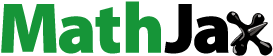
ABSTRACT
Blends of polyamide 6/polystyrene (PA6/PS) (50/50 wt./wt. %) were loaded with different concentrations of boron carbide (B4C), (0, 5, 10, 15, 20 and 30 wt./wt. %) separately for EMI shielding. The effects of different weight fractions of fillers on thermal, electrical, as well as, mechanical behavior of the prepared composites were investigated. Thermal stability and hardness (shore D) of composites are studied. Addition of B4C enhances thermal stability of the prepared composites which attributed to the increase in physical and chemical cross-linking points, also to the interactions between the filler and polymer blend. Confirmation of thermal stability was done by determining the activation energy (Ea) for the thermal decomposition of all composites. Characterization of the prepared composites was done using SEM. With B4C-loaded PA6/PS blend composites, samples of 2 mm thick, SE values of 5–36 dB were achieved, satisfying the requirement for common commercial applications.
1. Introduction
Compounds with high molecular weight constitute one of the major fields of modern technology, which either natural or synthetic high polymers participate in a wide range of industrial applications [Citation1], and also can be acquired in different forms like powders, thin films etc. [Citation2]. So as to set a material suitable for applications in various technological fields, one needs to defeat certain constraints such as poor mechanical properties and processability and instability in ambient conditions. Several approaches have been made by many researchers to improve these properties [Citation3].
On the other hand, given the fast improvement in commercial, military and logical electronic gadgets and correspondence instruments, there has been an increased enthusiasm in developing materials that could shield against electromagnetic radiation (non-ionizing radiation) to prevent interference. An electromagnetic interference (EMI) shield is a boundary made of conductive material that attenuates radiated electromagnetic energy.
The hardest ceramic material discovered in nature after precious stone and cubic boron nitride [Citation4] is boron carbide (B4C) which is dark, strong with metallic gloss and rhombohedral structure with high synthetic solidness in the hostile environment and high protection from wear due to its higher mechanical quality utilized in applications with high temperature in light of high obstinacy property accomplished because of the high liquefying purpose of 2,450°C. It has low thermal conductivity and high compressive quality and hardness
Among the different accessible fillers, B4C has been seen as a standout material amongst the most promising ceramic materials in view of its extraordinary physical and mechanical properties, for example, high temperature and chemical stability, high hardness, great neutron retention ability, high modulus of elasticity, low density, high impact and wear resistance. The radiation protecting properties of different sorts of polymers fortified with B4C, a property of extraordinary significance in the atomic fuel transport and over voltage insurance conditions, have been as of now considered [Citation5,Citation6].
Polystyrene (PS), which is a transparent, rigid and brittle thermoplastic polymer that softens slightly over 100°C, turns into a viscous liquid at around 185°C. It is resistant to acids, alkalis, oils and alcohols and can be used in many applications that include electrical and thermal insulation, food packaging, audiocassette housings and toys. PS finds a diversity of applications in a wide range of markets due to its properties, low cost and eases of fabrication [Citation7,Citation8].
Polyamides incorporate a scope of materials, contingent upon the monomers utilized. Nylon-6,6 and nylon-6 keep on being the most well-known popular types, still accounting for more than 90% of nylon use.
Nylon, particularly nylon 6, is known to be extremely notch-sensitive and brittle at low temperature. Adding some thermoplastic elastomers or special additives is a normally utilized practice to obtain new materials with good impact properties [Citation9].
