lead and cadmium in the concentration ranges of 0.21–0.15 mg/kg and 2.10–1.2 mg/kg respectively were also reported by Ibrahim [Citation34] in the biodegradation of used engine oil by novel strains of Ochrobactrum anthropi HM-1 and Citrobacter freundii HM-2.
The highest percentage biodegradation of diesel oil (89.50%) was recorded in soil contaminated with 5% diesel and 0.1% lead (Table ). The increase in percentage biodegradation of diesel oil obtained in this study correlates with the trends in hydrocarbon utilizing bacterial counts observed in the study. The high biodegradation rates could be attributed to an increase in the activity of hydrocarbon utilizing bacteria at this oil pollution level [Citation35]. Biodegradation of crude oil by microorganisms appears to be the natural process by which the bulk of the oil contaminant is used as an organic carbon source, causing the breakdown of petroleum components to lower molecular compounds [Citation36]. The continuous increase in the percentage of diesel oil degradation is in line with the findings of other researchers [Citation35,Citation37] who reported that total petroleum hydrocarbon levels could be significantly reduced by increasing the incubation period during treatment of waste oil-contaminated soil.
In soil sample E (200 g of soil + 5% (10 g) (w/w) diesel oil + 0.2 mg (0.1%) lead), it was observed that only asphaltene fraction (esters) of hydrocarbons were left undegraded after 84 days of biodegradation; the alkanes and aromatic fractions of hydrocarbon had been completely degraded. Asphaltenes are generally considered as being resistant to microbial attack [Citation38]. However, this fraction of hydrocarbon is known to be more polar, which can easily be adsorbed to the lower part of the contaminated soil after biodegradation. It was also observed that peak and retention time correlates with alkane degradation of the soil samples. It is not surprising to record highest retention peak for soil sample E, this is because the efficient degradation of alkanes and aromatic hydrocarbon left behind residue of esters which could be denser than the degraded hydrocarbon. Microbial degradation of hydrocarbon fraction involves interplay of several enzymes. In the case of polyaromatic hydrocarbons, the reaction begins with an oxidation, where oxygenases enzyme groups participate in breaking aromatic ring [Citation14]. Metabolism of hydrocarbon by microorganisms to the formation of fatty acid and ester which they eventually incorporate to their cell has been reported by [Citation39]. The roles of some heavy metals on microbial activities during biodegradation of hydrocarbon have been investigated by previous researchers. Sarkar et al. [Citation17] reported the ability of hydrocarbon-degrading bacteria to withstand cadmium and lead, and their ability to produce oxidase, catalase and biosurfactants required for hydrocarbon degradation.
3.9 Gas chromatography and mass spectroscopy (GC-MS) of Un-degraded and Residual diesel oil Extracted from soil
Gas Chromatography and Mass Spectroscopy (GC-MS) revealed 71 individual hydrocarbons in soil contaminated with diesel oil only (Treatment A) at 0 day as shown in (Figure , Table ). The chromatogram revealed a significant decrease in the alkane and an increase in the ester constituents of the biodegraded oil samples after 84 days (Figure and Tables ).
Figure 5. Chromatogram of diesel oil contaminated soil (a) at day 0 of biodegradation, (b) after 84 days of biodegradation, (c): + 0.1 mg Cd after 84 days of biodegradation, (d) + 0.2 mg Cd after 84 days of biodegradation.
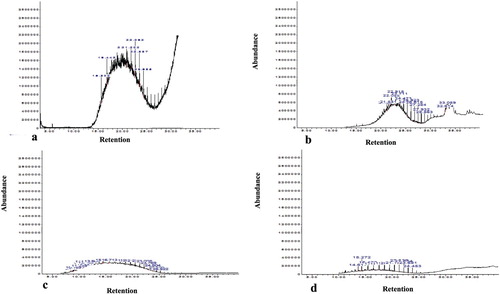
Table 5. Individual hydrocarbons identified in diesel oil contaminated soil at day 0 of biodegradation.
Table 6. Individual hydrocarbons identified in diesel oil contaminated soil after 84 days of biodegradation.
Table 7. Hydrocarbon compounds present in diesel oil contaminated soil + 0.1 mg Cd after 84 days of biodegradation.
Table 8. Biodegradation of hydrocarbons present in diesel oil contaminated soil + 0.2 mg Cd after 84 days.