Several researches have been developed and investigated the properties of Al-based metal matrix composites and non-developed polymer B4C blends or composites for the control of electromagnetic radiation. Auradi et al. [Citation10] reinforced 6061 Al matrix composites with B4C prepared by the stirring method. B4C distributed uniformly without clustering, which is confirmed by scanning electron microscopic (SEM) studies. Mechanical properties of the Al matrix composites have been improved by the addition of B4C. A microstructural study and tensile behaviour of B4C-filled A356 alloy composites have been studied by Pankaj et al. [Citation11]. A matrix composite containing B4C also fabricated by the stirring method, and SEM examined the microstructure of the composite which revealed the uniform distribution of filler in the matrix. B4C increases ultimate tensile strength and yields strength but the elongation of the composite decreases. Mechanical and tribological performances and wear behaviour of the poly proplyene (PP) composites have been studied by Dikea et al. [Citation12] with the addition of B4C. Hardness, yield strength, wear and friction under dry sliding were increased by increasing the B4C content. B4C was added with a constant amount of graphite particles by different volume fractions to study mechanical and wear behaviour of Al–Gr–B4C composites prepared by the stirring method by Senthilkumar et al. [Citation13]. Microstructural characterization studies confirmed a uniform distribution of B4C particles at the microscopic scale with less porosity. Micro-Vickers hardness test, compression strength and tribological behaviour were studied using the wear test, leading to an increase in wear resistance with an increase in B4C reinforcement.
The development in the use of electronic gadgets over a wide range of military, mechanical, business and customer areas has made another type of contamination known as noise or radio frequency interference or electromagnetic radiation or electromagnetic interference (EMI) that can cause obstruction or breaking down of equipment. Along these lines, there is a more prominent requirement for the effective shielding of segments from its adverse impacts.
The present investigation is concerned with detailed studies on thermal, electrical and mechanical properties of polyamide 6/polystyrene (PA6/PS) composites filled with B4C to achieve polymers which can be used in control of electromagnetic radiations.
2. Experimental
2.1. Materials and preparation of samples
2.1.1. Preparation of samples
PA6 which supplied from El-Nasr Company for chemicals and drugs (Egypt) with purity 99.99% and PS which supplied from Sigma-Aldrich Company with an average Mw of ∼192,000 were used in this study. The formulation of different mixtures is given in Table , and the blended composites were prepared. The samples (PA6/PS) with different volume fraction of fillers (0, 5, 10, 15, 20 and 30 wt./wt.%) of B4C, which has been supplied by Sigma-Aldrich Company with a maximum particle size of 45 µm and purity 99%, were prepared as follows. The PA6/PS (50:50) was loaded into the Brabender and mixed for 5 min. B4C was added into the composites and mixed for 4 min. The final mixing was carried out for 2 min in the two roll mill. Finally, the composites were moulded between smooth Teflon sheets at a temperature of 240°C and a pressure of 25 MPa in an electrically heated press for a specific time (10 min). The compression moulded samples were allowed to cool at room temperature and kept for maturation (24 h) before measurement.
Table 1. Composition of PA6/PS samples with different concentrations of B4C.
2.2. Measurements
2.2.1. Thermogravimetric analysis
The TGA thermograms were performed on a Shimadzu-50 instrument (Kyoto, Japan) at a heating rate of l0°C/min from room temperature to 600°C and under a constant rate of nitrogen (20 ml/min) to prevent thermal oxidation processes of polymer samples and to investigate the thermal stability.
2.2.2. Dielectric properties of the polymer blend
The dielectric properties were measured using a bridge (HIOKI 3538-50 LCR Hi Tester) in the frequency range of 102–106 Hz. The bridge connected via a standard interface (RS-232C interface, Hioki, Japan) to a PC for instrument control and data processing. The samples were in the form of disks of 0.2–0.3 cm thick and 1.0 cm in diameter and then sandwiched between two brass electrodes, and the dielectric constant and dielectric loss of the samples were measured at different temperatures.
2.2.3. Microstructural characterization and mechanical testing
The prepared composites were subjected to microstructural characterization using a Scanning Electron Microscopy Model Quanta FEG-250 instrument (from FEI Company, Netherlands) with an accelerating voltage of 30 kV, magnification 14× up to 1,000,000, and resolution for Gun is 1n to identify the morphology and distribution of B4C particles in the PA6/PS matrix. Samples were fractured in liquid nitrogen, and the fractured surfaces were gold-plated and then mounted over an aluminium stub with a double-sided electric adhesive tape. The vacuum was on the order of 10−4–10−6 mmHg during the scanning of the composite samples. Samples were characterized by studying the physical properties like hardness (shore D), the test was held at room temperature for each composition, and the average value was represented for each sample.
2.2.4. Electromagnetic interference measurements
The EMI measurements from 300 MHz to 4.5 GHz were carried out using HP synthesized CW frequency generators Model No. 83712B (transmitter) and HP Spectrum Analyzer model No. 8592L (receiver) with two opposite directed conical horned antenna. The EMI shielding effectiveness was measured as per the method specified by the American Military Standard MIL-STD-285. The PA6–PS/B4C samples with a thickness of about 2.0 mm were used during measurement. EMI SE was determined by the subtraction of the power level recorded with the testing samples in place from the power level recorded during the open reference measurement.
3. Results and discussion
3.1. Thermal stability and analysis
Thermal analysis techniques are used in virtually every area of modern science and technology. The basic information that these techniques provide, such as crystallinity, specific heat and expansion, are very important for the research and development of new products. Thermal analysis techniques also find increasing use in the area of quality control and assurance [Citation14]. One of the most widely used techniques is thermogravimetric analysis (TGA).
3.1.1. Thermogravimetric analysis
Thermal degradation of polymers could be described by TGA which provides a method for thermal stability testing [Citation15–21] and determines the activation energy of thermal decomposition reaction [Citation22,Citation23]. Information about the kinetics of decomposition can be obtained with proper experimental procedures and the choice of a polymer depending on thermogravimetric analysis data. The kinetic parameters determined from data of non-isothermal thermogravimetry are one of the most difficult kinetic problems. Methods of kinetic data analysis have been classified to integral, differential, and specified methods [Citation24–26] which have usually been applied to study the decomposition of polymeric materials from thermogravimetric data.
Thermal stability is always evaluated a sample by TGA which is used to measure the thermal properties of the unfilled PA6/PS (50:50 wt./wt.%) blend and its composites. Figure shows the dynamic TGA thermograms of unfilled blend and PA6/PS blends filled with different concentrations (0–30 wt./wt.%) of B4C. We can see only one sharp decomposition profile for all samples. The unfilled blend decomposition begins at 360°C and about 98% decomposition occurred at 550°C. Onset degradation temperatures, weight loss in the major degradation step and char residues are presented in Table . It is clear that at higher temperatures, the filled blend composites begin to decompose than the unfilled one which indicates that it is stable at higher temperatures. To compare the thermal stabilities of the blend composites, T5% was chosen which is the temperature of a sample which loses 5% of its weight (see Table ). T5% thus stands for the onset of thermal decomposition. At 520°C, PA6/PS blend as shown in Figure got decomposed completely while, in the composites, a char residue of about 2.3–29.3% was left depending on filler loading. Both char residue and onset temperature increase systematically with increasing filler loading. The addition of B4C enhances the thermal stability of the blend. The physical and chemical cross-linking points which are increased due to the interactions between the filler and the polymer blend are the reasons for the improvement in thermal stability of the filled polymer.
Figure 1. TGA thermograms for samples PA6–PS loaded with different concentrations of B4C filler particles and unfilled one.
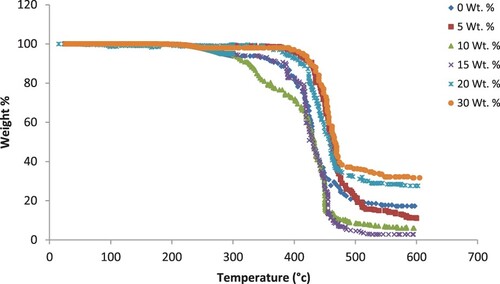
Table 2. Kinetic parameters for the thermal degradation of PA6–PS/B4C composites.
3.1.2. Activation energy (Ea)
Activation energy (Ea) is determined thermal stability by evaluating thermal decomposition of unfilled PA6/PS and filled composites according to the method represented by Horowitz and Metzger [Citation27,Citation28]. Plot a relation between ln {ln[(X0 – Xr)/(Xt − Xr)]} and θ (see Figure ) gives a straight line in which the activation energy can be calculated from a slope of {(Ea × 103)/} as R = 8.314 J K−1 mo1−1. In the slope, Xr and X0 are the final and initial weights of the samples, respectively. Xs is the remaining weight of the sample at a given temperature Ts [Citation29]:
(3.1)
(3.1) where T is the sample temperature and Ts the reference temperature which is the temperature where this relation applied [Citation30]
(3.2)
(3.2) The activation energies calculated for PA6–PS/B4C composites and the results are presented in Figure . The activation energy of thermal oxidation has been increased by increasing the B4C filler content, which enhances the thermal stability of the matrix.