4. Conclusion
Contamination of soil is a serious global concern. Hence, good and effective remediation technology is of great necessity. In this research, the bacteria isolated from diesel oil contaminated soil were Bacillus subtilis, Bacillus cereus, Bacillus licheniformis and Pseudomonas aeruginosa. These bacteria were able to utilize diesel oil as the sole source of carbon and energy. Co-contamination of diesel oil polluted soil with trace amount (0.05% and 0.1%) of cadmium and lead greatly influenced hydrocarbon utilizing bacterial population during biodegradation; particularly co-contamination with cadmium and lead at 0.1%. There was a decrease in the pH, and electrical conductivity but the nitrogen, phosphorous, organic carbon and organic matter increased during the 84 days of biodegradation. Diesel oil contaminated soil containing 0.1% lead recorded highest percentage degradation of 89.50% at the end of 84 days as compared to other treatments. With this study, it was observed that trace amount of lead and cadmium as co-contaminant in diesel-contaminated soil improved the rate of biodegradation of diesel by Bacillus and Pseudomonas species.
Disclosure statement
No potential conflict of interest was reported by the authors.
ORCID
O. Peter Abioye http://orcid.org/0000-0001-7728-3115
P. F. Aina http://orcid.org/0000-0002-1877-2290
Josiah U.J. Ijah http://orcid.org/0000-0002-4913-6721
Abiodun S. Aransiola http://orcid.org/0000-0002-5537-9172
References
- Abioye OP, Ijah UJJ, Aransiola SA. Phytoremediation of soil contaminants by biodiesel plant Jatropha curcas, Chapter 4 of K. Bauddh et al. (eds.), Phytoremediation potential of bioenergy plants, doi:10.1007/978-981-10-3084-0_4. Springer Nature Singapore Pte Ltd. (2017).
- Obot E, Antonio Q, Braide S, et al. Niger delta natural resource damage assessment and restoration project, phase 1 – scoping report. Federal Ministry of Environment, Abuja, Nigeria Conservation Foundation, Lagos, WWF UK, CEESP- IUCN Commission on Environmental, Economic, and Social Policy. (2006).
- United Nations Environment Programme, Environmental Assessment of Ogoniland, United Nations Environment Programme. ISBN, 978-92-807-3130-9. http,//www.unep.org/nigeria. Accessed 12 Oct 2014 (2011).
- Aransiola SA, Evinemi OT, Falade NO, et al. Assessment of water contamination in Nigeria review. J Bas App Res Int. 2016;19(1):62–76.
- Hoffmann T, Kutter C, Santamaria J. Capacity of Salvinia minima baker to tolerate and accumulate As and Pb. Engr Life Sci. 2005;4(1):61–65.
- Pathiratne K, Senarathna P. Accumulation of heavy metals in a food fish, Mystusgulin inhabiting Bolgoda lake, Sri Lanka. Sri Lanka J Aqu Sci. 2007;12:61–75.
- Dindar E, Fatma O, Topac S, et al. Biodegradation of used engine oil in a wastewater sludge-amended agricultural soil. Turkish J Agri Fore. 2016;40:631–641.
- Tiku DR, Antai SP, Asitok AD, et al. Hydrocarbon biodegradation, heavy metal tolerance, and antibiotic resistance among bacterial isolates from petroleum polluted and pristine soil samples in Calabar metropolis. Int J Cur Tre Pharmaco Med Sci. 2016;1(3):50–64.
- Bergey GH, John GH. Bergey’s manual of determinative bacteriology. 9th ed. Baltimore, MD: Williams and Wikkins; 1994.
- Rhodes JD. Electrical conductivity and total dissolved solids. In Sparks, Method of soil analysis. Published by: Soil Science Society of America, Inc. American Society of Agronomy, Inc. Madison, Wisconsin, USA. (1996) 417–436.
- Nelson DW, Sommers LE. Total carbon, organic carbon, and organic mater.p. 539–580.In A. L. Page (ed), methods of soil analysis. Part 2. 2nd ed. Chemical and microbiological properties. Agronomy monograph no.9. SSSA and ASA, Madison, WI. (1982).
- Alshawabkeh AN. Electrokinetic soil remediation, Challenges and Opportunities. Separation Sci Tech. 2009;44:2171–2187.
- Lindsay WL, Norvell WA. Development of DTPA soil test for zinc, iron, manganese and copper. Soil Sci Soc Am J. 1978;42:421–428.
- Xenia ME, Refugio RV. Microorganisms Metabolism during bioremediation of Oil contaminated soils. J Bioreme Biodegrada. 2016;7(2):340), doi: 10.4172/2155-6199.1000340
- Riskuwa-Shehu ML, Ijah UJJ. Enhanced Removal of crude Oil in soil by mixed Culture of Bacillus megaterium UL05 and Pseudomonas aeruginosa UL07. Int J Environ Bioremed Biodegrad. 2016;4(1):8–12.
- Abiodun O, Yemisi E, Olabode F, et al. Bioremediation of crude Oil polluted soil: Maize Husk Compost as a test Bioremediant. Pharmaceu Chem J. 2016;3(2):254–261.