Figure 2. ln {ln[(X0 − Xf)/(Xt − Xf)]} against θ for calculating the slope of samples PA6/PS loaded with different concentrations of B4C filler particles.
![Figure 2. ln {ln[(X0 − Xf)/(Xt − Xf)]} against θ for calculating the slope of samples PA6/PS loaded with different concentrations of B4C filler particles.](/cms/asset/20538f56-2f95-4aae-a721-627e1d72a28c/tusc_a_1710323_f0002_oc.jpg)
As a conclusion, the incorporation of B4C in the blend matrix improves its thermal stability.
3.2. Dielectric properties of the polymer blend
For potential applications in solid-state devices, conductivity relaxation behaviour in conducting polymer composite material studies has become an interesting area of active research [Citation31–33]. The permittivity or dielectric constant (ε′), which is a measure of the energy stored in a sample during a cyclic electric excitation and loss factor which are used to characterize molecular relaxations, was measured and usually the energy stored in the form of a non-uniform dipole distribution or ionic charge layers.
The dielectric constant (ε′) versus temperature of PA6/PS blends loaded with different concentrations of B4C (0, 5, 10, 15, 20 and 30 wt./wt.%) are shown in Figure at constant frequencies 1 and 100 kHz and also at temperatures varying from 20°C to 150°C.
Figure 4. Dielectric constant versus temperature at (a) 1 kHz and (b) 100 kHz of PA6–PS samples loaded with B4C (0–30 wt./wt.%).
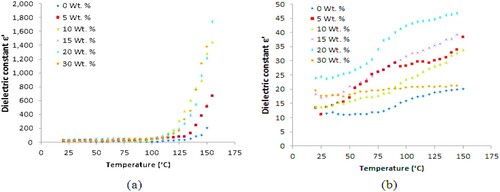
The dielectric constant ε′ increases significantly with increasing B4C content. In the dielectric constants versus temperature plots of all composites, it can be seen that there is a marginal increase in ε′ for all composites up to ≈150°C. At temperature above 150°C and at 1 kHz, ε′ dramatically increases with temperature and then decreases to its original value as the frequency increases to 100 kHz which demonstrates the presence of dielectric relaxation.
However, the loss peaks related to this relaxation are not evident in Figure , showing the dielectric loss versus temperature of the blends at the same temperature.
3.3. Mechanical properties
The mechanical property such as surface hardness (shore D) of PA6–PS/B4C composites is shown in Figure . As seen from the figure, the surface hardness goes through a maximum value at a B4C content of 10 wt./wt.% and then decreases.
In the filled material, as the filler concentration increased the hardness increases. And also in highly filled materials, the hardness of the composite approaches to the hardness of the filler.
Meanwhile, many types of filler such as B4C were found to induce a softening effect in the polymer matrix [Citation34]. B4C is a soft material but still may either decrease or increase the hardness of a polymer which depends on particle size and its interaction. The decrease in the surface hardness value above 10 wt./wt.% with the addition of B4C is again attributable to the softening effect of B4C.
3.4. Electromagnetic interference shielding effectiveness
The effect of electromagnetic shielding is to reduce the electromagnetic field effect in a specific region produced by some radiation sources and to adequately control the damage caused by electromagnetic radiation starting from one area to another. The guideline of action is the use of low-resistance conductor material, because the conductor material has a reflection and guiding effect on electromagnetic energy flow, and within the conductor material, it creates the current and magnetic polarization which is opposite with the source of electromagnetic field, thereby reducing the effect of radiation source in electromagnetic field; normally, it is represented by shielding effectiveness (SE) which refers to the ratio of the incident or reflection electromagnetic waves to the reflection or transmission of electromagnetic wave under shielding at the same location, that is, shielding material to the attenuation value of electromagnetic signal, the unit is dB.
Metallic powders, flakes, whiskers and other conducting fillers incorporation such as carbon black (CB) and graphite in conducting polymer composites have attracted wide attention due to their effectiveness in such applications as EMI shielding [Citation34–41], electrostatic charge dissipation [Citation39] and sensors for vapours and chemicals [Citation40]. Spurious voltage and current induced in the electronic circuits by the external sources can be defined as electromagnetic interference. Due to high demand on the reliability of electronics, radio frequency radiation source growth and avoiding damage to communication systems, EMI shielding is very important. The best materials are metals for electromagnetic shielding but expensive and heavy. Therefore, polymers can be used for housing the electronic devices due to their light weight, flexibility and less expensive. But due to their inherent EMI SE is practically zero, the technical approach is to incorporate electrically conductive filler in the polymer matrices.
The ratio of the transmitted power (Ptrans) to the incident power (Pinc) of the microwave passing through the sample can be defined as SE and is generally expressed in decibel (dB) as mentioned elsewhere:
(3.3)
(3.3) PA6/PS (50:50 wt./wt.%) blend of different loadings of B4C was examined at different applied frequencies for their EMI shielding. The SE-measured values starting from 500 MHz to 5 GHz are given in Figure . With B4C-loaded PA6/PS blend composites, with 2 mm thickness samples, values of SE about 5–36 dB can be obtained, which satisfy the requirement for common commercial applications. For high EMI SE, therefore, composites having (30 wt./wt.%) B4C loading are indicated to be quite effective and useful. SE of the composites increased with the different loadings of B4C. The dominant shielding characteristics of absorption from conductive polymer composites were indicated by the SE performance against the frequency of electromagnetic waves.
3.5. Scanning electron microscope
Figure shows the images of the PA6–PS loaded with different concentrations of B4C (5, 15 and 30 wt./wt.%) matrix. On the micrograph, dark areas are the regions of almost pure polymer material free of the filler, whereas light areas are the regions with B4C. It can be seen that the B4C particles are almost uniformly dispersed in the PA6–PS composite matrix. However, as the content of the B4C in the PA6–PS matrix increased from 5 to 30 wt.%, agglomerations of the B4C particles could not be avoided as shown in Figure (c). This is due to the decrease in the interparticle distances between the B4C particles with increasing B4C content in the matrix.
4. Conclusions
From the obtained results, the following conclusions can be derived. The addition of B4C enhances the thermal stability of the blend, Both char residue and onset temperature increase systematically with increasing filler loading, and a decomposition profile for all samples occurs in one sharp step. The addition of B4C as a filler increases the activation energy of thermal oxidation which enhances the thermal stability of the matrix. The surface hardness goes through a maximum value at a B4C content of 10 wt./wt.% and then decreases; this decrease in the surface hardness value above 10 wt./wt.% with the addition of B4C is attributable to the softening effect of B4C. The activation energy highly affected by filler concentration, and it is situated within the range of 0.58–0.68 eV for samples loaded with B4C. The dielectric constant and dielectric loss, which are used to characterize molecular relaxations, were investigated in the frequency range from 100 Hz to 5 MHz. ε′ and versus temperatures (25–150°C) fixed at frequencies of 1 and 100 kHz were measured. It is found that the dielectric constant increases significantly with increasing B4C content. Relaxation peaks are clearly formed for all samples, increase in B4C contents and shifts peaks to lower frequencies. EMI shielding characteristics of PA6/PS filled with B4C have been studied and found that the SE of the composites is frequency dependent. It increases with the filler loading. The results indicate that the composites having higher filler loading (30 wt./wt.%) can be used for EMI shielding and for some microwave applications.
Disclosure statement
No potential conflict of interest was reported by the authors.
References
- Elwy A, Badawy MM, Nasr GM. Electrical properties and penetration rate of solvent into irradiated LDPE/SBR conductive blend. Polym Degrad Stab. 1996;53:289–294. doi: 10.1016/0141-3910(96)00090-0
- Nasr GM, Osman HM, Omar MM, et al. Thermal and dielectric properties of PVC/PMMA loaded conductive PPY composites. Life Sci J. 2014;11(4):127–134.
- Nasr GM, Amin M, Shaker HM. Mechanical properties of natural rubber nano composites. Int J Acad Res. 2014;6.