- Sarkar P, Ajoy R, Siddhartha P, et al. Enrichment and characterization of hydrocarbon-degrading bacteria from petroleum refinery waste as potent bioaugmentation agent for in situ bioremediation. Bioresources Technol. 2017;242:15–27.
- Agnello C, Bagard M, Van Hullebusch D, et al. Comparative bioremediation of heavymetals and petroleumhydrocarbons co-contaminated soil by natural attenuation, phytoremediation. Sci Total Environ. 2015;1:563–564.
- Olama Z, Hanafy H, Darin M. Environmental studies on the microbial degradation of oil hydrocarbons and its application in Lebanese oil polluted coastal and marine ecosystem. Int J Curr Microbio Appl Sci. 2013;2(6):1–18.
- Wang J, Wang M, Wei X. Effects of petroleum in soil growth of maize in loess hilly region of north Shaanxi. Environ Poll Contr. 2012;34(2):55–59.
- Obasi N, Eberechukwu E, Anyanwu I, et al. Effects of organic manures on the physicochemical properties of crude oil polluted soils. Afri J Biochem Res. 2013;7(6):67–75.
- Ijah UJJ, Safiyanu H, Abioye OP. Comparative study of biodegradation of crude oil in soil amended with Chicken droppings and NPK fertilizer. Sci W J. 2008;3(2):63–67.
- Liu PWG, Chang T, Chen C, et al. Effects of soil organic matter and bacterial community shift on bioremediation of diesel-contaminated soil. Int J Biodeter Biodegrad. 2013;85:661–670.
- Sandor M, Schrader S. Interaction of earthworms and enchytraeids in organically amended soil. North-Western J Zool. 2012;8(1):46–56.
- Azarpira H, Behdarvand P, Dhumal K. Effect of different bulking materials and earthworms species on bioremediation potential of municipal sewage sludge. J Environ Earth Sci. 2013;3(12):68–72.
- Milala M, Blessing D, Abdulrahman A. Effects of spent engine Oil on soil physicochemical properties of and microorganisms (bacteria). Asian J Sci Tech. 2015;6:1032–1035.
- Olaniran A, Adhika B, Balakrishna P. Bioavailability of heavy metals in soil, impact on microbial biodegradation of organic compounds and possible improvement strategies. Int J Mol Sci. 2013;14(5):10197–10228.
- Smith E, Thavamani P, Ramadass K, et al. Remediation trials for hydrocarbon-contaminated soils in arid environments, evaluation of bioslurry and biopiling techniques. Int Biodeter Biodegrad. 2015;101:56–65.
- NRCS. Soil electrical conductivity. Soil quality kit—guide for educators. Natural resources conservation service, United States Department of Agriculture; (2013) 7.
- Ekperusi OA, Aigbodion FI. Bioremediation of petroleum hydrocarbons from crude oil-contaminated soil with the earthworm, Hyperiodrilus africanus3. Biotechno. 2015;5(6):957–965.
- Azubuike CC, Chikere CB, Okpokwasili GC. Bioremediation techniques–classification based on site of application, principles, advantages, limitations and prospects. World J Microbial Biotechnol. 2016;32(11):180), https://doi.org/10.1007/s11274-016-2137-x
- Stephen E, Okwute L, Peter J, et al. Microbiological and physicochemical studies of spent lubricating oil contaminated soil amended with wood ash. J Biosci Biotechnol Discovery. 2016;1:36–41.
- Lang F, Tarayre C, Destain J, et al. The effect of nutrients on the degradation of hydrocarbons in Mangrove Ecosystems by microorganisms. Int J Environ Resour. 2016;10(4):583–592.
- Ibrahim HMM. Biodegradation of used engine oil by novel strains of Ochrobactrumanthropi HM-1 and Citrobacter freundii HM-2 isolated from oil-contaminated soil. 3 Biotech. 2016;6(2):226, doi: 10.1007/s13205-016-0540-5
- Abioye OP, Agamuthu P, AbdulAziz A. Biodegradation of used lubricating oil by microbes isolated from pristine soil environment. MJS. 2012;31(1):1–7.
- Zhang Y, Li X, Guowen L, et al. Development of ELISA for detection of mercury based on specific monoclonal antibodies against mercury-chelate. Biol Trace Elem Res. 2011;144:854–864.
- Wang X, Frenq J, Zhao J. Effects of crude oil residuals on soil chemical properties in oil sites, Momonge Wetland, China. Environ Monit Assess. 2010;161(1-4):271–282.
- Eman K, Andrew B. Soil bioremediation approaches for petroleum hydrocarbon polluted Environments. AIMS Micro. 2010;3(1):25–49.
- Rojo F. Degradation of alkanes by bacteria. Environ Microb. 2009;11:2477–2490.