- Thevenot F. Boron carbide—a comprehensive review. J Eur Ceram Soc. 1990;6(4):205–225. doi: 10.1016/0955-2219(90)90048-K
- Mondal S, Banthia AK. Low-temperature synthetic route for boron carbide. J Eur Ceram Soc. 2005;25(2-3):287–291. doi: 10.1016/j.jeurceramsoc.2004.08.011
- Kaloshkin SD, Tcherdyntsev VV, Gorshenkov MV, et al. Radiation-protective polymer-matrix nanostructured composites. J Alloys Compd. 2012;536:S522–S526. doi: 10.1016/j.jallcom.2012.01.061
- Boundy RH, Boyer RF. Styrene: its polymers, copolymers, and derivatives. No. 115. New York: Reinhold; 1952.
- Craver C, Carraher C. Applied polymer science: 21st century. UK: Elsevier; 2000.
- Peng J, Zhang X, Qiao J, et al. Radiation preparation of ultrafine carboxylated styrene-butadiene rubber powders and application for nylon 6 as an impact modifier. J Appl Polym Sci. 2002;86:3040–3046. doi: 10.1002/app.11323
- Auradi V, Rajesh GL, Kori SA. Processing of B4C particulate reinforced 6061aluminum matrix composites by melt stirring involving two-step addition. Proc Mater Sci. 2014;6:1068–1076. doi: 10.1016/j.mspro.2014.07.177
- Jadhav PR, Sridhar BR, Nagaral M, et al. Mechanical behavior of B4C particulates reinforced A356 alloy composites. Am J Mater Sci. 2016;6(4A):51–55. DOI:10.5923/c.materials.201601.10.
- Dikea AS, Mindivanb F, Mindivanc H. Proceedings of the 3rd International Congress APMAS; 2014.
- Senthilkumar N, Tamizharasan T, Anbarasan M. Mechanical characterization and tribological behaviour of Al-Gr-B4C metal matrix composite prepared by stir casting technique. J Adv Eng Res. 2014;1.1:48–59.
- Brown ME, Gallagher PK. Handbook of thermal analysis and calorimetry: recent advances, techniques and applications. Vol. 5. UK: Elsevier; 2011.
- Lin Y-L, Yang M-H, Wang J-H. On the thermal degradation of poly(styrene sulfone)s. I: an apparatus for investigation of early stages of thermal degradation. Polym Test. 1996;15:525–536. doi: 10.1016/0142-9418(96)00009-8
- Nien K-E, Yang M-H, Chu T-J. The effects of reaction conditions on the synthetics and properties of polypyromellitimide. J Polym Eng. 1997;17(1):23–38. doi: 10.1515/POLYENG.1997.17.1.23
- Yang M-H. The two-stages thermal degradation of polyacrylamide. Polym Test. 1998;17:191–198. doi: 10.1016/S0142-9418(97)00036-6
- Lee R-J, Yang M-H, Wang J-H. On the thermal degradation of poly(styrene sulfone)s. Polym Test. 18 601–609 (1999). doi: 10.1016/S0142-9418(98)00058-0
- Yang M-H. The thermal degradation of polyacrylamide with adsorbed metal ions as stabilizers. Polym Test. 2000;19:85–91. doi: 10.1016/S0142-9418(98)00074-9
- Yang M-H. On the thermal degradation of poly(styrene sulfone)s VIII. Effect of structure on thermal characteristics. Polym Degrad Stab. 2002;76:69–77. doi: 10.1016/S0141-3910(01)00267-1
- Tsay D-K, Yang M-H, Wang J-H. On the thermal degradation of polysulfones IX. The early stages of thermal degradation of poly(1-butene sulfone) and poly(2-methyl-1-pentene sulfone). Polym Degrad Stab. 2002;76:251–257. doi: 10.1016/S0141-3910(02)00021-6
- Mishra R, Rao KJ. Thermal and morphological studies of binary and ternary composites of poly(vinylalcohol) with alumina and zirconia. Ceram Int. 2000;26:371–378. doi: 10.1016/S0272-8842(99)00066-8
- Rao PS, Subrahmanya S, Sathyanarayana DN. Synthesis by inverse emulsion pathway and characterization of conductive polyaniline-poly(ethylene-co-vinyl acetate) blends. Synth Met. 2003;139:397–404. doi: 10.1016/S0379-6779(03)00192-9
- Chu J-C, Lin Y-C, Yang M-H. Flexibility improvement of epoxy resin by using γ-butyrolactone. Polym Technol Eng. 1997;36:473–488. doi: 10.1080/03602559708000636
- Contat-Rodrigo L. Thermal characterization of the oxo-degradation of polypropylene containing a pro-oxidant/pro-degradant additive. Polym Degrad Stab. 2013;98:2117–2124. doi: 10.1016/j.polymdegradstab.2013.09.011
- Nishizaki H, Yosida K. Effect of molecular weight on various TGA methods in polystyrene degradation. J Appl Polyrn Sci. 1981;26(10):3503–3504. doi: 10.1002/app.1981.070261029
- Horowitz HH, Metzger GA. A new analysis of thermogravimetric traces. Anal Chem. 1963;35:1464–1468. doi: 10.1021/ac60203a013
- Sinha D, Dwivedi KK. Radiation-induced modification on thermal properties of different nuclear track detectors. Radiat Meas. 2003;36:713–718. doi: 10.1016/S1350-4487(03)00232-4
- Nouh SA, Naby AA, Sellin PJ. Modification induced by proton irradiation in Makrofol-DE polycarbonate. Radiat Meas. 2007;42(10):1655–1660. doi: 10.1016/j.radmeas.2007.04.005
- Nouh SA, El-Mahdy NA, Morsy AA. Modification of thermal, optical and structural properties of Bayfol nuclear track detector by alpha particles irradiation. Radiat Meas. 2005;39:471–477. doi: 10.1016/j.radmeas.2004.10.007
- Heeger AJ, Kivelson S, Schrietter JR, et al. Solitons in conducting polymers. Rev Mod Phys. 1988;60:781. doi: 10.1103/RevModPhys.60.781
- Stafsform S, Bredas JL, Epstein AJ, et al. Polaron lattice in highly conducting polyaniline: theoretical and optical studies. Phys Rev Lett. 1987;59(13):1464. doi: 10.1103/PhysRevLett.59.1464
- Bhattacharyya S, Soka SK, Chakarvorty D. Conductivity relaxation behavior of interpenetrating polymer network composites of polypyrrole and poly(styrene-co-butyl acrylate). J Phys Sci, B: Polym Phys. 2000;38:1193.
- White L. Eur Rubber J. 1995;177(2):18.
- Sohi NJS, Rahaman M, Khastgir D. Dielectric property and electromagnetic interference shielding effectiveness of ethylene vinyl acetate-based conductive composites: effect of different type of carbon fillers. Polym Compos. 2011;32:1148–1154. doi: 10.1002/pc.21133
- Kim SH, Jang SH, Byun SW, et al. Electrical properties and EMI shielding characteristics of polypyrrole–nylon 6 composite fabrics. J Appl Polym Sci. 2003;87(12):l969–1974.
- Norman RH. Electrically conducting rubber composite. UK: Elsevier; 1970.
- Lonergan MC, et al. Array-based vapor sensing using chemically sensitive, carbon black−polymer resistors. Chem Mater. 1996;8:2298–2312. doi: 10.1021/cm960036j
- El-Tantawy F, Dishovsky N. Novel V-shaped negative temperature coefficient of conductivity thermistors and electromagnetic interference shielding effectiveness from butyl rubber-loaded boron carbide ceramic composites. J Appl Polym Sci. 2004;91:2756–2770. doi: 10.1002/app.13458
- Vittoria C, Koon NC, Lubitz P, et al. Microwave properties of (Fe0. 82B0. 18) 0.09 La0. 05R0. 05 amorphous metal alloys. J Appl Phys. 1984;55(6):1741–1742. doi: 10.1063/1.333461
- Chung DDL. Electromagnetic interference shielding effectiveness of carbon materials. Carbon 2001;39:279–285. doi: 10.1016/S0008-6223(00)00184-6